Abstract
Thiolated polyacrylates were shown to be permeation enhancers with notable potential. The aim of this study was to evaluate the permeation enhancing properties of a thiolated polycarbophil/glutathione (PCP-Cys/GSH) system for oral drug application in comparison to a well-established permeation enhancer, namely sodium caprate. In vitro permeation studies were conducted in Ussing-type chambers with sodium fluoresceine (NaFlu) and fluoresceine isothiocyanate labeled dextran (molecular mass 4 kDa; FD4) as model compounds. Bioavailability studies were carried out in Sprague Dawley rats with various formulations. Moreover, cytotoxic effects of both permeation enhancers were compared. Permeation enhancement ratios of 1% sodium caprate were found to be 3.0 (FD4) and 2.3 (NaFlu), whereas 1% PCP-Cys/0.5% GSH displayed enhancement ratios of 2.4 and 2.2. Both excipients performed at a similar level in vivo. Sodium caprate solutions increased oral bioavailability 2.2-fold (FD4) and 2.3-fold (NaFlu), while PCP-Cys hydrogels led to a 3.2-fold and 2.2-fold enhancement. Cell viability experiments revealed a significantly higher tolerance of Caco-2 cells towards 0.5% PCP-Cys (81% survival) compared to 0.5% sodium caprate (5%). As PCP-Cys is not absorbed from mucosal membranes due to its comparatively high molecular mass, systemic side-effects can be excluded. In conclusion, both systems displayed a similar potency for permeation enhancement of hydrophilic compounds. However, PCP-Cys seems to be less harmful to cultured cells.
Introduction
Modern medicine includes a vast number of therapeutic agents that have to be administered parenterally due to their null or strongly limited bioavailability after oral administration. Commonly, these agents are hydrophilic compounds of relatively high molecular mass or strongly charged compounds. According to these physiochemical properties the paracellular pathway appears to be the most favorable route across mucosal epithelia (CitationHayashi et al., 1997). Unfortunately, drug absorption across this pathway is hampered by tight junctions between epithelial cells which reduce paracellular permeability (CitationDiamond, 1977).
Over two decades a wide range of studies in cell culture systems as well as rodents have shown that medium-chain fatty acids enhance the transport of drugs across mucosal membranes (CitationAungst, 2000). Among these, sodium caprate (C10) seems to be the most investigated and most effective permeation enhancer. Dilatation of tight junctions by contraction of perijunctional actin rings via intracellular Ca2+-activation is supposed to cause the permeation enhancing effect of C10 (CitationAnderberg et al., 1993). Nowadays, C10 is widely accepted and has been investigated as an adjuvant enhancing drug absorption on various routes besides the oral one such as the nasal (CitationChavanpatil & Vavia, 2005) or transdermal (CitationIbrahim & Li, 2010) route. Moreover, it is already used clinically in Doctacillin™ suppositories. Nevertheless, several in vitro cytotoxicity studies revealed that C10 displays a notable cytotoxicity (CitationSakai et al., 1998).
More recently, thiolated poly(acrylic acid) conjugates and polycarbophil-cysteine (PCP-Cys), in particular, were shown to elicit strongly improved permeation of hydrophilic compounds compared to the corresponding unmodified polymers (CitationClausen & Bernkop-Schnurch, 2000). These polymeric compounds are capable of increasing local concentrations of GSH by reducing oxidized glutathione (GSSG). GSH in turn is able to inhibit protein tyrosin phosphatase (PTP) by disulfide bond formation with the active site of the enzyme (CitationClausen et al., 2002). PTP is involved in the closure of tight junctions by dephosphorylation of occludin. Thus, PTP inhibition results in prolonged opening of tight junctions and in disruption of cell–cell adhesions which are accompanied by an enhanced permeability for hydrophilic compounds such as peptides and proteins or heparin derivatives (CitationWachtel et al., 1999). This effect can be improved by co-administration of GSH. In comparison to the well-established permeation enhancer C10, thiolated poly(acrylic acid) conjugates showed negligible cytotoxic effects within in vitro studies (CitationVetter et al., 2010). Moreover, polyacrylates of a sufficiently high molecular mass are not absorbed from mucosal membranes according to the literature (CitationRiley et al., 2001). Hence, systemic toxic effects after mucosal administration can be excluded.
In contrast to previous studies, it was the objective of this study to evaluate the potential of PCP-Cys as a permeation enhancer for two different model compounds in the presence of GSH compared to the widely accepted excipient C10 instead of just unmodified polymers and buffer controls. For this purpose, in vitro permeation studies on freshly excised rat intestine, cytotoxicity studies on Caco-2 cells, as well as in vivo bioavailability studies in Sprague-Dawley rats were performed. Sodium fluoresceine (NaFlu) and fluoresceine isothiocyanate-dextran (molecular mass 4 kDa; FD4) were utilized as model compounds for highly hydrophilic drugs of different molecular masses.
Materials and methods
Materials
Noveon® AA-1 USP Polycarbophil (PCP) was obtained as a kind donation from Lubrizol (Hamburg, Germany). 1-Ethyl-3-(dimethylaminopropyl) carbodiimide (≥ 98%) (EDAC) was purchased from Acros Organics (Geel, Belgium). All other chemicals were of analytical grade and were purchased from Sigma Aldrich (Vienna, Austria).
Polymer synthesis
The PCP-Cys conjugate (PCP-Cys) was synthesized as described previously by our research group (CitationBernkop-Schnurch et al., 1999). In brief, cysteine was covalently attached to PCP via amide bond formation between the primary amino group of L-cysteine and carboxylic groups of PCP. One gram of PCP was hydrated in 250 mL of demineralized water and neutralized with 1 M NaOH before pre-dissolved EDAC (1 g in 5 mL of water, pH 5) was added in order to activate the carboxylic acid moieties. After 15 min, pre-dissolved L-cysteine hydrochloride (1 g in 5 mL of water, pH 5) was added and pH of the reaction mixture was adjusted to 5. The reaction mixture was stirred for 3 h and pH was re-adjusted to 5, regularly. The resulting conjugate was isolated by dialysis against 0.2 mM HCl twice, twice against 0.2 mM HCl containing 1% NaCl and again twice against 0.2 mM HCl. Dialysis was conducted at 10°C and in the dark in order to minimize oxidation of thiol groups. Finally, pH was adjusted to 4 and the polymer was frozen at 70°C before being lyophilized at −75°C condenser temperature and 4 × 10−4 mbar (Benchtop 2K, VirTis, Gardiner, NY). The polymer conjugate was stored at 4°C until further use.
PCP-Cys conjugate characterization
Free thiol groups on the polymer backbone were quantified via reductive cleavage of Ellman’s reagent (5,5′-dithiobis-(2-nitrobenzoic acid) or DTNB) to 2-nitro-5-thiobenzoate at pH 8 (CitationBernkop-Schnurch et al., 1999). For this purpose, 1 mg polymer was hydrated in 500 µL of 0.5 M phosphate buffer before 500 µL of a 0.03% (m/v) solution of DTNB in the same buffer was added. Thiol concentrations were derived from a calibration curve which was obtained from solutions of different L-cysteine concentrations.
The total amount of attached L-cysteine was quantified with Ellman’s reagent after exhaustive reduction with sodium borohydride (CitationHabeeb, 1973).
In vitro permeation studies across freshly excised rat intestinal mucosa
Right after sacrificing a rat (Sprague-Dawley rat, 300–400 g body weight), the small intestine (lower jejunum and ileum) was excised and pieces of 3–4 cm2 were mounted in Ussing-type chambers without stripping the underlying muscle layer. Ussing-type chambers with a volume of 1 mL in each chamber and a permeation area of 0.64 cm2 were utilized. Two different media were prepared for the basolateral and the apical chamber. For the apical chamber a buffer comprising 138 mM NaCl, 5 mM KCl, 10 mM glucose and 10 mM N-(2-hydroxyethyl)piperazine-N′-(2-ethanesulfonic acid) (HEPES) was prepared at pH 6.8. The buffer for the basolateral chamber included the same components plus 2 mM CaCl2 and 1 mM MgCl2 at pH 7.4. Ca2+ and Mg2+ were omitted in the apical chamber in order to prevent precipitation of calcium and magnesium soaps of C10 and PCP-Cys complexes with Ca2+/Mg2+, respectively. This does not have any harmful influence on the intestinal membrane (CitationAnderberg et al., 1993). The intestinal membrane was pre-incubated for 30 min with these artificial fluids. After this pre-incubation buffer solutions were replaced by test solutions. Within these test solutions, NaFlu (10 µg/mL) and FD4 (1 mg/mL) were utilized as model compounds. Permeation of both model compounds was investigated in the absence of any permeation enhancer (control) and with 1% (w/v) PCP-Cys and 1% (w/v) C10, respectively. In order to investigate the full potential of PCP-Cys as a permeation enhancer, GSH was added to the apical chamber in a final concentration of 0.5% (w/v). Controls of GSH alone have been omitted as it has already been demonstrated that PCP-Cys significantly prolongs the activity of GSH (CitationClausen et al., 2002). Moreover, the permeation behavior of C10 itself was investigated in order to be able to estimate its absorption from the intestine and potential risks of systemic adverse effects. All experiments were carried out at 37°C. Samples of 100 µL were taken from the basolateral chamber after 0, 60, 120, and 180 min and replaced by an equal volume of the corresponding buffer.
NaFlu and FD4 concentrations within the samples were determined on a microplate fluorometer (Infinite™ M200, Tecan, Grödig, Austria). Cumulative corrections were made for previously removed samples in order to determine the total amount of permeated compound.
For C10 analysis the samples which have been withdrawn during permeation experiments were acidified with 10 µL 5 M HCl, extracted with 400 µL of ethyl acetate and spiked with pentadecanoic acid (0.1 mg/mL) as an internal standard. Aqueous solutions of increasing C10 concentrations were treated in the same manner for a calibration curve. C10 concentrations within the samples were analyzed via a gas chromatography method on an Agilent 6890N GC system coupled to a 5973inert mass selective detector in scan mode. Separation was performed on an Agilent DB-FFAP 30 m × 0.25 mm × 0.25 µm column with helium as carrier gas (1.2 mL/min). The injection mode was splitless with an injection volume of 1 µL and an inlet temperature of 280°C. The following temperature program was applied: 5 min at 100°C, then 15°C/min up to 250°C, then 12 min at 250°C.
Apparent permeability coefficients were calculated according to the following equation:
where Q (µg) is the total amount of permeated compound, A (0.64 cm2) the permeation area, c (µg/mL) the initial concentration, and t (10,800 s) the total time of the permeation experiment. Enhancement ratios were calculated from Papp values by the following equation:
Preparation of test formulations for in vivo studies
Twelve different formulations were prepared for in vivo studies. The composition of the different dosage forms is given in . GSH was included in PCP-Cys formulations in order to investigate the maximum potential of such delivery systems.
Table 1. Composition of dosage forms.
Solutions for intravenous application
Solutions for i.v. application were prepared by dissolving 2.5 mg of the particular model compound in 250 µL of sterile and isotonic phosphate buffered saline (PBS), pH 7.4.
Oral control solutions without permeation enhancers
Oral control solutions were prepared by dissolving 2.5 mg of either NaFlu or FD4 in 500 µL PBS, pH 7.4.
Oral solution containing C10
Five milligrams of C10 and 2.5 mg of the particular model compound were dissolved in 500 µL of PBS, pH 7.4, in order to evaluate the C10 permeation enhancing effect in solution.
PCP-Cys hydrogels
PCP-Cys hydrogels were produced by dissolving 2.5 mg NaFlu or FD4 and 0.5 mg GSH in 500 µL of PBS, pH 7.4. Then, 5 mg of PCP-Cys were added. The mixture was stirred until an homogenous mixture was obtained and PCP-Cys was completely swollen.
PCP-Cys minitablets
In order to obtain homogenous material that can be compressed to tablets, PCP-Cys (5 mg), NaFlu or FD4 (2.5 mg) and GSH (0.5 mg) were dissolved in demineralized water and the pH was adjusted to 6. These mixtures were stirred for 2 h, then frozen at −70°C, and finally lyophilized. The lyophilized product was compressed to minitablets with a diameter of 2.5 mm (Single punch tablet press, Paul Weber, Remshalden-Grünbach, Germany).
C10 minitablets
In order to obtain a homogenous mixture, 5 mg C10 and 2.5 mg of either model compound were blended in a mortar. Tablets of 7.5 mg weight were compressed to 2.5 mm flat-faced disks.
In vitro release of tablet formulations
Due to their small size, the in vitro release rates of NaFlu and FD4 from minitablets has been investigated by a method, which does not conform to one of the widely accepted pharmacopoeias. The in vitro release rates of both model compounds from tablets were determined by placing the tablets into 15 mL tubes containing 10 mL release medium (50 mm phosphate buffer, pH 6.8). The utilized volume of release medium displays the approximate volume of an intestine of a rat (250 g body weight) (CitationDavies & Morris, 1993). The closed tubes were then placed into an oscillating water bath and incubated at 37°C.
Cell viability studies
Caco-2 cells were sub-cultured on 24-well plates in minimum essential medium (MEM) containing 10% fetal calf serum (FCS) for 14 days to gain fully differentiated cells. MEM was exchanged every 48 h. FCS was omitted 1 day before the actual experiment.
In order to investigate potential effects on cell viability a 0.5% solution of sodium caprate and a 0.5% hydrogel of PCP-Cys were prepared and Caco-2 cells were incubated with those for 12 h. Pure MEM served as a positive control. After the incubation period the MTT assay was performed on Caco-2 cells (CitationCiapetti et al., 1993). Samples were removed from the plate and the cells were thoroughly washed three times with isotonic phosphate buffered saline (PBS). Afterwards, 500 µL of a MTT solution in FCS-free MEM (0.5 mg/mL) was added to each well and the cells were incubated for another hour. Thereafter, the supernatants were removed and the cells were washed two more times. The converted dye was solubilized with 1000 μL DMSO and mixed thoroughly for proper dissolution of the dye. This solution was centrifuged at 13,400 rpm for 2 min. The absorbance of the resulting solution was recorded immediately at λ = 570 nm. Cell viability was calculated as the percental absorbance of each sample corresponding to the absorbance of MEM controls.
In vivo investigations of oral bioavailability
The protocol for the in vivo studies on animals was approved by the Animal Ethical Committee of Vienna, Austria, and adheres to the Principles of Laboratory Animal Care. For the in vivo studies male Sprague-Dawley rats (240–250 g body weight) were used. Rats were housed in a constant temperature environment with a 12/12 h light/dark cycle and standard pellet diet. Rats were divided into 12 cohorts of six animals and fasted for 2 h before being treated with the different test formulations from . Intravenous injections were administered to the right tail vein. Liquid formulations and hydrogels were administered through a flexible plastic stomach tube with a round tip in order to minimize trauma. Tablets were administered by placing them deeply into the throat in order to initiate the swallow-reflex. Dosed animals were fasted for a further 2 h. Access to water was guaranteed throughout the whole experiment. Blood samples of 150 µL were taken from the left tail vein at pre-determined time points. After the experiment rats were sacrificed with an overdose of ketamine/xylazine.
The collected blood samples were spiked with 30 µL of a 3.8% sodium citrate solution in order to prevent blood clotting and were centrifuged. The supernatant plasma was collected and frozen at −70°C until further analysis. Prior to analysis, the plasma samples were mixed with an equal volume of ice-cold methanol and stored at 4°C overnight for deproteinization. The processed samples were finally analysed via high performance liquid chromatography (Hitachi LaChromeElite series, L-2130 pump, L-2200 auto sampler and L-2480 fluorescence detector) after appropriate dilution steps. NaFlu concentrations were determined with an isocratic method (65% 10 mM phosphate buffer (pH 7)/35 % acetonitrile) on a Supelcosil LC-8-DB column with a flow rate of 0.5 mL/min. FD4 concentrations were determined on a Vydac 218TP54 column with the same isocratic method as for NaFlu and a flow rate of 0.5 mL/min. Both substances were detected with a fluorescence detector (excitation wavelength λEx = 490 nm; emission wavelength λEm = 515 nm).
Statistical data analysis
The pharmacokinetic parameters of the model drugs after administration of test formulations were obtained by applying a non-compartment pharmacokinetic analysis to the plasma concentration/time data. This analysis was performed with the computer software OriginPro 7G SR5 version 7.0552 (OriginLab, Northhampton, MA). The area under the curve (plasma concentration vs time) was calculated for the time of the last measurement by means of the trapezoidal rule. Kinetic data from individual values were used. The absolute bioavailability of the model drugs were calculated from the area under curve values after intravenous administration.
Statistical data analysis was performed with the student t-test at p < 0.05 as the minimal level of significance.
Results
PCP-Cys conjugate characterization
PCP-Cys conjugates have previously been characterized in detail regarding mucoadhesive, cohesive, and swelling properties (CitationBernkop-Schnurch et al., 1999).
After lyophilization, a white and odorless product of fibrous structure was obtained. The conjugate which is used within this study displays a total amount of 594.08 ± 109.2 µmol thiol groups per gram polymer of which 468.9 ± 97.7 are present in their reduced form.
In vitro permeation studies across freshly excised rat intestinal mucosa
The permeation enhancing effects of 1% (w/v) C10 and 1% (w/v) PCP-Cys/0.5% (w/v) GSH on the transport rates of a hydrophilic small molecule (NaFlu) and a hydrophilic macromolecule (FD4) across freshly excised rat intestinal mucosa were evaluated in Ussing-type diffusion chambers. Moreover, the transport of C10 itself across the intestinal mucosa was investigated. The permeation behavior of all investigated compounds across the intestinal mucosa is depicted in and . Papp values and permeation enhancement ratios were calculated for interpretation purposes and are provided in .
Table 2. Comparison of the apparent permeability coefficients of model compounds in the presence of indicated permeation enhancers. Indicated values are means ± SD (n ≥ 4).
Figure 1. Cumulative transport of NaFlu in buffer (squares), of NaFlu with 1 % sodium caprate (diamonds), of NaFlu with 1 % PCP-Cys/0.5 % GSH (circles) and of sodium caprate (dashed line) across rat intestinal mucosa. Indicated values are means of at least three experiments ± standard deviation. 1,2 are not statistically different but differ significantly from 3.
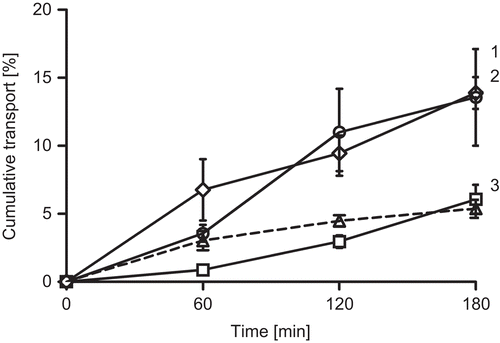
Figure 2. Cumulative transport of FD4 in buffer (squares), of FD4 with 1 % sodium caprate (diamonds), of FD4 with 1 % PCP-Cys/0.5 % GSH (circles) and of sodium caprate (dashed line) across rat intestinal mucosa. Indicated values are means of at least three experiments ± standard deviation. 1,2 are not statistically different but differ significantly from 3.
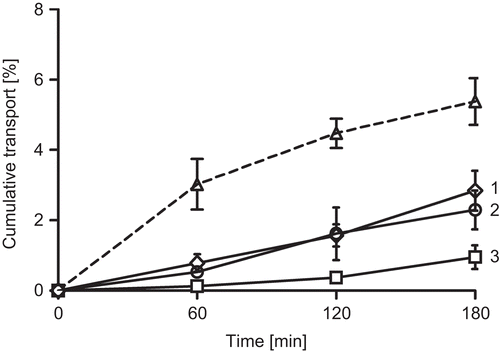
Results from these studies indicate that C10 as well as PCP-Cys/GSH can significantly improve permeation of hydrophilic compounds across rat intestinal mucosa. Within these experiments C10 caused a slightly more enhanced permeation of both model compounds across the mucosa. The difference between the permeation enhancing effects of 1% C10 and 1% PCP-Cys/0.5% GSH was found to be non-significant.
Moreover, it could be demonstrated that C10 itself is taken up across the intestinal mucosa. It was found that C10 permeates to a similar extent as NaFlu but more rapidly than FD4.
In vitro release studies
Release profiles of FD4 and NaFlu are presented in . Within 60–120 min, almost 100% of both model compounds are released from PCP-Cys tablets and both model compounds display similar release profiles. In comparison, C10 tablets dissolved in less than 5 min within the utilized release medium (data not shown). Consequently, drug release was completed within this time.
Cell viability studies
During cell viability experiments it was found that a 0.5% solution of C10 shows severely toxic effects on Caco-2 cell within 12 h, whereas a 0.5% dispersion of PCP-Cys displays a viability rate of over 80%. The observations regarding C10 are in good accordance with the results of CitationSakai et al. (1998), while cell viability rates for PCP-Cys are in a similar range as those obtained by CitationVetter et al. (2010). Results of the MTT assay are depicted in .
In vivo investigations of oral bioavailability
Besides permeation studies on freshly excised rat intestinal mucosa, the potential of both permeation enhancers was investigated in vivo. Plasma concentration-time curves of NaFlu and FD4 in combination with the two different permeation enhancers as liquid and solid dosage forms are provided in and . The corresponding pharmacokinetic parameters are summarized in .
Table 3. Pharmacokinetic parameters after oral administration of NaFlu and FD4 with C10 and PCP-Cys as permeation enhancers.
Figure 5. Sodium fluoresceine plasma concentration-time profiles after administration of liquid formulations (A) and tablets (B) with 5 mg sodium caprate (squares) and 5 mg PCP-Cys/0.5 mg GSH (circles) in comparison to buffer control solution (diamonds). Indicated values are means of at least three experiments ± standard deviation.
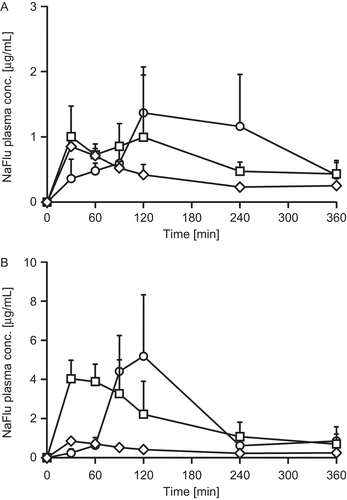
Figure 6. FD4 plasma concentration-time profiles after administration of liquid formulations (A) and tablets (B) with 5 mg sodium caprate (squares) and 5 mg PCP-Cys/0.5 mg GSH (circles) in comparison to buffer control solution (diamonds). Indicated values are means of at least three experiments ± standard deviation.
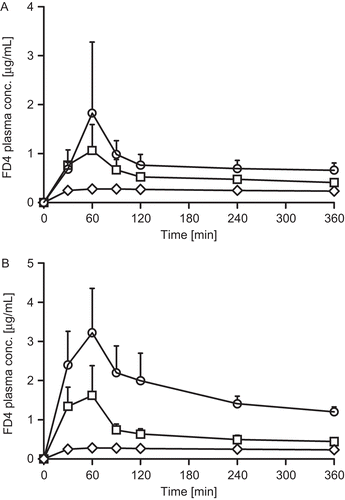
The bioavailability of NaFlu was in the same range with a non-significant difference when C10 or PCP-Cys/GSH, respectively, were co-administered in liquid formulations. Similarly, the bioavailability of FD4 did not differ significantly after administration of liquid C10 or PCP-Cys/GSH formulations, respectively. After administration of tablets comprising these different types of excipients, a similar bioavailability of NaFlu was observed with either adjuvant, whereas the bioavailability of FD4 was significantly higher after administration of PCP-Cys/GSH tablets in comparison to C10.
Discussion
Permeation enhancers of different chemical structure have widely been studied as adjuvants for oral drug delivery of drugs with a low bioavailability. Under physiological conditions, absorption of hydrophilic molecules and hydrophilic macromolecules in particular is drastically hampered by the intestinal epithelium and especially its tight junctions. In this present study, C10 and PCP-Cys/GSH increased the transport of NaFlu and FD4 across rat intestinal mucosa, indicating a notable disrupting impact on the intestinal barrier. C10 showed a more pronounced permeation in the first hour of the experiment, while PCP-Cys showed a better permeation in the final 2 h. These findings might be attributed to the different mechanisms of action as well as the fact that C10 itself diffuses across the membrane, which reduces the amount of permeation enhancer in the apical compartment. The obtained results for PCP-Cys correlate well with the conclusions drawn by CitationClausen et al. (2002) and CitationVetter et al. (2010) regarding the permeation enhancing effects on the paracellular transport of NaFlu and FD4. The slight evident differences to these studies might be attributed to the different tissues that have been utilized. The results regarding the permeation enhancing effect of C10 on hydrophilic small molecules as well as macromolecules coincide with previous studies (CitationMaher et al., 2009). Additionally, it could be demonstrated that C10 itself permeates across intestinal membranes. Hence, it can be assumed that its permeation behavior as well as dilution across the GIT, related to its good solubility in water, may potentially limit its effect under in vivo conditions.
The permeation enhancing effects of C10 are well investigated within cell culture models as well as transport studies across intact intestinal epithelia. It is widely accepted that C10 is capable of increasing the paracellular transport of certain drugs since various paracellular markers like mannitol (CitationChao et al., 1999), fluorescein isothiocyanate labeled dextrans of different molecular masses (CitationLindmark et al., 1998), or heparin (CitationCho et al., 2002) were utilized in numerous experiments. Nevertheless, only a few studies have been published reporting on successful oral drug delivery by means of co-administration of C10. Besides, results from these studies are not completely coherent. CitationLin et al. (2006) were able to increase the bioavailability of Ophiopogon japonicus polysaccharide in rats only after intraduodenal administration 20-fold by designing a C10-containing matrix tablet that released drug and permeation enhancer in a synchronized manner. The bioavailability of heparin in rats could be increased almost 3-fold with C10 in solution with a C10 dose of 100 mg/kg (CitationMotlekar et al., 2005). On the contrary, CitationZornoza et al. (2004) state that C10 did not improve the oral bioavailability of acamprosate in rats. Improved intestinal absorption of a model decapeptide by co-administration of C10 could be demonstrated after intrajejunal instillation (CitationChao et al., 1999).
PCP-Cys, which was compared to C10 within this study, could be demonstrated to improve the bioavailability of human growth hormone on the nasal route and low molecular weight heparin on the oral route significantly (CitationKast et al., 2003; CitationLeitner et al., 2004). However, these studies do not allow a clear assessment of the general performance of such systems for oral drug delivery. Therefore, within the current study, oral bioavailability of NaFlu and FD4 was investigated in rats after co-administration of PCP-Cys/GSH as well as C10 in order to gain further insight into the potential of PCP-Cys/GSH systems. Liquid formulations of both permeation enhancers did not show any significant difference between each other for neither of the model compounds. These observations underline the results of the in vitro results and suggest that both systems have a similar potential as permeation enhancers. Within in vivo studies with PCP-Cys tablets, a significantly higher bioavailability was observed for FD4 in comparison to all other FD4-containing formulations. Since liquid formulations have shown similar effects, it is assumed that mucoadhesive and release sustaining properties of PCP-Cys contribute to this increase. These properties would lead to less distinct dilution effects during the GIT passage and provide higher local concentrations of the model compound. Interestingly, no significant difference could be observed between NaFlu-containing tablets based on C10 and PCP-Cys, despite their different solubility and release features of the adjuvants. However, both tablets displayed a significantly higher bioavailability than any other formulation within this study. This can possibly be explained by the poor solubility of NaFlu under acidic conditions. NaFlu probably did not precipitate after administration of solutions as the acid of the stomach was sufficiently diluted by the buffer of the formulation. Nevertheless, it can be assumed that NaFlu was not dissolved in the stomach after tablet application. Hence, dilution would be reduced and an increased amount of NaFlu would be present in the intestine for absorption. If increased amounts of NaFlu reach the intestine due to its solubility, mucoadhesive and sustained release features of PCP-Cys are at least partially evened out. However, the results of these studies suggest that PCP-Cys/GSH can be a suitable alternative to permeation enhancers of the fatty acid type, at least for oral application. In order to judge the potential on other routes of drug administration, further studies will be necessary.
Cell viability studies with PCP-Cys and C10 could demonstrate that PCP-Cys is better tolerated by cultured cells under the chosen conditions. As already demonstrated by CitationSakai et al. (1998), C10 shows severe cytotoxic effects at a concentration of 0.5%, which still leads to controversial discussions regarding the value of C10 as a permeation enhancer. During this study the MTT assay revealed distinct cytotoxic effects for C10 in this concentration (viability rates of less than 10%), whereas viability rates of more than 80% for PCP-Cys might allow risk-free drug administration. Moreover, it could be demonstrated within the scope of this study that C10 itself is well absorbed across intestinal membranes. Consequently, systemic side-effects cannot be excluded when high amounts of C10 are administered in order to improve drug uptake. Regarding PCP-Cys, it was already demonstrated that polyacrylates are not absorbed from the intestine at all (CitationRiley et al., 2001). Accordingly, systemic toxic side-effects of PCP-Cys can be excluded.
It has been known for several years now that certain thiolated polymers show improved permeation enhancing properties compared to the corresponding unmodified polymers. The results of this present study add valuable new information to this research area, as it could be demonstrated for the first time that PCP-Cys elicits permeation enhancing properties similar to those of sodium caprate, besides good physiological compatibility and sustained release features.
Conclusion
Within the framework of this study, PCP-Cys/GSH was evaluated as a permeation enhancing system in comparison to the established adjuvant sodium caprate. In vitro permeation studies on freshly excised rat intestine revealed that PCP-Cys and C10 are comparatively potent permeation enhancers. These results were backed up by in vivo experiments in which PCP-Cys/GSH and C10 were found to be similarly potent adjuvants in liquid formulations. As C10 is well-established as a permeation enhancer and already commercialized, PCP-Cys must be recognized as a promising addition to the permeation enhancement field since it shows comparable effects. Results of in vivo experiments with minitablets indicate that further properties of PCP-Cys like mucoadhesion and sustained release may contribute to an additional increase in bioavailability of potential drugs. Furthermore, results of this study indicate a good biocompatibility of PCP-Cys, which does not bear the risk of systemic side-effects.
Declaration of Interest
The authors report no conflicts of interest. The authors alone are responsible for the content and writing of the paper.
References
- Anderberg, E.K., Lindmark, T., Artursson, P. (1993). Sodium caprate elicits dilatations in human intestinal tight junctions and enhances drug absorption by the paracellular route. Pharm Res. 10:857–64.
- Aungst, B.J. (2000). Intestinal permeation enhancers. J Pharm Sci. 89:429–42.
- Bernkop-Schnurch A., Schwarz V., Steininger, S. (1999). Polymers with thiol groups: a new generation of mucoadhesive polymers? Pharm Res. 16:876–81.
- Chao, A.C., Nguyen, J.V., Broughall, M., Griffin, A., Fix, J.A., Daddona, P.E. (1999). In vitro and in vivo evaluation of effects of sodium caprate on enteral peptide absorption and on mucosal morphology. Int J Pharm. 191:15–24.
- Chavanpatil, M.D., Vavia, P.R. (2005). Nasal drug delivery of sumatriptan succinate. Pharmazie. 60:347–9.
- Cho, S.Y., Kim, J.S., Li, H., Shim, C., Linhardt, R.J., Kim, Y.S. (2002). Enhancement of paracellular transport of heparin disaccharide across Caco-2 cell monolayers. Arch Pharm Res. 25:86–92.
- Ciapetti, G., Cenni, E., Pratelli, L., Pizzoferrato, A. (1993). In vitro evaluation of cell/biomaterial interaction by MTT assay. Biomaterials. 14:359–64.
- Clausen, A.E., Bernkop-Schnurch, A. (2000). In vitro evaluation of the permeation-enhancing effect of thiolated polycarbophil. J Pharm Sci. 89:1253–61.
- Clausen, A.E., Kast, C.E., Bernkop-Schnurch, A. (2002). The role of glutathione in the permeation enhancing effect of thiolated polymers. Pharm Res. 19:602–8.
- Davies, B., Morris, T. (1993). Physiological parameters in laboratory animals and humans. Pharm Res. 10:1093–5.
- Diamond, J.M. (1977). Twenty-first Bowditch lecture. The epithelial junction: bridge, gate, and fence. Physiologist. 20:10–18.
- Habeeb, A.F. (1973). A sensitive method for localization of disulfide containing peptides in column effluents. Anal Biochem. 56:60–5.
- Hayashi, M., Tomita, M., Awazu, S. (1997). Transcellular and paracellular contribution to transport processes in the colorectal route. Adv Drug Deliv Rev. 28:191–204.
- Ibrahim, S.A., Li, S.K. (2010). Efficiency of fatty acids as chemical penetration enhancers: mechanisms and structure enhancement relationship. Pharm Res. 27:115–25.
- Kast, C.E., Guggi, D., Langoth, N., Bernkop-Schnurch, A. (2003). Development and in vivo evaluation of an oral delivery system for low molecular weight heparin based on thiolated polycarbophil. Pharm Res. 20:931–6.
- Leitner, V.M., Guggi, D., Bernkop-Schnurch, A. (2004). Thiomers in noninvasive polypeptide delivery: in vitro and in vivo characterization of a polycarbophil-cysteine/glutathione gel formulation for human growth hormone. J Pharm Sci. 93:1682–91.
- Lin, X., Xu, D.S., Feng, Y., Li, S.M., Lu, Z.L., Shen, L. (2006). Release-controlling absorption enhancement of enterally administered Ophiopogon japonicus polysaccharide by sodium caprate in rats. J Pharm Sci. 95:2534–42.
- Lindmark, T., Schipper, N., Lasorova, L., De Boer, A.G., Artursson, P. (1998). Absorption enhancement in intestinal epithelial Caco-2 monolayers by sodium caprate: assessment of molecular weight dependence and demonstration of transport routes. J Drug Target. 5:215–23.
- Maher, S., Kennelly, R., Bzik, V.A., Baird, A.W., Wang, X., Winter, D., Brayden, D.J. (2009). Evaluation of intestinal absorption enhancement and local mucosal toxicity of two promoters. I. Studies in isolated rat and human colonic mucosae. Eur J Pharm Sci. 38:291–300.
- Motlekar, N.A., Srivenugopal, K.S., Wachtel, M.S., Youan, B.B. (2005). Oral delivery of low-molecular-weight heparin using sodium caprate as absorption enhancer reaches therapeutic levels. J Drug Target. 13:573–83.
- Riley, R.G., Green, K.L., Smart, J.D., Tsibouklis, J., Davis, J.A., Hampson, F., Dettmar, P.W., Wilber, W.R. (2001). The gastrointestinal transit profile of 14C-labelled poly(acrylic acids): an in vivo study. Biomaterials. 22:1861–7.
- Sakai, M., Imai, T., Ohtake, H., Otagiri, M. (1998). Cytotoxicity of absorption enhancers in Caco-2 cell monolayers. J Pharm Pharmacol. 50:1101–8.
- Vetter, A., Martien, R., Bernkop-Schnurch, A. (2010). Thiolated polycarbophil as an adjuvant for permeation enhancement in nasal delivery of antisense oligonucleotides. J Pharm Sci. 99:1427–39.
- Wachtel, M., Frei, K., Ehler, E., Fontana, A., Winterhalter, K., Gloor, S.M. (1999). Occludin proteolysis and increased permeability in endothelial cells through tyrosine phosphatase inhibition. J Cell Sci. 112:4347–56.
- Zornoza, T., Cano-Cebrian, M.J., Nalda-Molina, R., Guerri, C., Granero, L., Polache, A. (2004). Assessment and modulation of acamprosate intestinal absorption: comparative studies using in situ, in vitro (CACO-2 cell monolayers) and in vivo models. Eur J Pharm Sci. 22:347–56.