Abstract
Simkania negevensis is a Chlamydia-related bacterium discovered in 1993 and represents the founding member of the Simkaniaceae family within the Chlamydiales order. As other Chlamydiales, it is an obligate intracellular bacterium characterized by a biphasic developmental cycle. Its similarities with the pathogenic Chlamydia trachomatis and Chlamydia pneumoniae make it an interesting bacterium. So far, little is known about its biology, but S. negevensis harbors various microbiological characteristics of interest, including a strong association of the Simkania-containing vacuole with the ER and the presence of an intron in the 23S rRNA encoding gene. Evidence of human exposition has been reported worldwide. However, there is a lack of robust clinical studies evaluating its implication in human diseases; current data suggest an association with pneumonia and bronchiolitis making S. negevensis a potential emerging pathogen. Owing to its fastidious growth requirements, the clinical relevance of S. negevensis is probably underestimated. In this review, we summarize the current knowledge on S. negevensis and explore future research challenges.
Introduction
Several members of the Chlamydiales order are of high clinical importance. For example, Chlamydia trachomatis is currently the second most common sexually transmitted disease after human papillomavirus (HPV) (Forhan et al., Citation2009). This infection can cause pelvic inflammatory disease (PID) (Bartlett et al., Citation2013) and lead to infertility as well as obstetrical complications such as miscarriage and preterm birth (Baud et al., Citation2011a, Citation2008; Gimenes et al., Citation2014; Pellati et al., Citation2008). Similarly, Chlamydia pneumoniae commonly causes bronchitis and pneumonia in children and young adults (Asner et al., Citation2014; Blasi et al., Citation2009; Principi & Esposito, Citation2001) and Chlamydia psittaci is an important zoonotic agent associated with atypical pneumonia as well as systemic infections (Knittler & Sachse, Citation2015; Schachter, Citation1986). In the past decades, various Chlamydia-related bacteria have been discovered, notably Simkania negevensis (S. negevensis). These bacteria have been classified in the Chlamydiales order, as they exhibit a biphasic developmental cycle with infectious Elementary Bodies (EBs) and replicative Reticulate Bodies (RBs) similar to what is known for Chlamydia spp. Growing evidence suggests a pathogenic role for many of these Chlamydia-related bacteria mainly in respiratory diseases and in obstetrical complications (Baud et al., Citation2014, Citation2008; Corsaro & Greub, Citation2006; Greub, Citation2009; Greub & Raoult, Citation2002a; Greub et al., Citation2003a; Lamoth & Greub, Citation2010). Due to the lack of commercial diagnosis methods and clinical guidelines, these strict intracellular bacteria are, however, not routinely screened in the clinic. It is therefore essential to better understand the biology and to clearly define their clinical importance as they may represent important human pathogens.
In this review, we summarize the current knowledge on S. negevensis and explore future research challenges.
History
Simkania negevensis was discovered in 1993 as a cell culture contaminant. In their first report, Simona Kahane et al. describe the micro-organism “Z” as an intracellular bacterium, which exhibited a two-phase replicative cycle similar to Chlamydia spp. However, it was not recognized neither by Chlamydia-specific PCR primers, nor by Chlamydia-specific antibodies (Kahane et al., Citation1993). The analysis of the 16S rRNA encoding gene showed an 83% homology with Chlamydia spp. and 73% with Rickettsia (Kahane et al., Citation1995); the authors concluded to the discovery of a new Chlamydia-like bacterium. To better characterize the relationship of the microorganism with members of the Chlamydiaceae family, the 16S/23S rRNA intergenic spacer and Domain I of the rRNA encoding gene of these species were analyzed (Everett & Andersen, Citation1997). This work led to the description of the Simkaniaceae family within the Chlamydiales order with the microorganism Simkania «Z» as a founding member. It was renamed, afterwards, Simkania negevensis in honor of Simona Kahane from the Ben-Gurion University of Negev, Israel (Everett et al., Citation1999a).
S. negevensis was the first Chlamydia-like organism described. Its discovery gave an insight of the wide ecological diversity of the Chlamydiales order, and opened new research fields. Indeed, in the past decade, various Chlamydia-like organisms have been discovered (Corsaro & Greub, Citation2006; Corsaro & Venditti, Citation2009; Horn, Citation2008; Lienard et al., Citation2011b), notably using amoebal co-culture techniques (Jacquier et al., Citation2013). In 1999, based on the percentage of the 16S and 23S rRNA encoding gene sequence similarities (>80 and <90%), Everett et al., proposed, in addition to the Simkaniaceae family, two novel families within the Chlamydiales order: the Waddliaceae and the Parachlamydiaceae (Everett et al., Citation1999a). These “Everett” cutoffs have been validated by the International Subcommittee of Taxonomy (Greub, Citation2010) and have been recently extended by Pillonel et al. to use six additional taxonomically informative genes (Pillonel et al., Citation2015). Currently, the order is divided into nine family-level lineages, namely the Chlamydiaceae, Clavichlamydiaceae, Criblamydiaceae, Parachlamydiaceae, Parilichlamydiaceae, Piscichlamydiaceae, Rhabdochlamydiaceae, Simkaniaceae and Waddliaceae (Bavoil et al., Citation2013; Stride et al., Citation2013). Such division might however only represent a small portion of the ecological diversity of this order as suggested by Lagkouvardos et al., who estimate that more than 180 potential family-level lineages likely exist (Lagkouvardos et al., Citation2014).
Microbiology
Simkaniaceae diversity
The Simkania genus belongs to the Simkaniaceae family, within the Chlamydiales order and consists of a single species, S. negevensis. Simkaniaceae comprise three genera: Simkania, Fritschea and Syngnamydia; the latter two genera are reported as Candidatus strains, since they have not been yet recovered by culture. Fritschea were first identified in 2003 as endosymbionts of arthropods and are subdivided into two Candidatus species: F. bemisiae and F. eriococci (Everett et al., Citation2005; Thao et al., Citation2003; Xue et al., Citation2012). Syngnamydia, which also includes two Candidatus species (S. venezia and S. salmonis), were recently described as pathogens in fishes, causing epitheliocystis, a disease characterized by large cysts in the gills (Fehr et al., Citation2013; Nylund et al., Citation2015). Additionally, seven DNA sequences recovered in environmental samples were recently described as putative members of the Simkaniaceae family based on their 16S rRNA and 23S rRNA encoding genes sequences. The first sequence (EF177461) was recovered in gastrodermal cells of Xenoturbella, a primitive marine worm (Israelsson, Citation2007). Three other sequences (FJ976094, FJ976095 and AF448723.3) were identified in environmental water samples and grouped as the cvE9 cluster (cvE38, cvE41 and cvE9) (Corsaro & Venditti, Citation2009; Corsaro et al., Citation2002). The last three were recovered from unicellular eukaryotes present in the ocean crust and from citrus plants suffering from the Yellow dragon disease (Huanglongbing), a mortal disease in citrus, caused by vector transmitted Gram-negative bacteria (Sagaram et al., Citation2009; Santelli et al., Citation2008; Sauvadet et al., Citation2011). The evolutionary relationships of Simkaniaceae members as well as related sequences is shown in .
Simkania negevensis: genome and evolution
All Chlamydiales were derived from a Chlamydiae/Planctomycetes/Verrucomicrobia common ancestor (Budd & Devos, Citation2012). Three evolutionary clusters appear to exist: (i) the Chlamydiaceae/Clavichlamydiaceae cluster, which harbors the smallest genomes and seems to have branched the earliest, (ii) the Parachlamydiaceae/Waddliaceae/Criblamydiaceae cluster and (iii) the Simkaniaceae/Rhabdochlamydiaceae cluster (Lagkouvardos et al., Citation2014). Indeed, Simkaniaceae share an 86–87% homology in the 16S rRNA encoding gene sequence with Rhabdochlamydiaceae (Kostanjsek et al., Citation2004) and only 82% homology with Chlamydiaceae (Kahane et al., Citation1995). Comparative analysis of the full genome sequences showed more than 400 conserved core genes preserved in all Chlamydiales members (Bertelli et al., Citation2010; Collingro et al., Citation2011; Pillonel et al., Citation2015). Among them, 20 are highly informative taxonomically and should be preferentially used to assign new strains at species level (Pillonel et al., Citation2015).
Figure 1. Evolutionary relationships of Simkaniaceae. The evolutionary history was inferred using the Neighbor-Joining method (Saitou & Nei, Citation1987) based on 1548 nucleotides from 15 16S rRNA sequences. Sequences were retrieved from the NCBI database using “Simkania*” as a research word. The percentage of replicate trees, in which the associated taxa clustered together in the bootstrap test (100 replicates) are shown next to the branches (Efron et al., Citation1996). The evolutionary distances were computed using the Tamura 3-parameter method (Tamura, Citation1992) and are shown as number of base substitutions per site. The rate variation among sites was modeled with a gamma distribution. Evolutionary analyses were conducted in MEGA5 after sequence analysis on Geneious 7.1.7 (Kearse et al., Citation2012).
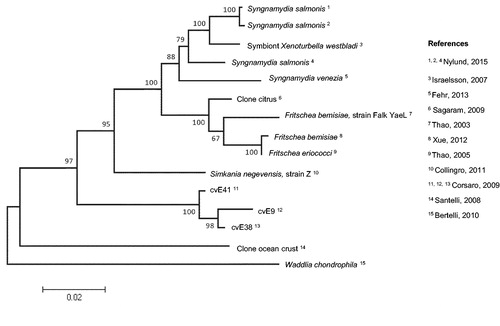
Simkania negevensis genome is characterized by a 2–3 fold larger size compared to the Chlamydiaceae members, but comparable to the genome size of Waddliaceae and Parachlamydiaceae members (Collingro et al., Citation2011), as shown in . This suggests a large biosynthetic ability, allowing adaptation to various environments. The analysis of S. negevensis genome revealed the presence of a type I intron localized in the 23S rRNA gene (Everett et al., Citation1999b). Type I introns are self-splicing motile introns, which have so far only been described in eukaryotic cells. To date, S. negevensis and Coxiella burnetii are the only bacterial species, which possess such genetic structure (Nesbø & Doolittle, Citation2003). This molecular pattern is also present in the ameba Acanthamoeba castellanii as well as in the algal chloroplasts (Everett et al., Citation1999b) and therefore may have been acquired from horizontal gene transfer. This discovery, along with the high proportion of eukaryotic-like genes in chlamydial genomes, questions the possible relationship between Chlamydiales and chloroplasts and their possible implication in the eukaryogenesis (Brinkman et al., Citation2002). The function of this intron remains unclear, but evidence showed that the intron is not spliced from the 23S rRNA and might delay growth by reducing ribosomal function (Everett et al., Citation1999b). It is not known whether this intron was also present, at first, in other Chlamydiales growing in amebae and was subsequently eliminated to improve replication or whether it was horizontally acquired after the divergence of S. negevensis from other Chlamydiales.
Table 1. General features of Simkania negevensis compared to other Chlamydiales members.
Simkania negevensis possess a 132 kb plasmid, which carries a type IV secretion system (T4SS) encoded by a tra operon. A similar tra operon is also present in the genome of Protochlamydia amoebophila (Greub et al., Citation2004a) and of Parachlamydia acanthamoebae (Greub et al., Citation2009), two members of the Parachlamydiaceae family. These operons exhibit a striking similarity with the tra genes carried by the conjugative F-plasmid of Escherichia coli. Thus, it is suspected that these chlamydial operons encode a conjugative DNA transfer system. In addition, the S. negevensis tra operon also exhibits genetic similarities to the T4SS conjugative system encoded in Rickettsia belli, where conjugation has been demonstrated (Ogata et al., Citation2006), reinforcing the putative role of the S. negevensis T4SS in DNA transfer. Independent of the presence of the T4SS, the identification of a plasmid in the S. negevensis genome is of utmost interest, as it might play a significant role in the pathogenesis of S. negevensis infection, as suggested for the 7500 kb Chlamydia spp. plasmid. Indeed, it has been shown both in vivo (Kari et al., Citation2011; O’Connell et al., Citation2007) and in vitro (Porcella et al., Citation2015) that infections with a plasmid deficient strain resulted in attenuated infections exhibiting a lower inflammatory response. An adaptive immune response was however elicited enabling protection against re-infection (Kari et al., Citation2011; O’Connell et al., Citation2007; Porcella et al., Citation2015). The mechanisms by which the chlamydial plasmid modulates the pathogenesis are still unclear, but they are probably related to: (i) direct effect of proteins encoded by the plasmid (Li et al., Citation2008; Liu et al., Citation2014, p. 201) and (ii) regulation of the expression of other virulence factors encoded by the chromosome (Carlson et al., Citation2008; Song et al., Citation2013). Interestingly, the S. negevensis plasmid encodes various proteins of interest, among which is one copy of the Macrophage Infectivity Potentiator (Mip) (Collingro et al., Citation2011). This lipoprotein is highly similar to the Mip protein of Legionella pneumophila. In Chlamydia, it has been shown to be essential for an optimal intracellular infection and to induce the production of pro-inflammatory cytokines (Bas et al., Citation2008; Lundemose et al., Citation1993a, Citationb).
Biochemical characteristics
Cell wall and surface proteins
The composition of the outer membrane is of significant importance for the pathogenesis of Chlamydiales. In the EB state, membrane proteins are implicated in the entry within the host and serve as major antigens for the immune response. In the RB state, membrane proteins may be part of the secretion systems used to corrupt the host cell whereas others may serve as porins to uptake nutrients from the host cytoplasm. A comparison of the structure of the membrane of various members of the Chlamydiales order is shown in .
The Chlamydial Outer Membrane Complex (COMC) of the Chlamydiaceae is mainly composed by the MOMP (ompA) protein and by two cystein-rich proteins, OmcA and OmcB (Caldwell et al., Citation1981). MOMP (ompA) represents 60% of the surface proteins, at least in purified EBs and serves as a porin (Bavoil et al., Citation1984). Aistleitner et al. have recently studied the structure of S. negevensis outer membrane (Aistleitner et al., Citation2015). Using a computerized proteomic approach, 65 OMPs were identified as major components of the S. negevensis EB’s membrane. Among them 37 were MOMP-like proteins, which resemble the MOMP (ompA) of Chlamydiaceae. Out of the 37 MOMP-like proteins, 30 contained beta-sheet conformations, suggesting a role as porins. Two of them demonstrated high similarities to MOMP proteins of veterinary Chlamydia species (Collingro et al., Citation2011). S. negevensis outer membrane lacks the cysteine-rich proteins (OmcA and OmcB) present in other Chlamydiales. These cysteine-rich proteins are sensitive to the redox state of the environment and they might provide some rigidity and resistance to osmotic pressure through the formation of disulfide bridges. Some authors suggested that they may replace the peptidoglycan layer present in the periplasm of other Gram-negative bacteria (Everett & Hatch, Citation1995; Sun et al., Citation2007). No homologs of OmcA and OmcB were identified in S. negevensis genome and none of the identified MOMP-like proteins could serve this function as their content in cysteine was extremely low (Aistleitner et al., Citation2015). Such differences may result in a more flexible envelope and a lack of surface proteins regulated by the redox state. Such regulations are associated with modifications of the function of several surface proteins in Chlamydiaceae through reduction and reoxidation of the cysteine residues and could play a role in the switch from the EB to the RB state. For example, the permeability of MOMP is increased once reduced (Bavoil et al., Citation1984) and the type III secretion system (T3SS) in Chlamydia is also described as influenced by the redox state (Betts-Hampikian & Fields, Citation2011).
Studying the morphology of EBs and RBs particles is difficult and has been the subject of various works. As an example, crescent bodies and star bodies were reported as infectious EB-like particles of Parachlamydiaceae and Criblamydiaceae, respectively (Lienard et al., Citation2011b; Rusconi et al., Citation2013; Thomas et al., Citation2006). Current knowledge suggests that these observations are largely influenced by the buffer and the fixatives used and therefore do not allow taxonomic discrimination, even if they correspond to some structural differences in the cell wall of the different bacterial families (Pilhofer et al., Citation2014; Rusconi et al., Citation2013). Noteworthy, S. negevensis EBs were described as highly pleomorphic particles, especially once located in the cytoplasm (Pilhofer et al., Citation2014). Such observation reinforces the hypothesis of a relative high cell wall flexibility due to the lack of cysteine-rich surface proteins in S. negevensis.
The inner membrane of most bacteria is protected by a cell wall composed of peptidoglycan. This structure is important for membrane stability as well as cell division. Until recently, it was thought that Chlamydiales were lacking peptidoglycan and their partial sensitivity to cell wall inhibitors such as penicillin was therefore not understood and considered as a paradox called the Chlamydiales anomaly. However, the presence of peptidoglycan was recently demonstrated in some members of the Chlamydiales, namely C. trachomatis, in which they were present as a ring structure at the division septum (Liechti et al., Citation2014) and P. amoebophila, which synthesizes sacculi that contain a modified form of peptidoglycan (Pilhofer et al., Citation2013). Additionally, a recent work identified two peptidoglycan-remodeling enzymes (the AmiA amidase and the NlpD endopeptidase) implicated in coordinated cell wall constriction during division. Such enzymes were shown to recognize peptidoglycan-like peptides in Chlamydiales (Frandi et al., Citation2014). Most of this work was done on Waddlia chondrophila, a Chlamydiales member that is susceptible to high dose of penicillin and also to fosfomycin, a drug active on MurA (Frandi et al., Citation2014; Jacquier et al., Citation2014). The MurA enzyme is implicated in peptidoglycan biosynthesis, suggesting the presence of peptidoglycan-like structures in at least three family-level lineages of the Chlamydiales order, namely the Chlamydiaceae, Waddliaceae and Parachlamydiaceae (Frandi et al., Citation2014; Jacquier et al., Citation2015, Citation2014). In their first report, Kahane et al. described S. negevensis as being resistant to 10 μg/ml of penicillin, as opposed to what was observed for C. trachomatis. It was, however, sensitive to cycloserine, an inhibitor of the peptidoglycan synthesis, similarly to other Chlamydiaceae. In addition, activity of the AmiA amidase and the NlpD endopeptidase was, recently, documented in S. negevensis, suggesting the presence of peptidoglycan-like structures in S. negevensis (Frandi et al., Citation2014), despite failure to isolate peptidoglycan containing sacculi in S. negevensis (Liechti et al., Citation2014). Their detection might be prevented by a restricted time-frame synthesis (Jacquier et al., Citation2015) or might require an additional method, similar to the one recently described by Packiam et al., which allowed the isolation of peptidoglycan fragments in C. trachomatis (Packiam et al., Citation2015).
Secretion systems
Intracellular bacteria parasitize their host’s metabolic pathways to their own advantage. In parallel, they must inhibit the trigger of the host’s immune system. One strategy consists in secreting various proteins into the host cytoplasm or environment through secretion systems (Greub, Citation2013). S. negevensis encodes a type III secretion system (T3SS) (Collingro et al., Citation2011). Hsia et al. first suggested the existence of a type III secretion system in Chlamydia (Hsia et al., Citation1997), which was likely present in the Chlamydiales common ancestor given its presence in every genome sequenced so far (Bertelli et al., Citation2015, Citation2010; Greub et al., Citation2009; Horn et al., Citation2004; Hovis et al., Citation2013; Stephens et al., Citation1998), as summarized in . The T3SS appears as a needle inserted in the inner and outer membranes of the bacterium, which protrudes into the cytoplasm of the cell through the inclusion membrane (Pilhofer et al., Citation2013). Although, the structure of the T3SS is highly conserved among Chlamydiales, effectors secreted through this system largely vary among families and genera (Collingro et al., Citation2011). Interestingly, S. negevensis does not possess gene encoding for major chaperones of the T3SS, namely the SctF, CdsF, CT666 and the Specific Chlamydiales Chaperon 3 (SCC3), which are conserved in Waddliaceae, Parachlamydiaceae and Chlamydiaceae (Collingro et al., Citation2011).
As mentioned above, S. negevensis plasmid encodes a T4SS. In addition to its likely role in plasmid propagation and DNA exchange, it may also allow secretion of proteins and may represent an important virulence factor.
Metabolism
Strict intracellular bacteria are often characterized by their important lack of metabolic pathways and the necessity to parasitize their hosts for nutrients and energy. However, genomic studies have revealed extended metabolic capabilities of S. negevensis compared to Chlamydiaceae (Collingro et al., Citation2011; Omsland et al., Citation2014). This extended metabolic capacity has also been observed for other Chlamydia-related bacteria exhibiting large genomes (Bertelli et al., Citation2010; Collingro et al., Citation2011; Greub et al., Citation2009; Horn et al., Citation2004), as shown in . Among major differences, we note their ability to directly use glucose for the glycolysis pathway due to the presence of a glucokinase, making them independent from the host cell glucose-6-phosphate (Bertelli et al., Citation2010; Collingro et al., Citation2011; Greub et al., Citation2009; Omsland et al., Citation2014). Additionally, they possess a complete citric acid cycle as opposed to Chlamydiaceae, which lack important enzymes and need a constant exchange with the host cell to produce energy by oxidation of acetyl-CoA (Omsland et al., Citation2014). In S. negevensis, entry in the citrate cycle can occur: (a) through acetyl-CoA produced from pyruvate following glycolysis and (b) through the transformation of asparagine in fumarate (Collingro et al., Citation2011; Omsland et al., Citation2014). Moreover, Waddliaceae, Parachlamydiaceae and Simkaniaceae are able to synthetize NAD + from nicotinamide (Bertelli et al., Citation2010; Omsland et al., Citation2014) and S. negevensis can, additionally, use asparagine as a source of NAD+ (Collingro et al., Citation2011; Knab et al., Citation2011; Omsland et al., Citation2014). Finally, an in vitro experiment by Kahane et al. suggests that S. negevensis is able to grow in a glucose free medium; indeed the number of infectious forming units was similar using a Roswell Park Memorial Institute (RPMI) media depleted in glucose and supplemented with 1% glucose (Kahane et al., Citation1999). Taken together, these findings suggest that S. negevensis is less dependent from the host cell in term of energy production compared to other Chlamydiales (Omsland et al., Citation2014).
Similarly to Chlamydiaceae, S. negevensis is unable to synthesize nucleotides de novo and relies on nucleotides transporters for their uptake. Four isoforms of nucleotides transporters have been identified in S. negevensis: SnSTT1, an ADP/ATP antiporter; SnSTT2, a guanine/ATP/H + symporter; SnSTT3 a global nucleotide triphosphate antiporter, also able to transport deoxy-CTP (dCTP). The function of the last transporter, SnSTT4, is unknown (Knab et al., Citation2011). The ability to transport dCTP is unique among prokaryotes and might represent a selective metabolic advantage for S. negevensis, favoring its relative growth in environments rich in cytosine.
Regarding amino-acid biosynthesis, S. negevensis is able to synthesize tyrosine, phenylalanine, proline, alanine, aspartate and glutamate (Collingro et al., Citation2011; Omsland et al., Citation2014). In addition, S. negevensis possesses a complete tryptophan operon (trp operon) (Collingro et al., Citation2011; Omsland et al., Citation2014). Some pathogenic Chlamydiaceae, notably the genitourinary strains of C. trachomatis, are partially capable of synthesizing this metabolite but enzymes required in this pathway are completely absent in other Chlamydia-related bacteria (Carlson et al., Citation2006; Omsland et al., Citation2014). It appears that tryptophan plays an important role in the pathogenesis of Chlamydia spp. infections. Tryptophan is, indeed, an important regulator of the chlamydial development cycle (Bonner et al., Citation2014). In case of tryptophan depletion, the expression pattern changes and forces the bacteria into a persistent form (Baud et al., Citation2008; Brunham & Rey-Ladino, Citation2005; Ibana et al., Citation2011). Tryptophan depletion can be induced in humans by IFN-γ production (Taylor & Feng, Citation1991). Thus, S. negevensis might be less sensitive to IFN-γ than Chlamydiaceae, which could play a role in the pathogenesis.
Culture characteristics
Cell permissiveness and growth cycle
S. negevensis is able to grow and survive in vitro in mammalian cells such as Vero cells (Kahane et al., Citation1993), epithelial cells from the respiratory and genitourinary tract, endothelial cells (Kahane et al., Citation2007a) as well as macrophages (Kahane et al., Citation2008). Growth is also described in arthropods (both in vitro and in vivo) (Croxatto et al., Citation2014; Sixt et al., Citation2012) and amebae (Kahane et al., Citation2001).
Similarly to other Chlamydiales, S. negevensis growth cycle is characterized by the presence of two developmental stages: one large replicative form, the Reticulate Body (RB), and one small compact form, which resembles to electron dense Elementary Body (EB) form of Chlamydiaceae. These two forms can be selectively fractionated on density gradients (Kahane et al., Citation1999, Citation1993). The high density of EBs is likely due to the presence of condensed DNA as shown by the presence of filamentous material on electron microscopy tomography (EMT). Even though gene expression might be downregulated through DNA condensation, EB particles are likely to be metabolically active as suggested by the presence of ribosomes (Pilhofer et al., Citation2014).
The growth cycle of S. negevensis is characterized by a production phase lasting approximately three days, during which particles are produced in an exponential way. During that time, the cytoplasm of the host cells shows increasing dark perinuclear patches. This is followed by a plateau phase, during which no or few particles are produced, but instead the particles previously produced are stored in multiple large cytoplasmic vacuoles of the host cells and cytopathic changes are observed. Based on culture observations, S. negevensis does not seem to induce cell lysis, or at least not to the same extent as what is observed for other members of the Chlamydiales order, such as W. chondrophila (Kebbi-Beghdadi et al., Citation2011). In comparison, C. trachomatis particles are released between 30 and 68 h post-infection depending on the strain (Miyairi et al., Citation2006), W. chondrophila particles are released 48–72 h upon cell lysis (Goy et al., Citation2008) and P. acanthamoebae induces lysis of infected amebae after 72–96 h depending on the initial multiplicity of infection (MOI) and incubation temperature (Greub & Raoult, Citation2002b; Greub et al., Citation2003b). However, despite the apparent absence of cell lysis, some particles seem to be released in the extracellular environment, as suggested by the presence of S. negevensis particles in the supernatant of infected insect cell cultures capable of inducing a second round of infection (Sixt et al., Citation2012). Recently, Hybiske et al. showed that C. trachomatis may exit from the host cell through the extrusion of a membrane-bound compartment (Hybiske & Stephens, Citation2007). This extrusion depended on actin polymerization, as well as on various proteins implicated in the remodeling of the cytoskeleton. A similar mechanism might be implicated in the extrusion of S. negevensis.
Interestingly, it has been proposed that S. negevensis RBs might be infectious, an aspect that has not been observed for other Chlamydiales so far. Indeed, Kahane et al, observed similar growth kinetics of S. negevensis purified EBs and RBs (Kahane et al., Citation2002). However, this has not been confirmed yet and further investigations are needed before making any definite conclusions.
The Simkania containing vacuole and its interaction with cellular organelles
S. negevensis replicates in a single intracellular vacuole hereafter called the Simkania containing vacuole (SCV). Interestingly, the SCV is closely associated with the endoplasmic reticulum (ER) of the host cell, being almost completely surrounded by the typical ribosomes-covered ER membrane (Mehlitz et al., Citation2014; Pilhofer et al., Citation2014). Such ER association was observed in infected epithelial cells, macrophages-derived cells and amebae, emphasizing the strong conservation of the phenotype. Intracellular traffic of S. negevensis clearly differs from P. acanthamoebae, which survives in the late endosome (Greub et al., Citation2005) and from C. trachomatis, which intercepts Golgi-derived vesicles and causes Golgi apparatus fragmentation (Heuer et al., Citation2009). Recruitment of the ER also occurs upon infection with other members of the Chlamydiales order, such as C. trachomatis and W. chondrophila, but to a lesser extent than what is observed following S. negevensis infection (reviewed in Derré, Citation2015). The mechanisms by which S. negevensis induces this tight association remain to be elucidated, but might rely on specific inclusion proteins or type III effector proteins. Indeed, Chlamydia spp. use their IncD protein to recruit the host ER-CERT protein to acquire ceramide (Derré et al., Citation2011). A recent work suggested that early retrograde transport is required to achieve an optimal S. negevensis infection, which seems to be implicated both in the SCV maturation, as well as in lipids uptake (Herweg et al., Citation2016). This was shown by the enrichment, at the SCV membrane, of Clathrin and COP1 related proteins, typical of the early endosomes and trans-Golgi network (TGN). This hypothesis is further supported by the reduced bacterial replication observed in the presence of specific retrograde transport inhibitors. In addition, ceramide vacuolar uptake was modified by these inhibitors, suggesting that S. negevensis has developed a mechanism to directly obtain ceramides from the Golgi.
The tight association observed between the SCV and the ER raises the question of activation of the ER-stress, which plays a significant role in limiting viral replication. Interestingly, S. negevensis is capable of inhibiting the ER-stress response despite a strong initial activation, and thus promoting host survival and indirectly promoting S. negevensis replication (Mehlitz et al., Citation2014).
In addition to the strong ER association, some association with the mitochondria was observed in mammal's cells in mammal’s cells, similarly to what is observed for W. chondrophila (Croxatto & Greub, Citation2010) and C. psittaci (Mehlitz et al., Citation2014). However, the phenotype of mitochondria association to the replicative vacuole is much stronger for W. chondrophila, which recruits mitochondria as early as 3 h post-infection using a redundant mechanism based on both microtubules and actin microfilaments (Croxatto & Greub, Citation2010). Mitochondria might represent an additional source of lipids, as well as ATP (Croxatto & Greub, Citation2010). It is still unclear whether S. negevensis has developed a specific mechanism to recruit the mitochondria, or if their association to the SCV is related to their natural association with the ER.
Epidemiology
Prevalence
Scarce data on the prevalence of Simkania are available in the literature, most of them provided by old studies. Caution should be taken when interpreting these results. The seroprevalence appears high in the Middle East (Israel and Jordanian), ranging from 55 to 65% among healthy adults and reaching 80% in Bedouins (Al-Younes & Paldanius, Citation2014; Friedman et al., Citation1999). Serological evidence of past infection was documented in 46% of pregnant women in Cornwall, UK (Friedman et al., Citation2006) and in 41% of Danish blood donors (Johnsen et al., Citation2005). In contrast, an overall prevalence of only 23.5% was observed among children and adults in Brooklyn, USA (Kumar et al., Citation2005) and only 4.5% of the population tested in Japan were seropositive, a low prevalence observed despite the low cutoff titer of 1:8 used in the study (Yamaguchi et al., Citation2005). The large discrepancy between these results could be explained by geographical differences in term of exposure and risk factors. However, the heterogeneity of diagnostic tools used between the studies makes comparisons difficult. Indeed, the prevalence observed in the Danish and Jordanian population was obtained using an immunofluorescence assay with a cutoff titer of 1:16 and 1:8, respectively, which probably overestimated the prevalence (Al-Younes & Paldanius, Citation2014; Johnsen et al., Citation2005). Nevertheless, the relatively high prevalence in Israel, England and USA was observed using the same ELISA assay based on purified EBs (Friedman et al., Citation2006, Citation1999; Kumar et al., Citation2005). Authors tested a 1/100 diluted serum and considered an OD (optical density) higher than 0.5 as positive. In conclusion, serological evidence of human exposure to Simkania infection was documented worldwide and prevalence seems to increase with increasing age (Friedman et al., Citation2006; Johnsen et al., Citation2005; Kumar et al., Citation2005).
Transmission and reservoir
S. negevensis was discovered as a cell culture contaminant. However, the natural host of Simkaniaceae remains unknown. As mentioned above, S. negevensis is able to grow in vitro in a wide range of hosts (i.e. mammalian cells, arthropods, amebae) (Kahane et al., Citation2008, Citation2007a, Citation2001; Sixt et al., Citation2012). Evidence of Simkaniaceae was documented in vivo in ticks (Croxatto et al., Citation2014; Pilloux et al., Citation2015) and in granulomatous lesions in reptiles (Soldati et al., Citation2004). Fritschea were discovered in arthropods (Everett et al., Citation2005) and Syngnamydia in fish (Fehr et al., Citation2013; Nylund et al., Citation2015). Additionally, various Simkania-related DNA sequences were amplified from marine environments (Israelsson, Citation2007; Santelli et al., Citation2008; Sauvadet et al., Citation2011) and in one case in vegetal cells (Sagaram et al., Citation2009). This wide host range is quite impressive and contrasts with the narrow host range of Chlamydia trachomatis (human-specific) and Chlamydia felis (cat-specific).
Various hypotheses on the reservoir of Simkaniaceae and their mode of transmission to humans have been proposed. Firstly, contaminated water might be a source of human infection, either as a free-living organism or through infected amebae. Indeed, a report showed that S. negevensis infectivity in vitro was still preserved after a seven-day incubation of free particles in distilled water and was even increased in cases of co-infection with amebae (Kahane et al., Citation2004). In comparison, the infectivity of C. trachomatis, considered as a sexually transmitted disease, was completely abolished after 5 h of incubation in the same conditions (Kahane et al., Citation2004). Moreover, co-culture of macrophages with S. negevensis-infected amebae resulted in the rapid death of the amebae and infection of the macrophages (Kahane et al., Citation2008). Amebae could therefore serve as reservoir and contribute to the selection of virulence traits (Greub & Raoult, Citation2004; Lamoth & Greub, Citation2010). This is in agreement with the identification of Simkania and amebae’s antigens in drinking water and in reclaimed wastewater used to irrigate crop in Israel (Donati et al., Citation2015; Kahane et al., Citation2004). Similarly, Simkaniaceae DNA was amplified from environmental water in Western Europe (Corsaro & Venditti, Citation2009; Corsaro et al., Citation2002) and, recently, in Italian swimming pools (Donati et al., Citation2015; Pérez et al., Citation2011, Citation2012). Transmission to humans through water is also suggested by a prospective study, in which it was possible to identify the presence of Simkania in the corresponding drinking water of children suffering from a Simkania-associated pneumonia in as much as 76.5% of cases. In this study, results were only considered as positive when congruent results were obtained by at least two different approaches (Nested PCR, culture in Vero cells and/or membrane enzyme immuno-assay (MEIA)) (Kahane et al., Citation2007b). Nevertheless, these results should be treated with caution as (i) only 34 children were included in the study and (ii) nested PCR contamination cannot be excluded due to the very high proportions of positive results observed in the study. Finally, an oral transmission of S. negevensis is likely since (i) a significant IgA response to Simkania was observed in patients with gastro-intestinal symptoms (Donati et al., Citation2013) and (ii) S. negevensis may replicate in vitro within gastro-intestinal cell lines (Kahane et al., Citation2007a).
Arthropods, such as ticks, might represent another source of infection. Vector transmission is quite common for other intracellular bacteria such as Rickettsia (Walker & Ismail, Citation2008). Moreover, Simkania-associated DNA was amplified from Swiss Ixodes ricinus ticks, though with a lower prevalence and amount than Rhabdochlamydiaceae (Croxatto et al., Citation2014; Pilloux et al., Citation2015).
Additional hypotheses of transmission include direct human to human transmission through aerosols or droplets due to the association with respiratory diseases (see pathogenic potential, hereafter), as well as sexual contacts or contacts with animals, which were described for other Chlamydiales (Baud & Greub, Citation2011; Baud et al., Citation2007; Gottlieb et al., Citation2013; Knittler & Sachse, Citation2015; Schachter, Citation1986). Sexual contact seems unlikely due to the high seroprevalence in children and the absence of cross-prevalence with C. trachomatis (Friedman et al., Citation1999). Finally, a zoonotic transmission has so far not been documented, but cannot be excluded.
Pathogenic potential
In vitro
Several in vitro aspects of S. negevensis infection suggest an important pathogenic potential. First, S. negevensis is able to grow and survive within macrophage-type cell line U937 (Kahane et al., Citation2008). Moreover, uninfected epithelial cells can be actively infected after co-culture with infected macrophages (Kahane et al., Citation2008). Interestingly, S. negevensis is resistant in vitro to LL-37 cathelicidin human peptide present in phagocyte cells, but is highly sensitive to five other animal cathelicidins (Donati et al., Citation2011). Moreover, Karunakaran et al. demonstrated that S. negevensis prevents TNF-α-induced apoptosis in host cells through the inhibition of the release of mitochondrial caspase-activating proteins (Karunakaran et al., Citation2011). This is similar to what is known for the pathogenic C. trachomatis and C. pneumoniae (Fan et al., Citation1998). However, the phenotype seems to be stronger in S. negevensis as it could be observed even at a very low MOI (MOI = 0.5) (Fan et al., Citation1998; Karunakaran et al., Citation2011). This anti-apoptotic capability represents an important virulence factor and is not observed in all Chlamydiales. For example, Parachlamydiaceae appear to instead induce apoptosis in macrophages (Greub et al., Citation2003c) and in insect cell lines (Sixt et al., Citation2012). The ability to inhibit apoptosis and the absence of cell lysis might allow S. negevensis to persist within its host and establish a persistent latent infection. Such infection might then be reactivated and might represent an important pathogenic mechanism.
Two types of S. negevensis infection have been described: (i) an active infection with production of new bacterial particles and observation of cytopathic effects in the host and (ii) a persistent infection without any cytopathic effects (Kahane et al., Citation2007a). In persistent infection, mostly RBs of atypical morphology were visualized within the infected cells. Persistence of infectious particles within the host was demonstrated by inoculation of uninfected Vero cells with persistently infected cells, which produced an active infection. Infection was however less efficient compared to inoculation with actively infected cells.
In Chlamydia spp., persistence may be induced by iron depletion (Raulston, Citation1997), IFN-γ (Beatty et al., Citation1994; Rottenberg et al., Citation2002) and penicillin treatment (Matsumoto & Manire, Citation1970). With S. negevensis, a latent infection can be observed either spontaneously in specific cell lines, namely intestinal or genitourinary lines, or could be induced by iron depletion. Reactivation was then possible after serial trypsin treatment (Kahane et al., Citation2007a). Interestingly, reactivation was also observed when persistently infected cells were cultured with macrophages-derived cells. The mechanism, by which reactivation is induced, is still unclear, but might rely on recognition of the infection by the macrophages and production of cytokines, which could promote the release of infectious S. negevensis particles (Kahane et al., Citation2008). In addition, an inflammatory response was observed in both types of infections, mostly through the production of IL-8 and IL-6 (Kahane et al., Citation2007a).
Lessons from the clinic
Since the discovery of S. negevensis, only few studies have been conducted to evaluate the implication of Simkania spp. in human diseases (see list of all studies in supplementary materials). An implication in respiratory diseases, particularly in bronchiolitis and pneumonia in children and young adults, is likely, as shown in and , respectively, which summarize the findings of major clinical studies. Thus, a significant association between Simkania and acute bronchiolitis was shown in children from Israel (Kahane et al., Citation1998). The authors used both a PCR and a cell culture approach to detect the organism in nasopharyngeal samples. A total of 239 patients and 78 controls were included over a period of two years. Of the 116 patients, from whom a possible etiologic agent was isolated, 60 were positive for Simkania by at least one of the techniques used. This was the second most common agent identified after respiratory syncitial virus (RSV) and statistically different from controls. Clinical findings were similar to patients infected by RSV. Similar results were also obtained in a study performed on English children with bronchiolitis. In 26% of the patients, evidence of a Simkania infection was provided by both PCR and culture. This rate reached 45% when considering only samples positive by PCR. However no controls were included in this study (Friedman et al., Citation2006).
Table 2. Prevalence of an acute Simkania negevensis infection in patients suffering from bronchiolitis.
Table 3. Prevalence of an acute Simkania infection in patients suffering from pneumonia.
Various works showed evidence of an acute infection with Simkania in cases of pneumonia in children and young adults (Donati et al., Citation2011; Fasoli et al., Citation2008; Heiskanen-Kosma et al., Citation2008; Kahane et al., Citation2007b; Lieberman et al., Citation1997; Lienard et al., Citation2011a; Nascimento-Carvalho et al., Citation2009). Acute infection was indicated either by a rise in paired sera of IgG, IgA or IgM defined as a four-fold increase of antibody titers observed by micro-immunofluorescence (Fasoli et al., Citation2008; Heiskanen-Kosma et al., Citation2008; Nascimento-Carvalho et al., Citation2009) or a rise of 0.5 OD in the ELISA signal (Lieberman et al., Citation1997), or by elevated titers of IgM or IgA or direct identification of Simkania through PCR, culture or antigen detection (Kahane et al., Citation2007b). Globally, a low prevalence of acute infection was observed (2–10%), except in the study performed by Kahane et al., in which Simkania was identified in almost all samples, including controls. Evidence of co-infections with other putative pathogens was found in 40–60% of the cases; the most commonly associated pathogens were Mycoplasma pneumoniae, RSV, Streptococcus pneumoniae and Chlamydia pneumoniae (Fasoli et al., Citation2008; Lieberman et al., Citation1997; Nascimento-Carvalho et al., Citation2009). Noteworthy, the association of Simkania infection with the occurrence of bronchiolitis or pneumonia was not confirmed in a study performed on children, in Brooklyn, USA (Kumar et al., Citation2005).
An association between Simkania infection and other respiratory diseases was also studied. However, no association was found with asthma (Korppi et al., Citation2006; Kumar et al., Citation2005), nor with exacerbation of Chronic Obstructive Pulmonary Disease (COPD) (Lieberman et al., Citation2002), chronic cough (Johnsen et al., Citation2005) or acute lung rejection (Husain et al., Citation2007). Results are difficult to interpret owing to low statistical power of these studies. However, a significant correlation with serological evidence of an acute infection was shown in unspecified respiratory tract infection among adults, in England (Friedman et al., Citation2006) and a higher prevalence of Simkania was documented in patients with a lung transplant as compared to other immunocompromised patients or immunocompetent patients (Husain et al., Citation2007).
Caution should be taken when interpreting the results since only few studies included a control group. In addition, the same group from the Ben-Gurion University of the Negev, in Israel, discovered S. negevensis and performed most of the studies suggesting a pathogenic role. The specificity of the diagnostic tools might have not been optimal and contamination could have occurred within the laboratory. Indeed, the prevalence observed in the studies performed by this group (26% in Israel (Kahane et al., Citation1998), 63.6% in Inuit patients (Greenberg et al., Citation2003) and 80–97.5% in lung transplants (Husain et al., Citation2007)) are strikingly high, even in the control groups (Kahane et al., Citation2007b; Kumar et al., Citation2005).
Moreover, recent studies performed by other groups have failed to confirm such high prevalence. Indeed, using a specific quantitative PCR, Niemi et al. could not identify any positive case of Simkania infection in 97 respiratory samples taken from adult patients with a suspected respiratory tract infection (Niemi et al., Citation2011). Similarly, only two samples from Swiss children with bronchiolitis were identified as positive for a Simkaniaceae infection by a validated pan-Chlamydiales quantitative PCR (Lienard et al., Citation2011a) and only one case of a co-infection with Simkania and C. psittaci was identified in a large German study investigating community acquired pneumonia (Dumke et al., Citation2015). This discrepancy between the results could either be due to differences in the reliability of the diagnostic techniques used or to geographical variations of the prevalence of the infection.
Diagnosis
Various techniques have been described to diagnose a S. negevensis infection, reviewed by Corsaro et al. (Corsaro & Greub, Citation2006). Most of them were developed in the early 2000s. They may now lack specificity due to the recent discovery of novel Chlamydiales species.
Direct identification of Simkania in clinical samples
PCR
PCR is currently the technique of choice to diagnose C. trachomatis or C. pneumoniae infections. Diagnostic real-time PCRs have also been developed and are routinely used in our laboratory to detect W. chondrophila, P. acanthamoebae and P. neagleriophila in clinical samples (Casson et al., Citation2008a, Citationb; Goy et al., Citation2009). By analogy, this technique should be preferentially used to detect Simkania spp. Most of the primers and probes developed earlier, which are listed in , are based on the detection of amplicons on agarose gel, a technique with a lower sensitivity and specificity than real-time PCR, at risk of contamination by amplicons. Thus, we currently recommend the use of a pan-Chlamydiales PCR (Lienard et al., Citation2011a) followed by sequencing to detect putative cases of infection.
Table 4. PCR used to detect Simkania in clinical and environmental samples.
Culture
Isolation of organisms through culture is crucial to prove the presence of viable bacteria in clinical samples and to provide a reference strain for a precise downstream characterization, as well as to provide antigens for serological assays. Simkania spp. may be difficult to recover from clinical samples through culture, as it requires cell culture, which is at risk of contamination by other bacteria present in the flora of non-sterile samples such as sputa, nasopharyngeal swabs or vaginal swabs. Owing to these culture difficulties, the sensitivity of culture seems to be lower than PCR (Corsaro & Greub, Citation2006; Kahane et al., Citation1998).
Culture in Vero cells is currently the method of choice to isolate Simkania spp. in clinical samples (Corsaro & Greub, Citation2006), although amoebal co-culture might represent an ideal alternative for respiratory samples, given the non-susceptibility of amebae to Mycoplasma and to most bacteria present in the physiological oropharynx flora (Greub et al., Citation2004b; Jacquier et al., Citation2013). Testing the cells used for cell culture for the presence of a possible contamination should be routinely done; this screening should be able to detect Mycoplasma spp. as well as Chlamydiales, including S. negevensis, since the later was first isolated as a cell culture contaminant. We recommend microscopy examination of cell lines and a Pan-Chlamydiales PCR (Lienard et al., Citation2011a), when contamination is suspected.
Others
Immunohistochemistry based on rabbit or mouse anti-S. negevensis antibodies may be used to visualize this bacterium in lesions when biopsies are available. By analogy with other Chlamydiales, Gram staining should be avoided since the results are inconsistent, as EBs result generally Gram-positive, whereas RBs result Gram-negative (Lamoth & Greub, Citation2010).
Serological studies
Micro-immunofluorescence (MIF) is currently the technique of choice to detect anti-C. pneumoniae specific antibodies (Dowell et al., Citation2001). By analogy, this technique should also be applied to detect anti-Simkania spp. specific antibodies. Current recommendations for Chlamydia and Chlamydia-related bacteria propose a cutoff titer of ≥1:64 for IgG to confirm a past infection and a cutoff of ≥1:32 for IgM for an acute infection (Corsaro & Greub, Citation2006). Acute infections may also be documented based on seroconversion defined as an increase in IgG titer from 0 to ≥1:64 in paired sera or a ≥4-fold rise in the IgG titer between acute- and convalescent-phase sera (Corsaro & Greub, Citation2006). Such recommendations should also be applied to detect a Simkania infection in order to enable inter-laboratories comparison of obtained results. Alternatively, an ELISA assay developed in our laboratory to detect W. chondrophila (Lienard et al., Citation2014) could be adapted for Simkania infections. Such ELISA might be a good alternative, as the results are not biased by subjective analysis. Nevertheless, we do not recommend using the assay developed by Lieberman et al. (Citation1997) since it has not been properly validated and may overestimate true seroprevalence.
Treatment and prevention
Antibiotic susceptibility
So far, Simkania infections have been treated empirically with macrolides, similar to other Chlamydia infections. Pneumonia was indeed successfully treated with a regimen of erythromycin (Lieberman et al., Citation1997). However, in vitro experiments evaluating antibiotic susceptibility are lacking. Long-term in vitro treatment (four months) with either rifampicin or azithromycin was shown to eliminate infectivity; however, DNA was still detectable and reactivation of the infection was possible with trypsin treatment after exposure to rifampicin (Kahane et al., Citation2007a). A treatment by doxycycline might alternatively be proposed for Simkania spp. infections based on the fact that it is one of the recommended treatment of Chlamydia and Chlamydia-related infections (de Barsy et al., Citation2014; Goy & Greub, Citation2009; Hammerschlag & Kohlhoff, Citation2012; Vouga et al., Citation2015). Similarly to P. acanthamoebae and N. hartmannellae (de Barsy et al., Citation2014; Vouga et al., Citation2015), S. negevensis is resistant to quinolones (Casson & Greub, Citation2006). This resistance is probably due to a mutation in two quinolones resistance-determining regions (QRDR) as shown by genomic analysis, namely in the QRDR of GyrA and ParC, respectively (Casson & Greub, Citation2006; de Barsy et al., Citation2014). Studies reported a resistant phenotype to β-lactams derivatives (Kahane et al., Citation1993). However, high concentrations of β-lactams induce the development of aberrant bodies in W. chondrophila (Jacquier et al., Citation2014), despite the fact that it is traditionally considered resistant to β-lactams (de Barsy et al., Citation2014). The recent discovery of peptidoglycan among Chlamydiales may explain this finding (Jacquier et al., Citation2015). A similar phenotype might be observed upon exposure of S. negevensis to high dose of β-lactams and might induce a partial bacteriostatic effect despite apparent resistance at lower concentrations.
Future challenges and conclusions
Simkania negevensis, an emerging pathogen?
So far the pathogenic role of S. negevensis is difficult to hammer out, due to the lack of standardized studies. Although a high serological prevalence was often observed, direct detection of the pathogen or evidence of acute infections was rare. These findings are similar to what is observed for C. pneumoniae, whose pathogenic role is nevertheless commonly accepted. Recent studies reported a prevalence of C. pneumoniae infection of <2% in patients suffering from community acquired pneumonia (Dumke et al., Citation2015; Pletz et al., Citation2011; Senn et al., Citation2011; Wellinghausen et al., Citation2006). In addition, the correlation between serology and direct identification of C. pneumoniae through PCR is not good (Wellinghausen et al., Citation2006) and might be explained by a delay (2–3 weeks) in the apparition of IgM (Kuo et al., Citation1995). Thus, recent studies evaluating the role of S. negevensis infection in pneumonia using MIF might have missed some of the cases. Therefore, an implication of Simkania in respiratory diseases, especially in children and young adults, cannot be ruled out and requires further investigations. Despite the questionable reliability of some of the older studies that did not include controls, cases of Simkania infection were identified even in recent studies, either as the only putative pathogen or with other organisms (Dumke et al., Citation2015; Fasoli et al., Citation2008; Heiskanen-Kosma et al., Citation2008; Nascimento-Carvalho et al., Citation2009). The identification of a few patients with either a specific Simkania-associated pneumonia or bronchiolitis suggests that it might be effectively a true pathogen and might be problematic in endemic regions. summarizes the clinical findings of these patients. Physicians practicing in such regions, especially in Middle-east, should be aware of the existence of Simkania. In addition, infection might be promoted in cases of reduction of host defenses, either by a co-infection or by reduction of the mucociliary function. No information on the prevalence of Simkania infection in hospitalized patient is available, but, similarly to C. pneumoniae (Steinhoff et al., Citation1996), the prevalence might be higher due to increased proportion of immunocompromised patients.
Table 5. Patients with Simkania-caused pneumonia.
Since other members of the Chlamydiales order including C. trachomatis and W. chondrophila have been associated with miscarriages (Baud et al., Citation2007, Citation2011b), a possible association of S. negevensis with adverse pregnancy outcomes should be investigated. In addition, pregnancy represents an immunotolerant state where S. negevensis could find an opportunity to reactivate (Alijotas-Reig et al., Citation2014; Kropf et al., Citation2007).
Simkaniaceae, toward a better comprehension of the Chlamydiales
Simkania negevensis harbors specificities not present in other Chlamydiales, notably, (i) the existence of a conjugative plasmid, which could promote genetic variations in this strict intracellular bacterium and (ii) a type-I intron suggesting a common evolutionary history with amebae and/or plant plastids. In addition, the distinct features of S. negevensis membrane and the specificities of its metabolic pathways could help in understanding the biology and evolution of this fascinating order.
In conclusion, S. negevensis represents a Chlamydia-related bacterium of high interest as it provides new insights into the biology and evolution of the Chlamydiales order. An implication in human diseases, particularly in respiratory tract infections in children and young adults, is suspected. Clinicians should thus consider S. negevensis in the differential diagnosis of respiratory tract infections and should search this pathogen using a pan-Chlamydiales PCR (Lienard et al., Citation2011a) or using another specific molecular test, that will be available in the near future. We recommend an antibiotic treatment with macrolides based on previous empirical observations, but doxycycline might represent a good alternative.
Search strategy and selection criteria
We searched PubMed for articles published from 1 January 1990 to 30 September 2015 with the term “Simkania*” to identify both “Simkania” and “Simkaniaceae” and identified 81 published articles. We did not search for articles older than this date range as the first report describing Simkaniaceae was published in May 1993. We also reviewed relevant references cited in these articles, as well as articles referring to the “microorganism Z”, which was the first name of S. negevensis. In addition, we searched the NCBI nucleotide database with the terms “Simkania” and “Simkaniaceae” to recover potential related sequences.
Summary points
Simkania negevensis is a novel Chlamydia-related bacterium and the founding member of the Simkaniaceae family within the Chlamydiales order.
S. negevensis is able to replicate within a wide range of hosts including amebae, arthropods, mammalian cells and reptiles.
Potential virulence factors include the presence of a conjugative plasmid, as well as a type III and a type IV secretion systems. Pathogenesis may include the ability to induce persistent latent infections.
S. negevensis possess a type I intron in the 23S rRNA encoding gene suggesting a common evolutionary history with amebae and/or plant plastids.
Evidence of human exposition has been described worldwide. Transmission may occur through contaminated water.
S. negevensis might represent an emerging pathogen associated with bronchiolitis and pneumonia in children and young adults.
Current diagnosis methods comprise PCR, culture with Vero cells or amebae and serological assays.
Macrolides are the treatment of choice. Quinolones should be avoided, as S. negevensis is naturally resistant. Caution should be made when using penicillin as it may induce a persistent stage.
Supplementary_clinical_studies_revised.pdf
Download PDF (357.5 KB)Disclosure statement
The authors report no declarations of interest.
Funding
Manon Vouga is founded through the MD-PhD grant by the Swiss National Science Foundation (SNSF) (no. 323530_158123). David Baud is supported by the Department “Femme-Mère-Enfant”, by the “Fondation Leenaards” through the “Bourse pour la relève académique”, by the Divesa Foundation and by the SNSF (no. 310030_156169/1). Gilbert Greubãs research is supported by various grants including grants from the SNSF (no 310030-162603 & Synergia CRSII3-141837).
References
- Aistleitner K, Anrather D, Schott T, et al. (2015). Conserved features and major differences in the outer membrane protein composition of Chlamydiae. Environ Microbiol 17:1397–413.
- Alijotas-Reig J, Llurba E, Gris JM. (2014). Potentiating maternal immune tolerance in pregnancy: a new challenging role for regulatory T cells. Placenta 35:241–8.
- Al-Younes HM, Paldanius M. (2014). High seroprevalence of Simkania negevensis in Jordan. Braz J Microbiol 45:1433–7.
- Asner SA, Jaton K, Kyprianidou S, et al. (2014). Chlamydia pneumoniae: possible association with asthma in children. Clin Infect Dis 58:1198–9.
- Bartlett EC, Levison WB, Munday PE. (2013). Pelvic inflammatory disease. BMJ 346:f3189.
- Bas S, Neff L, Vuillet M, et al. (2008). The proinflammatory cytokine response to Chlamydia trachomatis elementary bodies in human macrophages is partly mediated by a lipoprotein, the macrophage infectivity potentiator, through TLR2/TLR1/TLR6 and CD14. J Immunol Baltim Md 180:1158–68.
- Baud D, Goy G, Jaton K, et al. (2011a). Role of Chlamydia trachomatis in miscarriage. Emerg Infect Dis 17:1630–5.
- Baud D, Goy G, Osterheld MC, et al. (2011b). Waddlia chondrophila: from bovine abortion to human miscarriage. Clin Infect Dis 52:1469–71.
- Baud D, Goy G, Osterheld MC, et al. (2014). Role of Waddlia chondrophila placental infection in miscarriage. Emerg Infect Dis 20:460–4.
- Baud D, Greub G. (2011). Intracellular bacteria and adverse pregnancy outcomes. Clin Microbiol Infect 17:1312–22.
- Baud D, Regan L, Greub G. (2008). Emerging role of Chlamydia and Chlamydia-like organisms in adverse pregnancy outcomes. Curr Opin Infect Dis 21:70–6.
- Baud D, Thomas V, Arafa A, et al. (2007). Waddlia chondrophila, a potential agent of human fetal death. Emerg Infect Dis 13:1239–43.
- Bavoil P, Kaltenboeck B, Greub G. (2013). In Chlamydia veritas. Pathog Dis 67:89–90.
- Bavoil P, Ohlin A, Schachter J. (1984). Role of disulfide bonding in outer membrane structure and permeability in Chlamydia trachomatis. Infect Immun 44:479–85.
- Beatty WL, Morrison RP, Byrne GI. (1994). Persistent Chlamydiae: from cell culture to a paradigm for chlamydial pathogenesis. Microbiol Rev 58:686–99.
- Bertelli C, Aeby S, Chassot B, et al. (2015). Sequencing and characterizing the genome of Estrella lausannensis as an undergraduate project: training students and biological insights. Front Microbiol 6:101.
- Bertelli C, Collyn F, Croxatto A, et al. (2010). The Waddlia genome: a window into chlamydial biology. PLoS One 5:e10890.
- Betts-Hampikian HJ, Fields KA. (2011). Disulfide bonding within components of the Chlamydia type III secretion apparatus correlates with development. J Bacteriol 193:6950–9.
- Birkelund S, Morgan-Fisher M, Timmerman E, et al. (2009). Analysis of proteins in Chlamydia trachomatis L2 outer membrane complex, COMC. FEMS Immunol Med Microbiol 55:187–95.
- Blasi F, Tarsia P, Aliberti S. (2009). Chlamydophila pneumoniae. Clin Microbiol Infect 15:29–35.
- Bonner CA, Byrne GI, Jensen RA. (2014). Chlamydia exploit the mammalian tryptophan-depletion defense strategy as a counter-defensive cue to trigger a survival state of persistence. Front Cell Infect Microbiol 4:17.
- Brinkman FSL, Blanchard JL, Cherkasov A, et al. (2002). Evidence that plant-like genes in Chlamydia species reflect an ancestral relationship between Chlamydiaceae, cyanobacteria, and the chloroplast. Genome Res 12:1159–67.
- Brunham RC, Rey-Ladino J. (2005). Immunology of Chlamydia infection: implications for a Chlamydia trachomatis vaccine. Nat Rev Immunol 5:149–61.
- Budd A, Devos DP. (2012). Evaluating the evolutionary origins of unexpected character distributions within the bacterial Planctomycetes-Verrucomicrobia-Chlamydiae Superphylum. Front Microbiol 3:401.
- Caldwell HD, Kromhout J, Schachter J. (1981). Purification and partial characterization of the major outer membrane protein of Chlamydia trachomatis. Infect Immun 31:1161–76.
- Carlson JH, Whitmire WM, Crane DD, et al. (2008). The Chlamydia trachomatis plasmid is a transcriptional regulator of chromosomal genes and a virulence factor. Infect Immun 76:2273–83.
- Carlson JH, Wood H, Roshick C, et al. (2006). In vivo and in vitro studies of Chlamydia trachomatis TrpR:DNA interactions. Mol Microbiol 59:1678–91.
- Casson N, Greub G. (2006). Resistance of different Chlamydia-like organisms to quinolones and mutations in the quinoline resistance-determining region of the DNA gyrase A- and topoisomerase-encoding genes. Int J Antimicrob Agents 27:541–4.
- Casson N, Michel R, Müller KD, et al. (2008a). Protochlamydia naegleriophila as etiologic agent of pneumonia. Emerging Infect Dis 14:168–72. doi:10.3201/eid1401.070980.
- Casson N, Posfay-Barbe KM, Gervaix A, Greub G. (2008b). New diagnostic real-time PCR for specific detection of Parachlamydia acanthamoebae DNA in clinical samples. J Clin Microbiol 46:1491–3.
- Collingro A, Tischler P, Weinmaier T, et al. (2011). Unity in variety-the pan-genome of the Chlamydiae. Mol Biol Evol 28:3253–70.
- Corsaro D, Greub G. (2006). Pathogenic potential of novel Chlamydiae and diagnostic approaches to infections due to these obligate intracellular bacteria. Clin Microbiol Rev 19:283–97.
- Corsaro D, Venditti D. (2009). Detection of Chlamydiae from freshwater environments by PCR, amoeba coculture and mixed coculture. Res Microbiol 160:547–52.
- Corsaro D, Venditti D, Valassina M. (2002). New chlamydial lineages from freshwater samples. Microbiology (Reading, Engl.) 148:343–4.
- Croxatto A, Greub G. (2010). Early intracellular trafficking of Waddlia chondrophila in human macrophages. Microbiology (Reading, Engl.) 156:340–55.
- Croxatto A, Rieille N, Kernif T, et al. (2014). Presence of Chlamydiales DNA in ticks and fleas suggests that ticks are carriers of Chlamydiae. Ticks Tick-Borne Dis 5:359–65.
- de Barsy M, Bottinelli L, Greub G. (2014). Antibiotic susceptibility of Estrella lausannensis, a potential emerging pathogen. Microbes Infect 16:746–54.
- Derré I. (2015). Chlamydiae interaction with the endoplasmic reticulum: contact, function and consequences. Cell Microbiol 17:959–66.
- Derré I, Swiss R, Agaisse H. (2011). The lipid transfer protein CERT interacts with the Chlamydia inclusion protein IncD and participates to ER-Chlamydia inclusion membrane contact sites. PLoS Pathog 7:e1002092.
- Donati M, Cremonini E, Di Francesco A, et al. (2015). Prevalence of Simkania negevensis in chlorinated water from spa swimming pools and domestic supplies. J Appl Microbiol 118:1076–82.
- Donati M, Di Francesco A, Di Paolo M, et al. (2011). Activity of cathelicidin peptides against Simkania negevensis. Int J Pept 2011:708710.
- Donati M, Fiani N, Di Francesco A, et al. (2013). IgG and IgA response to Simkania negevensis in sera of patients with respiratory and gastrointestinal symptoms. New Microbiol 36:303–6.
- Dowell SF, Peeling RW, Boman J, et al. (2001). Standardizing Chlamydia pneumoniae assays: recommendations from the Centers for Disease Control and Prevention (USA) and the Laboratory Centre for Disease Control (Canada). Clin Infect Dis 33:492–503.
- Dumke R, Schnee C, Pletz MW, et al. (2015). Mycoplasma pneumoniae and Chlamydia spp. infection in community-acquired pneumonia, Germany, 2011–2012. Emerg Infect Dis 21:426–34.
- Efron B, Halloran E, Holmes S. (1996). Bootstrap confidence levels for phylogenetic trees. Proc Natl Acad Sci USA 93:13429–34.
- Everett KD, Andersen AA. (1997). The ribosomal intergenic spacer and domain I of the 23S rRNA gene are phylogenetic markers for Chlamydia spp. Int J Syst Bacteriol 47:461–73.
- Everett KD, Bush RM, Andersen AA. (1999a). Emended description of the order Chlamydiales, proposal of Parachlamydiaceae fam. nov. and Simkaniaceae fam. nov., each containing one monotypic genus, revised taxonomy of the family Chlamydiaceae, including a new genus and five new species, and standards for the identification of organisms. Int J Syst Bacteriol 49:415–40.
- Everett KDE, Thao M, Horn M, et al. (2005). Novel Chlamydiae in whiteflies and scale insects: endosymbionts “Candidatus Fritschea bemisiae” strain Falk and “Candidatus Fritschea eriococci” strain Elm. Int J Syst Evol Microbiol 55:1581–7.
- Everett KD, Hatch TP. (1995). Architecture of the cell envelope of Chlamydia psittaci 6BC. J Bacteriol 177:877–82.
- Everett KD, Kahane S, Bush RM, Friedman MG. (1999b). An unspliced group I intron in 23S rRNA links Chlamydiales, chloroplasts, and mitochondria. J Bacteriol 181:4734–40.
- Fan T, Lu H, Hu H, et al. (1998). Inhibition of apoptosis in Chlamydia-infected cells: blockade of mitochondrial cytochrome c release and caspase activation. J Exp Med 187:487–96.
- Fasoli L, Paldanius M, Don M, et al. (2008). Simkania negevensis in community-acquired pneumonia in Italian children. Scand J Infect Dis 40:269–72.
- Fehr A, Walther E, Schmidt-Posthaus H, et al. (2013). Candidatus Syngnamydia venezia, a novel member of the phylum Chlamydiae from the broad nosed pipefish, Syngnathus typhle. PLoS One 8:e70853.
- Forhan SE, Gottlieb SL, Sternberg MR, et al. (2009). Prevalence of sexually transmitted infections among female adolescents aged 14 to 19 in the United States. Pediatrics 124:1505–12.
- Frandi A, Jacquier N, Théraulaz L, et al. (2014). FtsZ-independent septal recruitment and function of cell wall remodelling enzymes in chlamydial pathogens. Nat Commun 5:4200. doi:10.1038/ncomms5200.
- Friedman MG, Galil A, Greenberg S, Kahane S. (1999). Seroprevalence of IgG antibodies to the chlamydia-like microorganism “Simkania Z” by ELISA. Epidemiol Infect 122:117–23.
- Friedman MG, Kahane S, Dvoskin B, Hartley JW. (2006). Detection of Simkania negevensis by culture, PCR, and serology in respiratory tract infection in Cornwall, UK. J Clin Pathol 59:331–3.
- Gimenes F, Souza RP, Bento JC, et al. (2014). Male infertility: a public health issue caused by sexually transmitted pathogens. Nat Rev Urol 11:672–87.
- Gottlieb SL, Xu F, Brunham RC. (2013). Screening and treating Chlamydia trachomatis genital infection to prevent pelvic inflammatory disease: interpretation of findings from randomized controlled trials. Sex Transm Dis 40:97–102.
- Goy G, Croxatto A, Greub G. (2008). Waddlia chondrophila enters and multiplies within human macrophages. Microbes Infect Inst Pasteur 10:556–62.
- Goy G, Croxatto A, Posfay-Barbe KM, et al. (2009). Development of a real-time PCR for the specific detection of Waddlia chondrophila in clinical samples. Eur J Clin Microbiol Infect Dis 28:1483–6.
- Goy G, Greub G. (2009). Antibiotic susceptibility of Waddlia chondrophila in Acanthamoeba castellanii amoebae. Antimicrob Agents Chemother 53:2663–6.
- Greenberg D, Banerji A, Friedman MG, et al. (2003). High rate of Simkania negevensis among Canadian inuit infants hospitalized with lower respiratory tract infections. Scand J Infect Dis 35:506–8.
- Greub G. (2013). Pathogenesis and cell corruption by intracellular bacteria. Microbes Infect Inst Pasteur 15:969–70.
- Greub G. (2010). International Committee on Systematics of Prokaryotes. Subcommittee on the taxonomy of the Chlamydiae: minutes of the inaugural closed meeting, 21 March 2009, Little Rock, AR, USA. Int J Syst Evol Microbiol 60:2691–3.
- Greub G. (2009). Parachlamydia acanthamoebae, an emerging agent of pneumonia. Clin Microbiol Infect 15:18–28.
- Greub G, Boyadjiev I, La Scola B, et al. (2003a). Serological hint suggesting that Parachlamydiaceae are agents of pneumonia in polytraumatized intensive care patients. Ann N Y Acad Sci 990:311–19.
- Greub G, Collyn F, Guy L, Roten CA. (2004a). A genomic island present along the bacterial chromosome of the Parachlamydiaceae UWE25, an obligate amoebal endosymbiont, encodes a potentially functional F-like conjugative DNA transfer system. BMC Microbiol 4:48.
- Greub G, Kebbi-Beghdadi C, Bertelli C, et al. (2009). High throughput sequencing and proteomics to identify immunogenic proteins of a new pathogen: the dirty genome approach. PLoS One 4:e8423.
- Greub G, La Scola B, Raoult D. (2004b). Amoebae-resisting bacteria isolated from human nasal swabs by amoebal coculture. Emerg Infect Dis 10:470–7.
- Greub G, La Scola B, Raoult D. (2003b). Parachlamydia acanthamoeba is endosymbiotic or lytic for Acanthamoeba polyphaga depending on the incubation temperature. Ann N Y Acad Sci 990:628–34.
- Greub G, Mege JL, Gorvel JP, et al. (2005). Intracellular trafficking of Parachlamydia acanthamoebae. Cell Microbiol 7:581–9.
- Greub G, Mege JL, Raoult D. (2003c). Parachlamydia acanthamoebae enters and multiplies within human macrophages and induces their apoptosis [corrected]. Infect Immun 71:5979–85.
- Greub G, Raoult D. (2004). Microorganisms resistant to free-living amoebae. Clin Microbiol Rev 17:413–33.
- Greub G, Raoult D. (2002a). Parachlamydiaceae: potential emerging pathogens. Emerg Infect Dis 8:625–30.
- Greub G, Raoult D. (2002b). Crescent bodies of Parachlamydia acanthamoebae and its life cycle within Acanthamoeba polyphaga: an electron micrograph study. Appl Environ Microbiol 68:3076–84.
- Hammerschlag MR, Kohlhoff SA. (2012). Treatment of chlamydial infections. Expert Opin Pharmacother 13:545–52.
- Heiskanen-Kosma T, Paldanius M, Korppi M. (2008). Simkania negevensis may be a true cause of community acquired pneumonia in children. Scand J Infect Dis 40:127–30.
- Herweg JA, Pons V, Becher D, et al. (2016). Proteomic analysis of the Simkania-containing vacuole: the central role of retrograde transport. Mol Microbiol 99:151–71.
- Heuer D, Rejman Lipinski A, Machuy N, et al. (2009). Chlamydia causes fragmentation of the Golgi compartment to ensure reproduction. Nature 457:731–5.
- Horn M. (2008). Chlamydiae as symbionts in eukaryotes. Annu Rev Microbiol 62:113–31.
- Horn M, Collingro A, Schmitz-Esser S, et al. (2004). Illuminating the evolutionary history of Chlamydiae. Science 304:728–30.
- Hovis KM, Mojica S, McDermott JE, et al. (2013). Genus-optimized strategy for the identification of chlamydial type III secretion substrates. Pathog Dis 69:213–22.
- Hsia RC, Pannekoek Y, Ingerowski E, Bavoil PM. (1997). Type III secretion genes identify a putative virulence locus of Chlamydia. Mol Microbiol 25:351–9.
- Husain S, Kahane S, Friedman MG, et al. (2007). Simkania negevensis in bronchoalveolar lavage of lung transplant recipients: a possible association with acute rejection. Transplantation 83:138–43.
- Hybiske K, Stephens RS. (2007). Mechanisms of host cell exit by the intracellular bacterium Chlamydia. Proc Natl Acad Sci USA 104:11430–5.
- Ibana JA, Belland RJ, Zea AH, et al. (2011). Inhibition of indoleamine 2,3-dioxygenase activity by levo-1-methyl tryptophan blocks gamma interferon-induced Chlamydia trachomatis persistence in human epithelial cells. Infect Immun 79:4425–37.
- Israelsson O. (2007). Chlamydial symbionts in the enigmatic Xenoturbella (Deuterostomia). J Invertebr Pathol 96:213–20.
- Jacquier N, Aeby S, Lienard J, Greub G. (2013). Discovery of new intracellular pathogens by amoebal coculture and amoebal enrichment approaches. J Vis Exp JoVE e51055.
- Jacquier N, Frandi A, Pillonel T, et al. (2014). Cell wall precursors are required to organize the chlamydial division septum. Nat Commun 5:3578.
- Jacquier N, Viollier P, Greub G. (2015). The role of peptidoglycans in chlamydial cell division: towards resolving the chlamydial anomaly. FEMS Microbiol Rev 39:262–75.
- Johnsen S, Birkebaek N, Andersen PL, et al. (2005). Indirect immunofluorescence and real time PCR for detection of Simkania negevensis infection in Danish adults with persistent cough and in healthy controls. Scand J Infect Dis 37:251–5.
- Kahane S, Dvoskin B, Friedman MG. (2008). The role of monocyte/macrophages as vehicles of dissemination of Simkania negevensis: an in vitro simulation model. FEMS Immunol Med Microbiol 52:219–27.
- Kahane S, Dvoskin B, Mathias M, Friedman MG. (2001). Infection of Acanthamoeba polyphaga with Simkania negevensis and S. negevensis survival within amoebal cysts. Appl Environ Microbiol 67:4789–95.
- Kahane S, Everett KD, Kimmel N, Friedman MG. (1999). Simkania negevensis strain ZT: growth, antigenic and genome characteristics. Int J Syst Bacteriol 49:815–20.
- Kahane S, Fruchter D, Dvoskin B, Friedman MG. (2007a). Versatility of Simkania negevensis infection in vitro and induction of host cell inflammatory cytokine response. J Infect 55:e13–21.
- Kahane S, Gonen R, Sayada C, et al. (1993). Description and partial characterization of a new Chlamydia-like microorganism. FEMS Microbiol Lett 109:329–33.
- Kahane S, Greenberg D, Friedman MG, et al. (1998). High prevalence of “‘Simkania Z’ a novel Chlamydia-like bacterium, in infants with acute bronchiolitis”. J Infect Dis 177:1425–9.
- Kahane S, Greenberg D, Newman N, et al. (2007b). Domestic water supplies as a possible source of infection with Simkania. J Infect 54:75–81.
- Kahane S, Kimmel N, Friedman MG. (2002). The growth cycle of Simkania negevensis. Microbiology (Reading, Engl.) 148:735–42.
- Kahane S, Metzer E, Friedman MG. (1995). Evidence that the novel microorganism “Z” may belong to a new genus in the family Chlamydiaceae. FEMS Microbiol Lett 126:203–7.
- Kahane S, Platzner N, Dvoskin B, et al. (2004). Evidence for the presence of Simkania negevensis in drinking water and in reclaimed wastewater in Israel. Appl Environ Microbiol 70:3346–51.
- Kari L, Whitmire WM, Olivares-Zavaleta N, et al. (2011). A live-attenuated chlamydial vaccine protects against trachoma in nonhuman primates. J Exp Med 208:2217–23.
- Karunakaran K, Mehlitz A, Rudel T. (2011). Evolutionary conservation of infection-induced cell death inhibition among Chlamydiales. PLoS One 6:e22528.
- Kearse M, Moir R, Wilson A, et al. (2012). Geneious basic: an integrated and extendable desktop software platform for the organization and analysis of sequence data. Bioinform Oxf Engl 28:1647–9.
- Kebbi-Beghdadi C, Cisse O, Greub G. (2011). Permissivity of Vero cells, human pneumocytes and human endometrial cells to Waddlia chondrophila. Microbes Infect Inst Pasteur 13:566–74.
- Kebbi-Beghdadi C, Domröse A, Becker E, et al. (2015). OmpA family proteins and Pmp-like autotransporter: new adhesins of Waddlia chondrophila. Pathog Dis 73:ftv035.
- Knab S, Mushak TM, Schmitz-Esser S, et al. (2011). Nucleotide parasitism by Simkania negevensis (Chlamydiae). J Bacteriol 193:225–35.
- Knittler MR, Sachse K. (2015). Chlamydia psittaci: update on an underestimated zoonotic agent. Pathog Dis 73:1–15.
- Korppi M, Paldanius M, Hyvärinen A, Nevalainen A. (2006). Simkania negevensis and newly diagnosed asthma: a case-control study in 1- to 6-year-old children. Respirol Carlton Vic 11:80–3.
- Kostanjsek R, Strus J, Drobne D, Avgustin G. (2004). “Candidatus Rhabdochlamydia porcellionis”, an intracellular bacterium from the hepatopancreas of the terrestrial isopod Porcellio scaber (Crustacea: Isopoda). Int J Syst Evol Microbiol 54:543–9.
- Kropf P, Baud D, Marshall SE, et al. (2007). Arginase activity mediates reversible T cell hyporesponsiveness in human pregnancy. Eur J Immunol 37:935–45.
- Kumar S, Kohlhoff SA, Gelling M, et al. (2005). Infection with Simkania negevensis in Brooklyn, New York. Pediatr Infect Dis J 24:989–92.
- Kuo CC, Jackson LA, Campbell LA, Grayston JT. (1995). Chlamydia pneumoniae (TWAR). Clin Microbiol Rev 8:451–61.
- Lagkouvardos I, Weinmaier T, Lauro FM, et al. (2014). Integrating metagenomic and amplicon databases to resolve the phylogenetic and ecological diversity of the Chlamydiae. ISME J 8:115–25.
- Lamoth F, Greub G. (2010). Amoebal pathogens as emerging causal agents of pneumonia. FEMS Microbiol Rev 34:260–80.
- Lieberman D, Dvoskin B, Lieberman DV, et al. (2002). Serological evidence of acute infection with the Chlamydia-like microorganism Simkania negevensis (Z) in acute exacerbation of chronic obstructive pulmonary disease. Eur J Clin Microbiol Infect Dis 21:307–9.
- Lieberman D, Kahane S, Lieberman D, Friedman MG. (1997). Pneumonia with serological evidence of acute infection with the Chlamydia-like microorganism “Z”. Am J Respir Crit Care Med 156:578–82.
- Liechti GW, Kuru E, Hall E, et al. (2014). A new metabolic cell-wall labelling method reveals peptidoglycan in Chlamydia trachomatis. Nature 506:507–10.
- Lienard J, Croxatto A, Aeby S, et al. (2011a). Development of a new chlamydiales-specific real-time PCR and its application to respiratory clinical samples. J Clin Microbiol 49:2637–42.
- Lienard J, Croxatto A, Gervaix A, et al. (2014). Undressing of Waddlia chondrophila to enrich its outer membrane proteins to develop a new species-specific ELISA. New Microbes New Infect 2:13–24.
- Lienard J, Croxatto A, Prod’hom G, Greub G. (2011b). Estrella lausannensis, a new star in the Chlamydiales order. Microbes Infect Inst Pasteur 13:1232–41.
- Liu Y, Huang Y, Yang Z, et al. (2014). Plasmid-encoded Pgp3 is a major virulence factor for Chlamydia muridarum to induce hydrosalpinx in mice. Infect Immun 82:5327–35.
- Li Z, Chen D, Zhong Y, et al. (2008). The chlamydial plasmid-encoded protein pgp3 is secreted into the cytosol of Chlamydia-infected cells. Infect Immun 76:3415–28.
- Lundemose AG, Kay JE, Pearce JH. (1993a). Chlamydia trachomatis Mip-like protein has peptidyl-prolyl cis/trans isomerase activity that is inhibited by FK506 and rapamycin and is implicated in initiation of chlamydial infection. Mol Microbiol 7:777–83.
- Lundemose AG, Rouch DA, Penn CW, Pearce JH. (1993b). The Chlamydia trachomatis Mip-like protein is a lipoprotein. J Bacteriol 175:3669–71.
- Matsumoto A, Manire GP. (1970). Electron microscopic observations on the effects of penicillin on the morphology of Chlamydia psittaci. J Bacteriol 101:278–85.
- Mehlitz A, Karunakaran K, Herweg JA, et al. (2014). The chlamydial organism Simkania negevensis forms ER vacuole contact sites and inhibits ER-stress. Cell Microbiol 16:1224–43.
- Miyairi I, Mahdi OS, Ouellette SP, et al. (2006). Different growth rates of Chlamydia trachomatis biovars reflect pathotype. J Infect Dis 194:350–7.
- Nascimento-Carvalho CM, Cardoso MRA, Paldanius M, et al. (2009). Simkania negevensis infection among Brazilian children hospitalized with community-acquired pneumonia. J Infect 58:250–3.
- Nesbø CL, Doolittle WF. (2003). Active self-splicing group I introns in 23S rRNA genes of hyperthermophilic bacteria, derived from introns in eukaryotic organelles. Proc Natl Acad Sci USA 100:10806–11.
- Niemi S, Greub G, Puolakkainen M. (2011). Chlamydia-related bacteria in respiratory samples in Finland. Microbes Infect Inst Pasteur 13:824–7.
- Nylund S, Steigen A, Karlsbakk E, et al. (2015). Characterization of “Candidatus Syngnamydia salmonis” (Chlamydiales, Simkaniaceae). A bacterium associated with epitheliocystis in Atlantic Salmon (Salmo salar L.). Arch Microbiol 197:17–25.
- O’Connell CM, Ingalls RR, Andrews CW, et al. (2007). Plasmid-deficient Chlamydia muridarum fail to induce immune pathology and protect against oviduct disease. J Immunol Baltim Md 1950 179:4027–34.
- Ogata H, La Scola B, Audic S, et al. (2006). Genome sequence of Rickettsia bellii illuminates the role of amoebae in gene exchanges between intracellular pathogens. PLoS Genet 2:e76.
- Omsland A, Sixt BS, Horn M, Hackstadt T. (2014). Chlamydial metabolism revisited: interspecies metabolic variability and developmental stage-specific physiologic activities. FEMS Microbiol Rev 38:779–801.
- Packiam M, Weinrick B, Jacobs WR, Maurelli AT. (2015). Structural characterization of muropeptides from Chlamydia trachomatis peptidoglycan by mass spectrometry resolves “chlamydial anomaly”. Proc Natl Acad Sci USA 112:11660–5.
- Pellati D, Mylonakis I, Bertoloni G, et al. (2008). Genital tract infections and infertility. Eur J Obstet Gynecol Reprod Biol 140:3–11.
- Pérez LM, Codony F, Ríos K, et al. (2011). Prevalence study of Simkania negevensis in cooling towers in Spain. J Water Health 9:312–16.
- Pérez LM, Codony F, Ríos K, et al. (2012). Searching Simkania negevensis in environmental waters. Folia Microbiol (Praha) 57:11–14.
- Pilhofer M, Aistleitner K, Biboy J, et al. (2013). Discovery of chlamydial peptidoglycan reveals bacteria with murein sacculi but without FtsZ. Nat Commun 4:2856. doi:10.1038/ncomms3856..
- Pilhofer M, Aistleitner K, Ladinsky MS, et al. (2014). Architecture and host interface of environmental Chlamydiae revealed by electron cryotomography. Environ Microbiol 16:417–29.
- Pillonel T, Bertelli C, Salamin N, Greub G. (2015). Taxogenomics of the Chlamydiales. Int J Syst Evol Microbiol 65:1381–93.
- Pilloux L, Aeby S, Gaümann R, et al. (2015). The high prevalence and diversity of Chlamydiales DNA within Ixodes ricinus ticks suggest a role for ticks as reservoirs and vectors of Chlamydia-related bacteria. Appl Environ Microbiol 81:8177–82.
- Pletz MW, Rohde G, Schütte H, et al. (2011). Epidemiology and aetiology of community-acquired pneumonia (CAP). Dtsch Med Wochenschr 1946 136:775–80.
- Porcella SF, Carlson JH, Sturdevant DE, et al. (2015). Transcriptional profiling of human epithelial cells infected with plasmid-bearing and plasmid-deficient Chlamydia trachomatis. Infect Immun 83:534–43.
- Principi N, Esposito S. (2001). Emerging role of Mycoplasma pneumoniae and Chlamydia pneumoniae in paediatric respiratory tract infections. Lancet Infect Dis 1:334–44.
- Raulston JE. (1997). Response of Chlamydia trachomatis serovar E to iron restriction in vitro and evidence for iron-regulated chlamydial proteins. Infect Immun 65:4539–47.
- Rottenberg ME, Gigliotti-Rothfuchs A, Wigzell H. (2002). The role of IFN-gamma in the outcome of chlamydial infection. Curr Opin Immunol 14:444–51.
- Rusconi B, Greub G. (2013). Discovery of catalases in members of the Chlamydiales order. J Bacteriol 195:3543–51.
- Rusconi B, Lienard J, Aeby S, et al. (2013). Crescent and star shapes of members of the Chlamydiales order: impact of fixative methods. Antonie Van Leeuwenhoek 104:521–32.
- Sagaram US, DeAngelis KM, Trivedi P, et al. (2009). Bacterial diversity analysis of Huanglongbing pathogen-infected citrus, using PhyloChip arrays and 16S rRNA gene clone library sequencing. Appl Environ Microbiol 75:1566–74.
- Saitou N, Nei M. (1987). The neighbor-joining method: a new method for reconstructing phylogenetic trees. Mol Biol Evol 4:406–25.
- Santelli CM, Orcutt BN, Banning E, et al. (2008). Abundance and diversity of microbial life in ocean crust. Nature 453:653–6.
- Sauvadet AL, Le Panse S, Roussel EG, et al. 2011. Tripartite interactions between Cirratulidae (Polychaeta), Durchoniella (Ciliophora, Astomatia) and Bacteria: A Russian Doll complex in anoxic coastal environments.
- Schachter J. (1986). Chlamydia psittaci – ‘reemergence’ of a forgotten pathogen. N Engl J Med 315:189–91.
- Senn L, Jaton K, Fitting JW, Greub G. (2011). Does respiratory infection due to Chlamydia pneumoniae still exist? Clin Infect Dis 53:847–8.
- Sixt BS, Hiess B, König L, Horn M. (2012). Lack of effective anti-apoptotic activities restricts growth of Parachlamydiaceae in insect cells. PLoS One 7:e29565.
- Soldati G, Lu ZH, Vaughan L, et al. (2004). Detection of mycobacteria and Chlamydiae in granulomatous inflammation of reptiles: a retrospective study. Vet Pathol 41:388–97.
- Song L, Carlson JH, Whitmire WM, et al. (2013). Chlamydia trachomatis plasmid-encoded Pgp4 is a transcriptional regulator of virulence-associated genes. Infect Immun 81:636–44.
- Steinhoff D, Lode H, Ruckdeschel G, et al. (1996). Chlamydia pneumoniae as a cause of community-acquired pneumonia in hospitalized patients in Berlin. Clin Infect Dis 22:958–64.
- Stephens RS, Kalman S, Lammel C, et al. (1998). Genome sequence of an obligate intracellular pathogen of humans: Chlamydia trachomatis. Science 282:754–9.
- Stride MC, Polkinghorne A, Miller TL, et al. (2013). Molecular characterization of “Candidatus Parilichlamydia carangidicola,” a novel Chlamydia-like epitheliocystis agent in yellowtail kingfish, Seriola lalandi (Valenciennes), and the proposal of a new family, “Candidatus Parilichlamydiaceae” fam. nov. (order Chlamydiales). Appl Environ Microbiol 79:1590–7.
- Sun G, Pal S, Sarcon AK, et al. (2007). Structural and functional analyses of the major outer membrane protein of Chlamydia trachomatis. J Bacteriol 189:6222–35.
- Tamura K. (1992). Estimation of the number of nucleotide substitutions when there are strong transition–transversion and G + C-content biases. Mol Biol Evol 9:678–87.
- Taylor MW, Feng GS. (1991). Relationship between interferon-gamma, indoleamine 2,3-dioxygenase, and tryptophan catabolism. FASEB J 5:2516–22.
- Thao ML, Baumann L, Hess JM, et al. (2003). Phylogenetic evidence for two new insect-associated Chlamydia of the family Simkaniaceae. Curr Microbiol 47:46–50.
- Thomas V, Casson N, Greub G. (2006). Criblamydia sequanensis, a new intracellular Chlamydiales isolated from Seine river water using amoebal co-culture. Environ Microbiol 8:2125–35.
- Vouga M, Diabi H, Boulos A, et al. (2015). Antibiotic susceptibility of Neochlamydia hartmanellae and Parachlamydia acanthamoebae in amoebae. Microbes Infect Inst Pasteur 17:761–5.
- Walker DH, Ismail N. (2008). Emerging and re-emerging rickettsioses: endothelial cell infection and early disease events. Nat Rev Microbiol 6:375–86.
- Wellinghausen N, Straube E, Freidank H, et al. (2006). Low prevalence of Chlamydia pneumoniae in adults with community-acquired pneumonia. Int J Med Microbiol 296:485–91.
- Xue X, Li SJ, Ahmed MZ, et al. (2012). Inactivation of Wolbachia reveals its biological roles in whitefly host. PLoS One 7:e48148.
- Yamaguchi T, Yamazaki T, Inoue M, et al. (2005). Prevalence of antibodies against Simkania negevensis in a healthy Japanese population determined by the microimmunofluorescence test. FEMS Immunol Med Microbiol 43:21–7.