Abstract
Context: Solid lipid nanoparticles (SLN) are drug carriers possessing numerous features useful for topical application. A copious scientific literature outlined their ability as potential delivery systems for lipophilic drugs, while the entrapment of a hydrophilic drug inside the hydrophobic matrix of SLN is often difficult to obtain.
Objective: To develop SLN intended for loading caffeine (SLN-CAF) and to evaluate the permeation profile of this substance through the skin once released from the lipid nanocarriers. Caffeine is an interesting compound showing anticancer and protective effects upon topical administration, although its penetration through the skin is compromised by its hydrophilicity.
Materials and methods: SLN-CAF were formulated by using a modification of the quasi-emulsion solvent diffusion technique (QESD) and characterized by PCS and DSC analyses. In vitro percutaneous absorption studies were effected using excised human skin membranes (i.e. Stratum Corneum Epidermis or SCE).
Results: SLN-CAF were in a nanometric range (182.6 ± 8.4 nm) and showed an interesting payload value (75% ± 1.1). DSC studies suggest the presence of a well-defined system and the successful drug incorporation. Furthermore, SLN-CAF generated a significantly faster permeation than a control formulation over 24 h of monitoring.
Discussion and conclusions: SLN-CAF were characterized by valid dimensions and a good encapsulation efficiency, although the active to incorporate showed a hydrophilic character. This result confirms the suitability of the formulation strategy employed in the present work. Furthermore, the in vitro evidence outline the key role of lipid nanoparticles in enhancing caffeine permeation through the skin.
Introduction
Caffeine (1,3,7-trimethylxanthine) is a natural alkaloid found in tea leaves, coffee, cocoa, guarana and kola nuts. This active shows potential anticancer effects upon oral and topical administration, and is able to protect the skin from ultraviolet (UV) light induced skin cancer (Dias et al., Citation1999; Koo et al., Citation2007; Lu et al., Citation2007; Roure et al., Citation2011; Gee et al., Citation2012).
Cosmetic use of topical caffeine is also of interest as this agent is included in anti-cellulite creams/gels where it is known to favour the liberation of triglycerides and carnitine, stimulating the transfer of free fatty acids into the mitochondria in order to produce energy. Caffeine-based products are promoted to improve skin aspect although few clinical studies have been published related to their efficacy (Koo et al., Citation2007; Roure et al., Citation2011).
Notwithstanding these interesting features, topical administration of caffeine is compromised by its hydrophilicity (logP = −0.07) which make its penetration through the stratum corneum difficult (Dias et al., Citation1999).
Different approaches have been studied to overcome this limitation and to enhance the penetration of caffeine through the skin, but not always with excellent results (Yamamura et al., Citation1996; Bolzinger et al., Citation2008; Shakeel & Ramadan, Citation2010).
Solid lipid nanoparticles (SLN) are colloidal carrier systems composed of a high melting point lipid as a solid core coated by surfactants. They show many advantages such as feasibility of incorporation of lipophilic and hydrophilic drugs, improved physical stability, low cost and ease of scale up and manufacturing (Müller et al., Citation2002).
Furthermore, these lipid nanocarriers possess a number of important features useful for topical application. In fact, their particle size ensures high adhesion to the stratum corneum thus enhancing the amount of encapsulated agent, which penetrates into the viable skin. Furthermore, particles with size ranging from ∼200–400 nm show an occlusive effect due to a film formation on the skin, which can enhance drug penetration into specific skin layers (Ricci et al., Citation2005; Puglia & Bonina, Citation2012). Different examples regarding the benefits associated to the application of lipid nanoparticle strategy to the formulation of products aimed to dermal administration are reported in literature (Puglia et al., Citation2008; Mitri et al., Citation2011; Aggarwal & Goindi, Citation2013).
It is widely recognized that SLN are potential delivery systems for lipophilic drugs where aqueous solubility of the drug is the limiting factor for their absorption. Conversely, the entrapment of a hydrophilic drug inside the hydrophobic matrix of SLN is a real challenge, in fact most of the examples reported in literature, regarding hydrophilic molecules, show lipid nanoparticles characterized by a low payload and often realized using toxic organic solvents, which could compromise the safety profile of the formulated nanocarriers (Hu et al., Citation2004; Trotta et al., Citation2005; Gallarate et al., Citation2009; Kang et al., Citation2009).
The aim of the present study was to develop and characterize SLN intended for loading caffeine (SLN-CAF). The nanocarriers were formulated by using a modification of the quasi-emulsion solvent diffusion technique (QESD), a particular approach to the general solvent-change method (Kawashima et al., Citation1989). SLN-CAF were characterized by photocorrelation spectroscopy (PCS) and DSC studies were performed to obtain information regarding the crystalline structure of lipid matrix. In vitro percutaneous absorption studies were effected using excised human skin membranes (i.e. Stratum Corneum Epidermis or SCE) to evaluate the permeation profile of caffeine through the skin once released from the lipid nanocarriers.
Materials and methods
Materials
Caffeine and xanthan gum were purchased from Sigma–Aldrich (ST. Louis, MO). Softisan 100 (Hydrogenated Coco-Glycerides) was obtained by Sasol Germany (Hamburg, Germany). Pluronic® F68 (poloxamer 188) was purchased from BASF (Florham Park, NJ). High-performance liquid chromatography (HPLC)-grade solvents and water were purchased from Carlo Erba Reagents (Milan, Italy). When not specified, chemicals and reagents were of the highest purity grade commercially available.
SLN preparation
Blank and SLN-CAF were prepared by using a variation of the QESD method (Kawashima et al., Citation1989). Briefly, caffeine (0.408 g) was dissolved in 5 ml of deionized water heated to 50 °C approximately. The resulting solution was kept under constant magnetic stirring. The oil phase constituted by Softisan 100 (0.816 g), ethanol (18 ml) and water (4 ml) was heated at 50 °C and slowly added to the aqueous solution containing caffeine.
The dispersion thus obtained was subjected to the action of a high-speed homogenizer (UltraTurrax T25; IKA-Werke GmbH & Co. KG, Staufen, Germany at 24 000 rpm) and then it was slowly added into a hot (50 °C) surfactant solution (9 ml of water in which were dissolved 0.7 g of Pluronic F68). Finally, SLN were precipitated in an ice bath by quickly adding cold water (200 ml) to the quasi-emulsion under high-speed homogenization. After stirring (30 min), the suspension was filtered and stored at 4 °C for further characterization.
Characterization of SLN-CAF
Particle size distribution and zeta potential measurements
Mean particle size of the lipid dispersions was measured by photon correlation spectroscopy (PCS). A Zetamaster (Malvern Instrument Ltd., Sparing Lane South, Worcs, England), equipped with a solid-state laser having a nominal power of 4.5 mW with a maximum output of 5 mW 670 nm, was employed. Analyses were performed using a 90° scattering angle at 20 ± 0.2 °C. Samples were prepared by diluting 10 µl of SLN suspension with 2 ml of deionized water previously filtered through a 0.2 -µm Acrodisc LC 13 PVDF filter (Pall-Gelman Laboratory, Ann Harbor, Michigan). During the experiment, refractive index of the samples always matched the liquid (toluene) to avoid stray light. The Zeta (ξ) potential was automatically calculated from electrophoretic mobility based on the Smoluchowski equation (Equation Equation1(1) ):
(1)
where
is the measured electrophoretic velocity, η is the viscosity, ε is the electrical permittivity of the electrolytic solution and E is the electric field. The accuracy was 0.12 μm cm/V s for aqueous systems. Samples were suspended in distilled water and the measurements were recorded at 25 °C.
Determination of drug loading
The percentage of caffeine entrapped in the lipid matrix was determined as follows: a fixed amount of SLN dispersion was filtered using a Pellicon XL tangential ultrafiltration system (Millipore, Milan, Italy) equipped with a polyethersulfone Biomax 1000 membrane (Millipore, Milan, Italy) with a 1 000 000 Da molecular weight cut-off. An amount of retained material was freeze dried, dissolved in dichloromethane and analyzed by UV spectrophotometry at 273 nm (Lambda 52, PerkinElmer, MA). Calibration curves for the validated UV assays of caffeine were performed on six solutions in the concentration range 10–100 µg/ml. The correlation coefficient was >0.99.
Each point represented the average of three measurements, and the error was calculated as standard deviation (±SD). Caffeine incorporation efficiency was expressed as active recovery and calculated using Equation (Equation2(2) ):
(2)
Possible lipid interferences during UV determination of caffeine were also investigated by comparing the two standard curves of the substance alone and in the presence of lipids. The differences observed between the standard curves were within the experimental error, thus inferring that no lipid interference occurred (data not shown).
DSC analysis
DSC scans of materials, empty and SLN-CAF were performed on a Mettler DSC 12E equipped with a Haake thermocryostate model D8-G. A Mettler TA89E and FP89 system software was used for the data acquisition. Indium was used to calibrate the instrument. The reference pan was filled with 100 µl of distilled water or empty pan. The raw materials were scanned at a speed of 5 °C/min in 20 a 100 °C temperature range; free caffeine, empty, and SLN-CAF were scanned at the same rate speed in the range from 20 °C to 250 °C. Each experiment was carried out in triplicate.
In vitro studies
Gel preparation
The gel formulations were prepared dispersing xantan gum (1% w/w) in glycerol (10% w/w) with constant stirring, after that SLN suspension (89% w/w) was added to obtain the hydrogel. A reference gel (GEL-CAF), containing the same amount of caffeine, was prepared as well. All the formulations were stored at 4 °C before use.
Skin membrane preparation
Samples of adult human skin (mean age 36 ± 8 years) were obtained from breast reduction operations. Subcutaneous fat was carefully trimmed and the skin was immersed in distilled water at 60 ± 1 °C for 2 min (Kligman & Christophers, Citation1963), after which SCE (stratum corneum and epidermis) was removed from the dermis using a dull scalpel blade. Epidermal membranes were dried in a desiccator at ∼25% relative humidity.
The dried samples were wrapped in aluminum foil and stored at 4 ± 1 °C until use. A previous research work demonstrated the maintenance of SC barrier characteristics after storage in the reported conditions (Swarbrick et al., Citation1982). Besides, preliminary experiments were carried out in order to assess the barrier integrity of SCE samples by measuring the in vitro permeability of [3H] water through the membranes using the Franz cell method described below. The value of calculated permeability coefficient (Pm) for [3H] water agreed well with those previously reported (Bronaugh et al., Citation1986).
In vitro skin permeation experiments
Samples of dried SCE were rehydrated by immersion in distilled water at room temperature for 1 h before being mounted in Franz-type diffusion cells supplied by LGA (Berkeley, California). The exposed skin surface area was 0.75 cm2 and the receiver compartment volume was of 4.5 ml.
The receptor compartment was filled with a solution of phosphate buffer (pH 6.8), stirred at 500 rpm and thermostated at 32 ± 1 °C during all experiments (Puglia et al., Citation2005).
A weighed amount of each formulation (SLN-CAF and GEL-CAF) was placed on the skin surface in the donor compartment and the latter was covered with Parafilm® (Pechiney Plastic Packaging Company, Chicago, IL). Each experiment was run in duplicate for 24 h using three different donors (n = 6). At predetermined intervals, samples (200 µl) of receiving solution were withdrawn and replaced with fresh solution. The samples were analyzed for CAF content by HPLC as described below. The results were expressed as cumulative amount of CAF permeating the SCE membranes after 24 h. Statistical analysis of data was performed using Student’s t-test.
HPLC analyses
The HPLC apparatus consisted of a Shimadzu LC10 AT Vp (Milan, Italy) equipped with a 20-µl loop injector and a SPD-M 10 A Vp Shimadzu photodiode array UV detector. Chromatography was performed using a Symmetry Shield C18 RP column (particle size, 5 µm; 250 mm × 4.6 mm i.d.; Phenomenex, Torrance, CA). The mobile phase was composed of 20% water (pH 3 adjusted with phosphoric acid) and 80% methanol and the detection was effected at 273 nm. The flow rate was set at 1 ml/min. The retention time was 7.5 min.
Results and discussion
SLN characterization
SLN-CAF were prepared with Softisan 100 (Hydrogenated Coco-Glycerides) and Pluronic F68 (poloxamer 188) as solid lipid and surfactant, respectively. We decided to use these materials after a screening for the identification of lipid matrices for CAF incorporation. Particularly, we chose hydrogenated coco-glycerides to prepare lipid nanoparticles since they are characterized by a low melting point (35 °C), a feature that we consider important for the formulation of the nanocarriers by using the preparation method described in the present manuscript. The formulation strategy, in fact, gave interesting results as confirmed by PCS analyses and drug loading determination. The ambitious goal was to obtain nanoparticles characterized by valid dimensions and good encapsulation efficiency although the active to incorporate showed a hydrophilic character. In fact, whatever the preparation method, due to their hydrophobic nature, lipid matrices may be more appropriate to incorporate lipophilic drugs, which can be easily dissolved in melted lipids. Hydrophilic substances are expected to be poorly encapsulated in SLN, tending to partition in water phase during the formulation of these carriers. From the results obtained, loaded and unloaded SLN were in a nanometric range (182.6 ± 8.4 and 225.1 ± 7.4 nm, respectively) and showed an interesting payload value (75% ± 1.1).
Zeta potential (ZP) is an important parameter that allows predicting about SLN physical stability and in theory, higher ZP values, being positive or negative, tend to stabilize particle suspension. Usually, particle aggregation is less likely to occur for charged particles with pronounced ZP (>|20|), due to the electrostatic repulsion between particles with the same electrical charge (Gonzalez-Mira et al., Citation2010). The analyses registered a slow decrease in zeta potential values for caffeine loaded SLN (−32.3 ± 2.64 mV) with respect to unloaded SLN (−29.3 ± 1.35 mV) predicting a good long-term stability for both formulations.
To be certain that the calorimetric peak of the SLN could be ascribed to just a well-defined system, we submitted the vehicles and the single components used to prepare the SLN to DSC analysis. shows the differential scanning calorimeter (DSC) thermograms of the component of SLN and empty or SLN-CAF dispersions. CAF shows a sharp endotherm peak having a melting point at 235 °C, while the solid components, Softisan 100 and Pluronic F68, presented an endothermic peak at 39.2 °C and 56.3 °C, respectively. When the solid lipid was formulated as nanoparticles, the endothermic peak happened at higher temperature (55.7 °C). This increase of temperature together to the absence of CAF peak could suggest the drug incorporation into SLN.
In vitro study
In , the plots of the cumulative amounts of caffeine permeated through human SCE membranes as a function of time are shown.
Figure 2. Cumulative amount of caffeine permeated through SCE membranes from SLN-CAF (▪) and GEL-CAF (•) formulations.
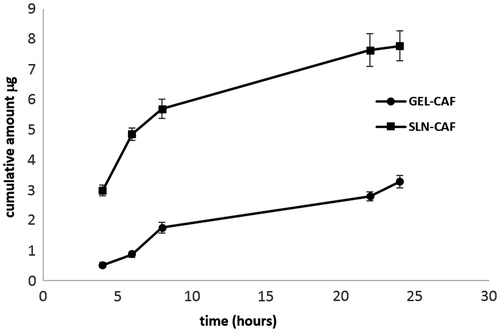
SLN-CAF generated a significantly faster permeation than GEL-CAF (p < 0.05) and the caffeine amount delivered in the receptor fluid from SLN-based formulation was always significantly higher compared to the gel formulation over 24 h.
Different strategies to optimize caffeine percutaneous absorption are reported in the literature. Bolzinger et al. (Citation2008), for instance, evaluated the effect of microemulsions on caffeine in vitro percutaneous absorption. The author observed a faster caffeine permeation with a microemulsion in comparison to a gel formulation containing the active compound. In order to explain the result, the researcher hypothesized a penetration enhancer effect of the surfactant, which could dissolve part of the interconeocyte lipids modifying the stratum corneum integrity.
Shakeel & Ramadan (Citation2010) formulated and characterized nanoemulsion-based forms containing caffeine. The authors evaluated the in vitro permeation profile of caffeine from these carriers observing a penetration enhancer effect that seems to be related to the carrier nanodimensions.
As regards the enhancer effect of lipid nanoparticles, it seems due to their occlusive character and to the consequent film formation after their application to the skin. Due to occlusion, water evaporation from the skin to the atmosphere is decreased and water is thus retained within the skin. The stratum corneum swells, which leads to a drug penetration enhancing effect (Jenning et al., Citation2000; Wissing et al., Citation2001; Loo et al., Citation2013).
This hypothesis is consistent with the results of other researchers and is useful to elucidate the role of lipid nanoparticles on in vivo skin delivery of active compounds, although it is not enough to explain the findings of our in vitro permeation study. In fact, we hypothesized that once applied to the stratum corneum the SLN, made of high biocompatible lipids, can fuse with stratum corneum lipids. This collapse of structure liberates permeant into a medium in which the active is poorly soluble. In these conditions, an increase of caffeine thermodynamic activity is observed so facilitating the delivery.
Küchler et al. (Citation2009) are in agreement with this explanation and pointed out the main role of the lipid matrix of SLN to promote the skin penetration of hydrophilic drugs. Besides, the author stated that both size of nanocarriers and surfactant used for SLN stabilization appeared of subsidiary relevance for the enhancement of drug skin permeation.
These preliminary results gave us important indication regarding the effect of lipid nanocarriers on caffeine percutaneous absorption, but are not enough to deeply understand the role of these carriers in determining caffeine dermal or transdermal fate. At this aim, in vivo studies are in progress to elucidate the mechanism involving caffeine percutaneous absorption from SLN-CAF formulation.
Conclusions
SLN employed in our study are endowed with a good encapsulation efficacy and with a well-determined size distribution. DSC studies performed on lipid nanoparticles showed that caffeine was incorporated into solid lipid matrix. In vitro percutaneous absorption studies showed that SLN could be a valid tool to optimize the topical delivery of caffeine.
Declaration of interest
The authors report no declaration of interest.
References
- Aggarwal N, Goindi S. (2013). Preparation and in vivo evaluation of solid lipid nanoparticles of grise of ulvin for dermal use. J Biomed Nanotechnol 9:564–76
- Bolzinger MA, Briançon S, Pelletier J, et al. (2008). Percutaneous release of caffeine from microemulsion, emulsion and gel dosage forms. Eur J Pharm Biopharm 68:446–51
- Bronaugh RL, Stewart RF, Simon M. (1986). Methods for in vitro percutaneous absorption studies VII: use of excised human skin. J Pharm Sci 75:1094–7
- Dias M, Farinha A, Faustino E, et al. (1999). Topical delivery of caffeine from some commercial formulations. Int J Pharm 182:41–7
- Gallarate M, Trotta M, Battaglia L, Chirio D. (2009). Preparation of solid lipid nanoparticles from W/O/W emulsions: preliminary studies on insulin encapsulation. J Microencapsul 26:394–402
- Gee CM, Nicolazzo JA, Watkinson AC, Finnin BC. (2012). Assessment of the lateral diffusion and penetration of topically applied drugs in humans using a novel concentric tape stripping design. Pharm Res 29:2035–46
- Gonzalez-Mira E, Egea MA, Garcia ML, Souto EB. (2010). Design and ocular tolerance of flurbiprofen loaded ultrasound-engineered NLC. Colloids Surf B Biointerfaces 81:412–21
- Hu FQ, Hong Y, Yuan H. (2004). Preparation and characterization of solid lipid nanoparticles containing peptide. Int J Pharm 273:29–35
- Jenning V, Gysler A, Schafer-Korting M, Gohla SH. (2000). Vitamin A loaded solid lipid nanoparticles for topical use: occlusive properties and drug targeting to the upper skin. Eur J Pharm Biopharm 49:211–8
- Kang KC, Jeong NH, Lee CI, Pyo HB. (2009). Preparation and characterization of SLNs (W/O/W type) contained lipoic acid PEG ester by variation lipid. J Ind Eng Chem 15:529–36
- Kawashima Y, Niwa T, Handa T, et al. (1989). Preparation of prolonged-release spherical micro-matrix of ibuprofen with acrylic polymer by the emulsion-solvent diffusion method for improving bioavailability. Chem Pharm Bull 37:425–9
- Kligman AM, Christophers E. (1963). Preparation of isolated sheets of human stratum corneum. Arch Dermatol 88:702–5
- Koo SW, Hirakawa S, Fujii S, et al. (2007). Protection from photodamage by topical application of caffeine after ultraviolet irradiation. Br J Dermatol 156:957–64
- Küchler S, Abdel-Mottaleb M, Lamprecht A, et al. (2009). Influence of nanocarrier type and size on skin delivery of hydrophilic agents. Int J Pharm 377:169–72
- Loo CH, Basri M, Ismail R, et al. (2013). Effect of compositions in nanostructured lipid carriers (NLC) on skin hydration and occlusion. Int J Nanomedicine 8:13–22
- Lu YP, Lou YR, Xie JG, et al. (2007). Caffeine and caffeine sodium benzoate have a sunscreen effect, enhance UVB-induced apoptosis, and inhibit UVB-induced skin carcinogenesis in SKH-1 mice. Carcinogenesis 28:199–206
- Mitri K, Shegokar R, Gohla S, et al. (2011). Lipid nanocarriers for dermal delivery of lutein: preparation, characterization, stability and performance. Int J Pharm 414:267–75
- Müller RH, Radtke M, Wissing SA. (2002). Solid lipid nanoparticles (SLN) and nanostructured lipid carriers (NLC) in cosmetic and dermatological preparations. Adv Drug Deliv Rev 54:S131–55
- Puglia C, Blasi P, Rizza L, et al. (2008). Lipid nanoparticles for prolonged topical delivery: an in vitro and in vivo investigation. Int J Pharm 357:295–304
- Puglia C, Bonina F. (2012). Lipid nanoparticles as novel delivery systems for cosmetics and dermal pharmaceuticals. Expert Opin Drug Del 9:429–41
- Puglia C, Tropea S, Rizza L, et al. (2005). In vitro percutaneous absorption studies and in vivo evaluation of anti-inflammatory activity of essential fatty acids (EFA) from fish oil extracts. Int J Pharm 299:41–8
- Ricci M, Puglia C, Bonina F, et al. (2005). Evaluation of indomethacin percutaneous absorption from nanostructured lipid carriers (NLC): in vitro and in vivo studies. J Pharm Sci 94:1149–59
- Roure R, Oddos T, Rossi A, et al. (2011). Evaluation of the efficacy of a topical cosmetic slimming product combining tetrahydroxypropyl ethylenediamine, caffeine, carnitine, forskolin and retinol, in vitro, ex vivo and in vivo studies. Int J Cosmet Sci 33:519–26
- Shakeel F, Ramadan W. (2010). Transdermal delivery of anticancer drug caffeine from water-in-oil nanoemulsions. Colloids Surf B Biointerfaces 75:356–62
- Swarbrick J, Lee G, Brom J. (1982). Drug permeation through human skin. I. Effects of storage conditions of skin. J Invest Dermatol 78:63–6
- Trotta M, Cavalli R, Carlotti ME, et al. (2005). Solid lipid micro-particles carrying insulin formed by solvent-in-water emulsion-diffusion technique. Int J Pharm 288:281–8
- Wissing SA, Lippacher A, Muller RH. (2001). Investigations on the occlusive properties of solid lipid nanoparticles (SLN). J Cosmet Sci 52:313–24
- Yamamura J, Koyasu M, Sato H, et al. (1996). Topical treatment of cutaneous herpes simplex virus-1 infection in mice with a specially formulated caffeine gel (Cafon). J Dermatol Sci 12:50–5