Abstract
The aim of this work was to prepare salts of 6-methoxy-2-naphthylacetic acid (6-MNA) to improve its physicochemical properties for percutaneous application. 6-MNA, an active metabolite of non-steroidal anti-inflammatory drug nabumetone has long half life and has the tendency to penetrate well into synovial fluid. The physicochemical properties of 6-MNA salts were investigated by solubility measurements, differential scanning calorimetry (DSC) and infrared (IR). The DSC thermograms and Fourier transform infrared (FT-IR) spectra indicated that 6-MNA formed salts with organic and alkali metal bases. Among the series, salts formed with amine bases (ethanolamine, diethanolamine, triethanolamine and diethylamine) had lower melting points while alkali metal salt (sodium) had higher melting point than 6-MNA. The salts had higher solubilities than 6-MNA as determined in phosphate buffer at pH 5.0 and 7.4. There is no significant difference in partition coefficient (log P) values between salts and 6-MNA at pH 5.0 but, at pH 7.4, the log P values for the salts increased by 4–10 times as compared to 6-MNA. In vitro permeation studies showed that all the salts increased the flux in comparision to 6-MNA, and the ethanolamine salt (1b) was found to be having 7.7 and 9.4 times higher permeability as compared to 6-MNA at pH 5.0 and 7.4, respectively.
Introduction
Non-steroidal anti-inflammatory drugs (NSAIDs) are widely used in symptomatic management of rheumatic diseases and other diverse forms of acute as well as chronic pain. Among the various therapeutic classes, NSAIDs is the most widely used class for the treatment of inflammatory conditions; however, its usage through oral route is limited due to gastrointestinal, cardiovascular and renal adverse effects (Evans et al., Citation1995a,Citationb; Ki & Choi, Citation2007; Motsko et al., Citation2006). Due to these adverse effects after oral administration, the usage of topical application of NSAIDs has increased, and the current guidelines also recommended use of topical NSAIDs (Altman, Citation2010). Topical use of the NSAIDs minimizes systemic concentration of the drugs, thereby avoiding these adverse effects and offering the desired local anti-inflammatory effect (Roberts & Cross, Citation1999). Topical administration of a drug has several other advantages such as protection of the drug from gastric and enzymatic degradation, bypassing hepatic first pass metabolism, provision of sustained release of the active agent at the site of application, ability to rapidly terminate therapy by removal of the formulation and low risk of systemic effects (Komatsu & Sakurada, Citation2012; Roberts & Cross, Citation1999).
Desipite these advantages, the percutaneous delivery of NSAIDs is not an easy task due to the barrier function of the skin, poor aqueous solubility of NSAIDs and low skin permeability (1–2%) in humans (Fang et al., Citation2003; Roberts & Cross, Citation1999; Rautio et al., Citation2000; Singh & Roberts, Citation1994; Suh et al., Citation1997; Yano et al., Citation1986). Furthermore, most of the NSAIDs have short biological half life hence they need to be applied quite frequently (Menassé et al., Citation1978).
Several strategies have been reported for enhancing the transdermal delivery of NSAIDs such as formulation approach, prodrug approach and salt/complex formation strategy. Unlike prodrug and formulation approach, the salt formation strategy enhances the skin transport of ionic drugs without modifying the molecular structure of the drug, changing the skin barrier function or using specific devices (Cheong & Choi, Citation2002). Selection of appropriate salt and prediction of influence of a particular chemical species on the behavior of the parent drug can be a difficult task because each salt possesses unique physicochemical properties (Komatsu & Sakurada, Citation2012). Salts generally have smaller dipoles and lower crystal lattice energies that result in higher solubilities in polar and non-polar solvents (Gerard & Mazzenga, Citation1991). The development of topical NSAID therapy that can achieve effective improvement in rheumatic pain with lower risk of NSAID-related adverse effects is important and further developments in this field are essential to improve rheumatic treatment paradigm.
Majority of the clinically used NSAIDs have short half life and less affinity toward joint cavity which adversely affects the treatment of rheumatic diseases. A recent study revealed that among the commercially available topical NSAID preparations used in Europan Union, only the topical ketoprofen preparations showed superior skin permeability (Komatsu & Sakurada, Citation2012). In inflammatory conditions, delivery of the drugs to underlying muscle and joints is important, and if there is a low clearance of the drug from the body, it increases deep tissue concentration (Lee & Maibach, Citation2006; Roberts & Cross, Citation1999). Hence, it is important that the NSAIDs used in rheumatic diseases must have long half life and possess affinity toward joint cavity.
In this regard, we chose 6-methoxy-2-naphthylacetic acid (6-MNA), an active metabolite of nabumetone for percutaneous drug delivery. It has advantages like having long half life (21–27 h), tendency to penetrate well into synovial fluid and absence of enterohepatic circulation (Davies, Citation1997; Friedel et al., Citation1993). Based on these favorable pharmacokinetic and pharmacodynamic properties of 6-MNA, we synthesized and evaluated various salts of 6-MNA for percutaneous drug delivery. Various organic and alkali metal bases, such as ethanolamine, diethanolamine, triethanolamine, diethylamine and sodium hydroxide, were used for the preparation of the salts. The differences between the physicochemical characteristics of 6-MNA and its salts were evaluated by using instrumental techniques like differential scanning calorimetry (DSC), Fourier transform infrared (FT-IR), scanning electron microscope (SEM) etc., and the impact of salt formation on percutaneous absorption was investigated in vitro using human cadavour skin.
Materials and methods
All the reagents and solvents required for syntheses were purified by general laboratory techniques before use. Purity of the compounds and completion of the reactions were monitored by thin layer chromatography on silica gel plates (60 F254; Merck, Germany), visualizing with ultraviolet light or iodine vapors. The yields reported here are unoptimized. Melting points were determined using a Veego make silicon oil bath-type melting point apparatus and are uncorrected. The IR spectra were recorded using KBr disc method in cm−1 on a Bruker FT-IR, Model 8300. The proton nuclear magnetic resonance spectroscopy (PMR) spectra were recorded in CDCl3 on a Bruker 400 MHz spectrometer (chemical shifts in δ ppm, coupling constant J in Hz). λmax were determined on Shimadzu 1800 UV spectrophotometer. Mass of the compounds was determined by LC-MS using electron impact as the source of ionization. DSC analysis was performed on Shimadzu DSC-60 model with thermal analyzer TA 60 WS. SEM model ESEM-EDAX XL-30 (Philips, The Netherlands) was used for the surface morphology study. In vitro skin permeation studies were conducted using Franz-type diffusion cell and human cadaver skin obtained from S.S.G. Hospital, Vadodara, Gujarat, India.
Synthesis of salts
6-MNA (1) required for the synthesis of the salts was prepared by the reported procedure (Arsenijevic et al., Citation1988; Furniss et al., Citation1996). 6-MNA (1) dissolved in dichloromethane and small amount of methanol was added to make the solution clear wherever required and reacted with equimolar amount of the base. The reaction mixtures were stirred for 6–8 h. The precipitated salts were collected by filtration and recrystallized from ethyl acetate to yield the pure salts (1a–1e) of 6-MNA.
High performance liquid chromatography analysis
High performance liquid chromatography (HPLC) was used for analysis. The analytical system consisted of an LC system (LC-20AD; Shimadzu, USA) and a UV-visible detector (SPD 20 A; Shimadzu). The chromatographic separation was carried out under isocratic reverse-phase conditions on purospher RP-18 C column (250 × 4.6 mm, 5 µ; Merck, Germany) with column temperature maintained at 25–28 °C. The injection volume used was 20 µl and the detection wavelength was 230 nm. The mobile phase was a mixture of 15 mM phosphate buffer and acetonitrile of pH 5.0–5.5 and the flow rate was 1.0 ml/min.
Aqueous solubility
The aqueous solubility of 6-MNA (1) and its salts (1a–1e) were determined in phosphate buffer (0.16 M) at pH 5.0 and 7.4 at room temperature. Excess amounts of each salt individually were added to phosphate buffer (0.5 ml). The mixtures were stirred and centrifuged at 8000 rpm for 5 min. The concentrations of each of the salts in their saturated solutions were analyzed by the HPLC. The pH of the solutions was held constant throughout the experiment (Rautio et al., Citation2000; Singh & Roberts, Citation1994).
Apparent partition coefficient (log Papp)
The apparent partition coefficients (log Papp) of 6-MNA (1) and its salts (1a–1e) were determined at room temperature between 1-octanol and phosphate buffer (0.16 M) at pH 5.0 and 7.4 using shake flask method. 1-Octanol was saturated with phosphate buffer for 24 h by stirring vigorously before use. A known concentration of the compound in phosphate buffer was shaken with a suitable fixed volume of 1-octanol. After shaking, both the phases were separated by centrifugation at 8000 rpm for 5–6 min. The concentrations of the compounds in the aqueous phase and in the 1-octanol phase were determined by HPLC (Rautio et al., Citation2000; Singh & Roberts, Citation1994).
In vitro skin permeation studies
In vitro skin permeation studies were performed by using glass Franz diffusion cell and excised human cadaver skin. Skin specimens were rehydrated before being mounted in the diffusion cell. The receptor medium (0.05 M phosphate buffer saline solution of pH 7.4) was stirred and kept at 37 ± 1 °C throughout the study. The compounds were applied as solutions in phosphate buffer (0.05 M) at pH 5.0 and 7.4. Infinite dose technique was used to determine the permeation profile of 6-MNA and the salts in which excess amount of the test compound was used and the increase in the amount of the salt and/or the parent along the experiments time frame was measured (Walters, 2000). At specified time intervals, aliquots (0.25 ml) were withdrawn from the receptor compartment and replaced with fresh buffer and the drug concentrations were analyzed by HPLC.
The steady-state flux for 6-MNA (1) and its salts (1a–1e) were determined by plotting the cumulative amount of the parent drug as measured in the receiver phase against time, and dividing the slope of the steady-state position by the surface area of the diffusion cell (4.906 cm2). The permeability coefficients (Kp) for the steady-state delivery were obtained by dividing the steady-state flux (Jss) by the solubilities of the compounds in the corresponding vehicle (Rautio et al., Citation2000; Singh & Roberts, Citation1994).
Statistical analysis
For ascertaining the statistical significance of the data, Graph Pad Prism software was used. Data are presented as mean ± standard deviation (n = 3–6). Groups were compared using analysis of variance (ANOVA) one-way test. Differences were considered statistically significant when p < 0.05.
Results and discussion
Syntheses of 6-MNA salts
6-MNA (1) required for the synthesis of the salts was prepared by the reported procedure (Arsenijevic et al., Citation1988; Furniss et al., Citation1996). In this study, some salts of 6-MNA with organic and alkali metal bases have been synthesized.
Alkanolamines such as ethanolamine, diethanolamine, triethanolamine and diethylamine (DEA) have been chosen for this purpose along with sodium salt. These alkanolamines are weak bases, and contain basic amino functional group and one or more polar hydroxyl groups except for DEA. Amino group will react with acidic functional group present in the 6-MNA resulting in salt formation, imparting aqueous solubility to the product, which is further enhanced by polar hydroxyl groups. Structures of the designed salts are shown in .
Characterization of salts
In order to confirm salt formation, various techniques such as FT-IR, NMR, DSC and SEM have been used to characterize the prepared salts. Physicochemical and spectral data of the compounds are listed in .
Table 1. Physicochemical and spectral data of compounds (1, 1a–1e).
Regarding the interaction between 6-MNA and alkanolamine bases, important information was gathered from IR spectroscopy. The FT-IR spectra of 6-MNA (1) and its salts are shown in . 6-MNA showed a strong sharp signal at 1693 cm−1, characteristic of the carbonyl stretching vibrations. The most obvious difference between the IR spectra of 6-MNA and its salts was found in the O–H and N–H stretching regions. 6-MNA salts (1a–1e) show peaks in the range of 3389–3285 cm−1 due to N–H stretching vibrations which were absent in 6-MNA. These results indicate a change in the structure of 6-MNA by salt formation. Salt 1b shows signal at 3285 cm−1 whereas 1c and 1d show peaks around 3385 cm−1, suggesting that the intermolecular hydrogen bonding in the salts might have shifted the N–H or O–H stretching bands.
Support for salt formation was also obtained from PMR spectra of MNA and its ethanolamine salt (1b). In the PMR spectrum, aromatic protons of 6-MNA appeared at δ 7.70–7.08 (Ar–H), and singlets appeared at δ 3.87 (3H; OCH3) and 3.68 (2H; –CH2) for methoxy and methylene protons, respectively. In case of ethanolamine salt, additional peaks appeared at δ 3.56–3.53 and 2.78–2.76 for the two methylene groups as triplets and a broad peak at δ 6.47 due to protons of ammonium and hydroxyl group.
shows DSC curve of 6-MNA (1) and its salts (1a–1e) in the experimental temperature range of 30–300 °C. The DSC curve of MNA shows a characteristic sharp endothermic peak of the drug at 171.55 °C corresponding to its melting point (171–173 °C). These values are in good agreement with each other. Significant decrease in melting point of the salts was observed as compared to 6-MNA except for sodium salt. The shift in melting points is the result of salt formation of 6-MNA. The melting points and DSC endothermic peak values of 6-MNA and its salts are listed in .
Surface morphology of the compounds varies from each other, and to evaluate this parameter, SEM of the prepared salts was performed. From this study, it was concluded that each salt has a different surface morphology as shown in .
Figure 4. Scanning electron microscopic images of compounds (1, 1a–1e).
Physicochemical evaluation
For the evaluation of various physicochemical parameters such as aqueous solubility, partition coefficient and in vitro skin permeability, HPLC method of analysis was developed. The calibration curves for 6-MNA (1) were plotted and linearity range was calculated.
Determination of aqueous solubility
Due to the biphasic nature of skin, an ideal salt form should exhibit adequate lipid solubility as well as aqueous solubility. Aqueous solubility of 6-MNA (1) and its salts (1a–1e) was determined in phosphate buffer at pH 5.0 and pH 7.4 as the environment of the outer surface of the skin is acidic (pH 4.2–6.5) (Rautio et al., Citation2000; Suh et al., Citation1997) and the values so obtained were given in . shows aqueous solubility of 6-MNA (1) and its salts (1a–1e). It can be concluded that 6-MNA has low solubility at pH 5.0 and higher solubility at pH 7.4, but all the salts have shown balanced solubilities at both pH conditions.
Table 2. Aqueous solubility and partition coefficient (log Papp) values of 6-MNA (1) and its salts at pH 5.0 and at pH 7.4.
Determination of apparent partition coefficient (log Papp)
Lipid solubility plays a crucial role in determining skin permeability of a compound because stratum corneum, the major barrier to drug permeation, is essentially lipoidal in nature and generally favors permeation of lipophilic drugs (Rautio et al., Citation2000). The apparent partition coefficients of 6-MNA (1) and salts (1a–1e) were determined by partitioning them between phosphate buffer (0.16 M) and saturated 1-octanol at both pH 5.0 and 7.4 using shake flask method. and show log Papp values of 6-MNA (1) and its salts (1a–1e). From , we can conclude that at pH 5.0, MNA shows higher lipophilicity than at pH 7.4 due to higher ionization of 6-MNA at pH 7.4 than 5.0. Furthermore, except salt (1e) all the salts (1a–1d) maintained lipophilicity in the range of 0.70–1.49 at both pH conditions.
In vitro skin permeation study
In vitro skin permeation study was performed using human cadaver skin. These diffusion experiments showed that salts of 6-MNA were able to permeate the skin. For each salt and parent drug, the cumulative amounts permeated through skin were plotted against time. A steady-state flux (Jss) was obtained by dividing the slope of that graph by surface area of the diffusion cell (4.906 cm2) (Rautio et al., Citation2000). The steady-state flux (Jss) of 6-MNA (1) and its salts (1a–1e) are given in and . All the salts have shown higher flux values than the parent NSAID (6-MNA). Among the salts, salt (1b) has shown the highest steady-state flux as shown in .
Figure 7. Steady state flux (Jss) of 6-MNA (1) and the salts (1a–1e) through excised human cadaver skin in vitro at 37 °C.
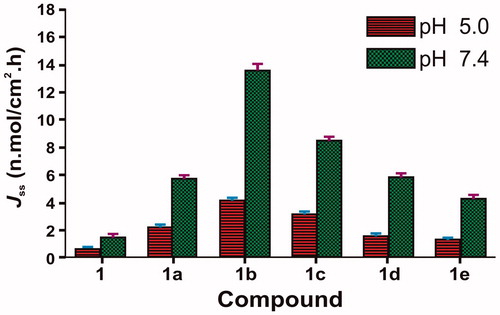
Table 3. Steady-state flux (Jss) and permeability coefficients (Kp) of 6-MNA (1) and the salts (1a–1e) through excised human cadaver skin in vitro at 37 °C.
As shown in , the ethanolamine salt (1b) displayed higher flux than the parent NSAID (6-MNA). The result also showed that the salts with higher flux have a good balance between aqueous solubility and partition coefficient. Furthermore, except for sodium salt, all the salts have lower melting points than the parent drug and higher permeability through the skin, which support previous reports, suggesting that a decrease in melting point or conversion of solid state to liquid state improves the permeability of drugs through skin (Singh & Singh, Citation1993).
Conclusion
Based on the results, it could be concluded that temporary masking of the carboxylic group of 6-MNA by salt formation is a useful strategy to improve percutaneous penetration. The salt formation approach for long-acting compounds having affinity toward joint tissue will increase local tissue concentration, minimize frequency of application and hence could be useful for the effective treatment of rheumatic diseases.
Acknowledgements
The authors thank All India Council for Technical Education (AICTE) New Delhi, India, for the award of National Doctoral Fellowship and The M.S. University of Baroda, Vadodara for the award of Junior Research fellowship to Vijay Pawar.
Declaration of interest
The authors report no conflicts of interest. The authors alone are responsible for the content and writing of this article.
References
- Altman RD. (2010). New guidelines for topical NSAIDs in the osteoarthritis treatment paradigm. Curr Med Res Opin 26:2871–6
- Arsenijevic L, Arsenijevic V, Horeau A, Jacques J. (1988). Synthesis of 2-acetyl-6-methoxynaphthalene. Org Syn 6:34–6
- Cheong HA, Choi HK. (2002). Enhanced percutaneous absoption of piroxicam via salt formation with ethanolamines. Pharm Res 19:1375–80
- Davies NM. (1997). Clinical pharmacokinetics of nabumetone. The dawn of selective cyclo-oxygenase-2 inhibition? Clin Pharmacokinet 33:404–16
- Evans JM, McMahon AD, McGilchrist MM, et al. (1995a). Topical non-steroidal anti-inflammatory drugs and admission to hospital for upper gastrointestinal bleeding and perforation: a record linkage case-control study. BMJ 311:22–6
- Evans JM, McGregor E, McMahon AD, et al. (1995b). Non-steroidal anti-inflammatory drugs and hospitalization for acute renal failure. QJM 88:551–7
- Fang L, Numajiri S, Kobayashi D, Morimoto Y. (2003). The use of complexation with alkanolamines to facilitate skin permeation of mefenamic acid. Int J Pharm 262:13–22
- Friedel HA, Langtry HD, Buckley MM. (1993). Nabumetone: a reappraisal of its pharmacology and therapeutic use in rheumatic diseases. Drugs 45:131–56
- Furniss BS, Hannaford JH, Smith PWG, Tachell AR. (1996). Vogel’s text book of practical organic chemistry, 5th edition. Singapore: Longman, 987–1054
- Gerard C, Mazzenga B. (1991). The transdermal delivery of zwitterionic drugs I: the solubility of zwitterion salts. J Control Rel 16:77–88
- Ki HM, Choi HK. (2007). The effect of meloxicam/ethanolamine salt formation on percutaneous absorption of meloxicam. Arch Pharm Res 30:215–21
- Komatsu T, Sakurada T. (2012). Comparision of the efficacy and skin permeability of topical NSAID preparations used in Europe. Eur J Pharm Sci 18:890–5
- Lee CM, Maibach HI. (2006). Deep percutaneous penetration into muscles and joints. J Pharm Sci 95:1405–13
- Motsko SP, Rascati KL, Busti AJ, et al. (2006). Temporal relationship between use of NSAIDs, including selective COX-2 inhibitors, and cardiovascular risk. Drug Saf 29:621–32
- Menassé R, Hedwall PR, Kraetz J, et al. (1978). Pharmacological properties of diclofenac sodium and its metabolites. Scand J Rheumatol 22:5–16
- Rautio J, Nevalainen T, Taipale H, et al. (2000). Piperazinylalkyl prodrugs of naproxen improve in vitro skin permeation. Eur J Pharm Sci 11:157–63
- Roberts MS, Cross SE. (1999). Percutaneous absorption of topically applied NSAIDs and other compounds: role of solute properties, skin physiology and delivery system. Inflammopharmacology 7:339–50
- Singh S, Singh J. (1993). Transdermal drug delivery by passive diffusion and iontophoresis: a review. Med Res Rev 13:569–621
- Singh P, Roberts MS. (1994). Skin permeability and local tissue concentrations of nonsteroidal anti-inflammatory drugs after topical application. J Pharmacol Exp Ther 268:144–51
- Suh H, Jun HW, Dzimianski MT, Lu GW. (1997). Pharmacokinetic and local tissue disposition studies of naproxen following topical and systemic administration in dogs and rats. Biopharm Drug Disp 18:623–33
- Yano T, Nakawaga A, Tsuji M, Noda K. (1986). Skin permeability of various non-steroidal anti-inflammatory drugs in man. Life Sci 39:1043–50