Abstract
Bexarotene (Bex) is a synthetic retinoid that exhibits anti-tumor activities. However, the low solubility of the compound hinders its development. In this study, bexarotene nanocrystal was developed and its surface was modified to improve the dissolution and absorption of the drug. The nanocrystals were prepared via precipitation, high-pressure homogenization method, and modified by folate-chitosan (FA-CS), which relied on the charge interaction between the negatively charged nanocrystals and the positively charged FA-CS. The physical–chemical properties in terms of particle size, size distribution, zeta potential, morphology and crystallinity were evaluated. The results showed that bexarotene nanocrystals with surface modification by folate–chitosan conjugates (FC–NC–Bex) with a mean particle size of 631.3 ± 2.7 nm, a polydispersity index of 0.33 ± 0.06 and a zeta potential of 24.6 ± 1.9 mV was obtained. The result of differential scanning calorimetry (DSC) showed that the nanocrystals were still in crystalline state after the preparation procedure. In the in vitro dissolution test, FC-NC-Bex showed significant increase in dissolution rate compared to raw bexarotene (nearly 6.5-fold). Compared to bexarotene suspension, FC-NC-Bex exhibited significant increase in AUC0–∞ (approximately 3-fold) and Cmax (about 1.5-fold). Taken together, the results suggested that FC-NC-Bex may provide a potential opportunity in enhancing the dissolution rate of bexarotene and its gastrointestinal absorption.
Introduction
Bexarotene is a synthetic retinoid that selectively activates the retinoid X receptor subfamily of retinoid receptors (Mehta et al., Citation2012). It exhibits anti-tumor activities by inhibiting cell growth, inducing differentiation and promoting apoptosis in a variety of tumor cell lines (Malik et al., Citation2011). Currently, bexarotene is clinically used in the treatment of cutaneous T-cell lymphoma (Hermann et al., Citation2005). It also shows promising inhibitory effect against non-small cell lung cancer (NSCLC) (Yen, Citation2004; Zhang et al., Citation2010; Mehta et al., Citation2012), ER-negative breast cancer (Li et al., Citation2008) and prostate cancer. Unfortunately, the low solubility (at saturation) and slow dissolution velocity of bexarotene has led to poor bioavailability and greatly limited its pharmaceutical development and clinical utilization.
During the past decades, nanocrystals have emerged as an important delivery system for overcoming the solubility problems of many drugs (Sun & Yeo, Citation2012). Nanocrystals are a submicron colloidal dispersion of drug particles that are stabilized by surfactants, polymers or a mixture of both (Pardeike et al., Citation2011). There are many advantages of this system (Pardeike & Müller, Citation2010). Owing to the increased surface-to-volume ratio of the nanocrystals, an increase in saturated solubility and very fast dissolution rate can be seen, especially with those with particle sizes of 1 μm, thus increasing their bioavailability after oral administration (Kocbek et al., Citation2006; Zhang et al., Citation2011; Gao et al., Citation2012). So far, many representative nanocrystals of water-insoluble drugs have been approved for use or for clinical trials, such as sirolimus (Rapamune®, Wyeth Pharmaceuticals Inc., Philadelphia, PA), fenofibrate (Tricor®, Abbott Laboratories, Chicago, IL), megestrol acetate (Megace ES®, Par Pharmaceuticals Inc., Spring Valley, NY). Obviously, nanocrystals have emerged as a promising strategy for the formulation of poorly soluble drugs.
In order to selectively deliver a drug to tumor tissues, active targeting through tumor-specific ligand/receptor interaction has been widely investigated (Paulos et al., Citation2004; Mansouri et al., Citation2006). An effective approach for improving the targeting capability is to modify the nanocrystals with chemical or biological reagents including antibodies or low molecular weight targeting agents, such as folic acid (FA) (Quan et al., Citation2012). Folate receptors are known to be overexpressed in the tissues of several human tumors, including ovarian, breast, liver, kidney, uterus, testis, brain, colon and lung cancers, but are rarely found on the surface of normal cells or localized to the apical surfaces of polarized epithelia (Morris & Sharma, Citation2010; Zhu et al., Citation2011). FA and FA conjugates are generally recognized as effective tumor targeting agents because of their specific binding with the folate receptors (Lu & Low, Citation2012). FA conjugates, which are covalently derivatized via their γ-carboxyl moiety, can retain the high-affinity ligand binding property of FA, and can enter cells by receptor-mediated endocytosis (Wan et al., Citation2008). Thus, folate-based candidates have been widely used in drug targeting-delivery systems.
The aim of this study was to prepare and characterize bexarotene nanocrystals containing surface modification. The surfaces of these nanocrystals were conjugated to FA–CS to enhance their solubility, dissolution rate and oral bioavailability. The characteristics of these surface-modified bexarotene nanocrystals were investigated and their release and subsequent bioavailability were evaluated in vitro and in vivo.
Materials and methods
Materials
Bexarotene and the internal standard () were synthesized by Shenyang Pharmaceutical University and were kindly donated by Professor Chen. F68 was obtained from Sigma (NJ). Soybean lecithin was purchased from Shanghai Taiwei Medicine Co. Ltd (Shanghai, China). Mannitol (HPLC grade) was obtained from Tianjin Siyou Fine Chemicals Co. Ltd (Tianjin, China). Chitosan (deacetylation degree >90.0%) was provided by Zhejiang Aoxing Biotechnology Co. Ltd (Zhejiang, China). FA was supplied by Hubei Henglvyuang Technology Co. Ltd (Hubei, China). 1-Ethyl-3-(3-dimethyllaminopropyl) carbodiimide hydrochloride (EDC.HCI) and N-hydroxysuccinimide (NHS) were purchased from Suzhou Highfine Biotech Co. Ltd (Suzhou, China). Acetonitrile (HPLC grade) was bought from Grace Company Inc (MD).
Methods
Synthesis of FA–CS conjugates
Unless otherwise stated, all the reactions were carried out in the dark to prevent the decomposition of FA. The synthesis of FA–CS conjugates is schematically shown in . Firstly, CS was dissolved in acetic acid solution. A solution containing a mixture of FA, NHS and EDC was then prepared by simultaneously dissolving the solid compounds in anhydrous DMSO and triethylamine (TEA). FA was activated by EDC and NHS under anhydrous conditions and in the presence of nitrogen for 2 h at room temperature. After that, the CS solution was added dropwise to the FA–NHS–EDC mixture with stirring at different reaction temperatures. It was then adjusted to pH 9.0 with diluted sodium hydroxide solution. The coagulation was purified by dialyzing against phosphate buffer (PBS, pH 7.4) for three days followed by dialysis against double-distilled water for another three days. Finally, yellow colored products (FA–CS) were collected and freeze-dried at −70 °C for 24 h.
Quantitative analysis of FA to CS coupling ratio
In order to determine the content of FA in the FA–CS conjugates, FA–CS was dissolved in 2% (v/v) acetic acid solution. To determine the content of FA in the FA–CS conjugate, the absorbance of the FA–CS conjugate was measured by a UV–Vis spectrophotometer (Shimadzu, Kyoto, Japan) at 363 nm and the FA content was calculated by reference to a standard calibration curve. The coupling ratio (CR) was obtained:
where WFA–CS was the total amount of FA–CS conjugates and WFA was the amount of FA in the FA–CS conjugate.
Characterization of FA–CS conjugates
FT-IR spectrum (GX-1, PerkinElmer, Waltham, MA) was obtained by scanning samples from 4000 to 400 cm−1. 1H-NMR spectrum was measured on a spectrometer (400MHZ Advance, Bruker, Berlin, Germany) using deuterated DMSO as a solvent for FA and deuterated hydrochloric acid (containing 10% deuterated DMSO) for FA–CS conjugates.
Preparation of bexarotene nanocrystals
Bexarotene nanocrystals were prepared using a tandem precipitation – high-pressure homogenization process. Briefly, bexarotene was dissolved in 2 mL ethanol, and the solution was then dispersed in a double-distilled water solution containing mixed stabilizers, with stirring for 30 min at 4 °C. The mixture was successively homogenized with 5 cycles at 7000 psi and 20 cycles at 16 000 psi in a Microfluidizer processor (Microfluidics, Westwood, MA). The samples were centrifuged at 12 000 g for 30 min at 4 °C to collect the bexarotene nanocrystals.
Preparation of FC–NC–Bex
The bexarotene nanocrystals were dispersed into 50 mL FA–CS solutions and vortexed for 20 min. The sample was then centrifuged at 12 000 g for 30 min at 4 °C to remove the free FA–CS conjugates. For long-term storage stability and convenient transportation, 5% (W/V) mannitol was added to the nanocrystals as a cryoprotectant and the mixture was rapidly cooled down to −80 °C in an Ultralow Temperature Freezer (900series, Thermo, Madison, WI) for 24 h and then freeze-dried for 48 h at −70 °C in a FD-1C-80 freeze dryer (Beijing Bo Yi Kang Experimental Instrument Co. Ltd., Beijing, China).
Characterization of FC–NC–Bex
Size and zeta potential
The particle size and the zeta potential were determined using a Zetasizer Nano ZS (Malvern, UK). Bexarotene nanocrystals were diluted with double-distilled water. All data were presented as the mean values of three independent samples produced under identical production conditions. The particle size of each sample was measured in triplicate at room temperature.
Morphology observation
Morphological evaluation of the nanocrystals was carried out by transmission electron microscopy (TEM) (H-7000, Hitachi, Tokyo, Japan). The freeze-dried powder was dissolved in double-distilled water to obtain a moderate concentration before measurement. Then one droplet of the sample was placed on a 200-mesh copper grid and stained with 2% (w/v) phosphotungstic acid for 20 s, dried at room temperature and then examined by TEM. The surface morphology of the nanocrystals was investigated by scanning electron microscopy (SEM) (S-4800, Hitachi, Tokyo, Japan).
Differential scanning calorimetry characterization
The phase transition of bexarotene crystals was analyzed by differential scanning calorimetry (DSC) (Mettler Toledo, Jiangsu, China) at a heating rate of 10 °C/min from 25 to 400 °C. This was done under dry nitrogen atmosphere and Al2O3 was used as a reference.
Saturation solubility
In order to assess whether the solubility of bexarotene at saturation was enhanced after nano-sizing, the solubility experiment was conducted with a shaker. Excess bexarotene and lyophilized FC–NC–Bex powder, as well as the physical mixture were dispersed in 10 mL 0.02% (v/v) Tween 80 aqueous solution, respectively. The temperature and stirring rate were set at 37 ± 0.5 °C and 100 rpm. After stirring for 72 h, 2 mL sample was withdrawn and centrifuged at 12 000 g for 10 min with TGL-20 M high-speed centrifuge (Hunan Xiangyi Centrifuge Instruments Co. Ltd. Hunan, China), then the supernatants were filtered through 0.22 μm microporous membrane filters before subjecting to HPLC analysis. The experiment was carried out in triplicate.
Dissolution evaluations
Three groups including bexarotene, physical mixture and FC–NC–Bex were all studied. Dissolution testing of the samples containing bexarotene (2 mg) was performed with the paddle method. The rotation speed of the paddle was set at 100 rpm. At 37 ± 0.5 °C, 350 mL of 0.02% (v/v) Tween 80 aqueous solution was used as the dissolution medium. Samples (2 mL) were withdrawn at specific time intervals and filtered immediately, and the amount of dissolved drug in filtrates was determined by HPLC.
In vivo pharmacokinetics study
Animals
Six- to eight-week-old male SD rats (weighing 250 ± 20 g) were used in all experiments. The animals were supplied by the Experimental Animal Center of Shenyang Pharmaceutical University (Shenyang, China). All rats were acclimatized at a temperature of 25 ± 2 °C and housed in cages for at least 3 days with free access to food and water. Prior to drug administration, the animals were fasted for 12 h, but still had access to water. All experimental procedures abided by the guidelines set out by the Animal Protection and Ethics Committee. Animal discomfort and the total number of animals used were kept to a minimum during the experiment.
Experimental design
FC–NC1–Bex was dispersed in normal saline and then administered to the rats. Bexarotene was suspended in 0.3% (w/v) CMC-Na solution and used as a control. The rats were randomly divided into two groups (five rats in each group). For oral administration, two groups received gavage administration of the bexarotene suspensions or the FC–NC–Bex suspension at a 60.0 mg/kg dose level. Blood (0.25 mL) was sampled from post-orbital vein at the following times: 0.25, 0.5, 1, 2, 3, 4, 6, 8, 12 and 24 h. Plasma was immediately separated by centrifugation (4000 g, 10 min) and stored at −20 °C until analysis.
Plasma sample analysis
A 100 μL of rat plasma was mixed with 20 μL internal standard solution (40 μg/mL) by vortexing for 20 s, followed by an addition of 100 μL acetonitrile and 20 μL 0.5 M hydrochloric acid, with vortexing between addition. Subsequently, the sample was extracted with 400 μL mixed organic solvent (n-pentane and isoamyl alcohol, 98:2, V/V) and vortexed for 10 min. After centrifugation at 4500 g for 15 min, the organic layer was transferred to another tube and evaporated under nitrogen gas at 40 °C. The residue was dissolved in 100 μL acetonitrile by vortexing for 4 min and ultrasonication for 2 min. After that, 20 μL of the sample was injected into the HPLC for analysis.
The concentration of bexarotene in the plasma was determined by an Agilent 1200 HPLC analysis system (Agilent, Santa Clara, CA) using a Welch Materials C18 column (5 μm, 4.6 mm × 250 mm) and a mobile phase consisting of a mixture of acetonitrile/buffer (10 mM ammonium acetate/acetic acid, 1:0.01, V/V) 87: 13. The column temperature was set at 30 °C and the flow rate was set at 1.0 mL/min. The eluent was monitored by UV absorbance at 260 nm.
Data analysis
Statistical analysis was performed using Student’s t test and significance was considered at the p < 0.05 level. Pharmacokinetic parameters were obtained by the computer program Drug and Statistics (DAS) version 2.0 (Mathematical Pharmacology Professional Committee of China, Shanghai, China).
Results and discussion
Synthesis of FA–CS conjugates
The coupling of FA to CS produced FA–CS conjugates with new and improved functions because the original properties of CS were maintained and the targeting ligand of FA was incorporated (Li et al., Citation2011). The carboxyl group of FA reacted with the amino groups of chitosan following the usual NHS/EDC reaction to form FA–CS conjugates via amide bond. The γ-COOH of FA is more prone to this reaction due to its higher reactivity although FA has two –COOH groups (Zhang et al., Citation2007). The final product FA–CS was synthesized via the reaction between the activated FA ester and the primary amine groups of CS, which resulted in the formation of amide bond under homogeneous conditions. Furthermore, the FA to CS molar ratio, reaction time and reaction temperature were the most important factors that affected the conjugation of FA to CS molecules (Ji et al., Citation2011; Li et al., Citation2011). Taking the FA to CS coupling ratio as an index, the L9 (33) orthogonal experimental design was used to produce the experimental results as shown in , which indicates A3B3C2 (FA to CS molar ratio of 2:1, reaction time of 24 h and reaction temperature of 40 °C) as the optimized formulation for the conjugation of FA to CS. Increases in FA to CS molar ratio and reaction time led to a higher degree of substitution, while CR decreased when the reaction temperature was increased to 45 °C. This could be due to the fact that higher temperature resulted in the degradation of CS and decomposition of FA.
Table 1. Design matrix based on L9 orthogonal array in synthesis experiments of FA–CS conjugates.
FT-IR analysis of FA–CS conjugates
Significant changes in the FT-IR spectrum of FA–CS compared to the FT-IR spectrum of CS confirmed the presence of FA–CS conjugates (). Four characteristic peaks were detected for the FT-IR spectrum of FA-CS. The peaks at 3415, 1694 and 1606 cm−1 were caused by the vibration of N–H, C = O, amino group in the pteridine ring, respectively, whereas the peak at 1485 cm−1 might be caused by C = C or C = N of FA. In the case of CS spectrum, the absorption peaks at 1631 and 1596 cm−1 were attributed to the vibration of amide I and amide II groups. In addition, the wide absorption band at 3442 cm−1 represented the vibration of the OH group and the strong absorption peaks at 1079 cm−1 belonged to the vibration of C–O–C. Significant difference was observed between the IR spectra of CS and FA–CS. It could be seen that the absorption peak at 3402 cm−1 became stronger due to the overlapping vibration of OH and N–H group. The FT-IR spectrum of FA-CS illustrated that the absorption at 1596 cm−1 disappeared and the peak appeared at 1605 cm−1 was likely derived from chitosan. Moreover, this peak also became stronger suggesting that some of the amino groups in chitosan might have been substituted (Aoki et al., Citation2003). Therefore, it was supposed that the NH2 groups in CS were partly converted into NH groups.
1H-NMR analysis of FA–CS conjugates
It was apparent that the FA–CS spectrum contained signals originating from both FA and FA–CS. As shown in the 1H-NMR spectrum of FA (), the signal at 11.47 ppm corresponds to the protons of H-20/23, the signal at 8.65 ppm corresponds to the proton at the 7-position of the pterin ring, and the signals at 8.13, 7.67, 6.92, 6.66, 4.50, 4.35, 2.52 and 2.02–2.04 ppm correspond to the protons of H-18, H-13/15, H-10, H-12/16, H-9, H-19, H-22 and H-21, respectively. The coupling of FA to CS resulted that in the overlapping of some sympathetic vibration peaks (3.46–4.29 ppm) due to the influence of solvent and the interaction between FA and CS. Coupling of FA to CS was confirmed by the appearance of the peculiar signals at 7.05 and 6.13 ppm, which were attributed to the aromatic protons of FA. In addition, the characteristic peaks of FA at 2.62 ppm (H-22), 2.16 ppm (H-21) and the characteristic peaks of CS at 3.63 ppm (H-5′), 3.02 ppm (H-2′), 1.97 ppm (H-4′) were still observed. The appearance of characteristic peaks in the 1H-NMR spectrum of FA–CS conjugates confirmed that FA was successfully conjugated to CS.
Formulation optimization of bexarotene nanocrystals
The choice of stabilizer is very important in the preparation of nanocrystals. The stabilizer (or mixture of stabilizers) should exhibit sufficient affinity for the droplet surface in order to stabilize the nanocrystals. In the preliminary experiments, F68, lecithin and HPMC were used as stabilizers to screen for the optimal formulation for bexarotene taking into consideration the zeta potential and storage stability. Thus, it is critical to identify the types and concentrations of stabilizers in order to obtain the optimal formulation composition with long-term storage stability (Sun et al., Citation2012). The zeta potential of the nanocrystals allows for the predictions of the storage stability of colloidal dispersions. According to the report, a zeta potential of about ± 20 mV can illustrate the long-term stability of the system when non-ionic surfactants are applied (Hao et al., Citation2012; Kakran et al., Citation2012). In this study, eight formulations () were investigated by precipitation–high pressure homogenization method. The zeta potential of formulas 2, 3, 5, 6, 7, 8 were between −20 mV and −30 mV. Moreover, after storing at 4 °C for 1 week, formulas 5, 6, 8 showed better short-term stability. However, in order to avoid possible side effects such as anaphylactic response and vascular irritability caused by surfactants, it would be safer to reduce the types and amounts of stabilizers used to some extent. Thus, formula 5 was selected as the optimized preparation, with the particle size and zeta potential of about 403.8 ± 4.4 nm and −23.4 ± 1.6 mV, respectively.
Table 2. Formulation composition of bexarotene nanocrystals.
Characterization of FC–NC–Bex
An attempt was made to modify the surface of the bexarotene nanocrystals to make them more selective in targeting to specific site in body. The modification was carried out by physical adsorption of three different concentrations of FA–CS (0.05, 0.1 and 0.2%, w/w) to bexarotene nanocrystals. It could be seen that the mean particle size increased from 501.1 ± 4.1 to 645.5 ± 3.9 nm when the concentration of FA–CS was increased from 0.05 to 0.1%. Nevertheless, when the concentration of FA–CS was increased to 0.2%, there was no significant increase in the mean particle size (631.3 ± 2.7 nm). Therefore, the 0.2% of FA–CS was selected as the optimal formulation.
The zeta-potential increased with the increasing concentration of FA–CS. The resulting positive charge of the surface of the bexarotene nanoparticles confirmed the deposition of the positively charged FA–CS on the surface of the negatively charged nanocrystals. The zeta-potential of 0.2% FA–CS was 24.6 ± 1.9 mV, indicating the surface-modified nanocrystals yielded a stable preparation.
DSC analysis
To characterize the freeze-dried FC–NC–Bex, DSC analysis of bexarotene, physical mixture and FC–NC–Bex were performed (). A single endothermic peak at 223.07 °C was observed for the DSC curve of bexarotene. This characteristic peaks were also present in the DSC curves of FC–NC–Bex as well as that of the physical mixture, meaning that the initial crystalline form of the drug was retained. Nevertheless, the peak of bexarotene in physical mixture and FC–NC–Bex showed a slight shift. This could be explained by the interaction of the excipients of F68, lecithin and mannitol with bexarotene. In addition, the peak position and intensity of bexarotene in physical mixture and FC–NC–Bex may appear deviation from raw bexarotene under the influence of heating rate and the amount.
Morphology observation by TEM
The morphology of surface FC–NC–Bex was analyzed by TEM. The combined method of precipitation and homogenization could achieve reproducible nano-sized particles with a narrow distribution. FC–NC–Bex was observed with small and uniform particle size of about 631.3 ± 2.7 nm for the surface-modified bexarotene nanocrystals, which appeared as spherical and did not adhere to each other (). Owing to the uniformity of this system, there was little tendency of aggregation or adhesion among the particles.
Morphology observation by SEM
The morphology of FC–NC–Bex and bexarotene are shown in . Under SEM observation, FC–NC–Bex appeared as flaky with small and irregular sizes. However, the particles from the bulk powder appeared much larger (microns), with irregular shapes, and composed mostly of fragmented drug crystals.
Solubility study
The solubility of the raw bexarotene, physical mixture and FC–NC–Bex were about 1.59, 4.80 and 18.65 µg/mL in 0.02% Tween-80 solution, respectively. Compared to the coarse bexarotene, the solubility of FC–NC–Bex was improved significantly. Moreover, the solubility of the physical mixture was also increased, which could be ascribed to the presence of surfactants. However, the markedly increased solubility of FC–NC–Bex at saturation was mainly attributed to smaller particle size of FC–NC–Bex. In general, if the particle size of a drug is reduced to the nanometer range, the saturation solubility of the drug would increase significantly. The results are explained by the Freundlich-Ostwald equation.
Dissolution experiments in vitro
In order to assess whether the goal of improving the dissolution rate of bexarotene was achieved, the in vitro dissolution profiles of different bexarotene samples were determined. The dissolution profiles of FC–NC–Bex, physical mixture and raw powder are shown in . The dissolution rate of the raw powder was very low: only 13% of the drug was dissolved in 0.02% Tween-80 within 10 min. A slight higher dissolution was obtained for the physical mixture, which may be attributed to the large amount of soluble excipients wrapped around the spherical particle. However, the dissolution rate of FC–NC–Bex was significantly enhanced, with 84% of the drug dissolved within 10 min, and complete dissolution occurred almost within 20 min. Enhancement in the dissolution rate of FC–NC–Bex was primarily attributed to the enlarged surface area and the decreased diffusion layer thickness.
Pharmacokinetic studies in vivo
To confirm the advantage of nanocrystals in improving the bioavailability of bexarotene, the in vivo test was carried out in rats and pharmacokinetic parameters of bexarotene were compared. The plasma concentration–time profiles of bexarotene suspension and FC–NC–Bex were shown in and the corresponding pharmacokinetic parameters were displayed in . It was clear from the data that the suspension group and the nanocrystals group were significantly different from each other in the corresponding parameters. Compared to rats treated with Bex-suspension, rats treated with FC–NC–Bex showed higher drug concentration all time points, especially at the initial time points. Both groups had similar Tmax (h) within the first 2 h of drug administration. The AUC0–∞ of Bex-suspension and FC–NC–Bex were 4.30 and 112.95 μg/mL·h, respectively. The higher AUC of FC–NC–Bex indicated significant increase in oral bioavailability of bexarotene. It is a biopharmaceutical classification system Class II substance (poorly soluble and highly permeable), which means that its dissolution is the rate-limiting step for absorption (Chen et al., Citation2013). The dissolution profiles suggested that nanocrystal drugs would have a higher dissolution rate than the coarse drug. Since nanocrystal drugs have much more surfaces under the same condition, they could easily dissolve in the intestinal fluid, and hence, smaller particles would tend to show a higher extent of uptake via both follicle-associated epithelia and absorptive enterocytes than larger particles (Guo et al., Citation2013). The results of in vivo pharmacokinetic studies indicated that nanocrystals of bexarotene may indeed provide a practical and effective approach to increase the oral bioavailability of bexarotene, and the excipients wrapped around the drug particles may further increase its bioavailability (Wu et al., Citation2011).
Figure 9. The mean plasma concentration–time curves of bexarotene in rats after oral administration (60 mg/kg) of Bex-suspension and FC–NC–Bex, the data were presented as mean ± S.D. (n = 5).
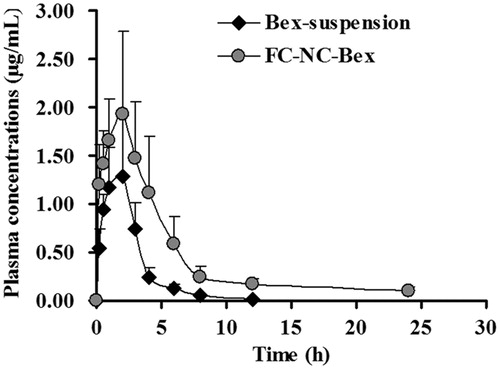
Table 3. Mean pharmacokinetic parameters of bexarotene after oral administration of Bex-suspension and FC–NC–Bex, mean ± S.D., n = 5.
Conclusion
In this study, bexarotene nanocrystals with surface modification were successfully prepared by conjugate with folate-chitosan. The morphology of surface-modified bexarotene nanocrystals was analyzed by TEM and SEM, which revealed a spherical-shape with non-self-adherent property. The crystalline state responsible for long-term stability was not altered according to DSC analysis. Significant increase in dissolution rate and oral bioavailability were obtained by reducing the particle size of bexarotene to the nanometer range. The effectiveness of this surface-modified bexarotene nanocrystal against tumor tissues will be a subject of future research.
Acknowledgements
The authors gratefully acknowledge the support provided by Department of Pharmaceutics, School of Pharmaceutical Sciences, Shandong University, China. We would like to thank Shenyang Pharmaceutical University, China for their support in animal experiments and bexarotene synthesis study. We also thank Dr. Alan K. Chang for helpful discussion and for revising the language of the manuscript.
Declaration of interest
The authors report no conflicts of interest. The authors alone are responsible for the content and writing of the paper.
This work was supported by the Liaoning Provincial Science and Technology Program of China, No. 2013305003.
References
- Aoki N, Nishikawa M, Hattori K. (2003). Synthesis of chitosan derivatives bearing cyclodextrin and adsorption of p-nonylphenol and bisphenol A. Carbohydr Polym 52:219–23
- Chen LJ, Wang YJ, Zhang JZ, et al. (2013). Bexarotene nanocrystal – oral and parenteral formulation development, characterization and pharmacokinetic evaluation. Eur J Pharm Biopharm; in press
- Gao L, Liu G, Ma JL, et al. (2012). Drug nanocrystals: in vivo performances. J Control Release 160:418–30
- Guo JJ, Yue PF, Lv JL, et al. (2013). Development and in vivo/in vitro evaluation of novel herpetrione nanosuspension. Int J Pharm 441:227–33
- Hao LL, Wang XY, Zhang DR, et al. (2012). Studies on the preparation, characterization and pharmacokinetics of Amoitone B nanocrystals. Int J Pharm 433:157–64
- Hermann TW, Yen WC, Tooker P, et al. (2005). The retinoid X receptor agonist bexarotene (Targretin) synergistically enhances the growth inhibitory activity of cytotoxic drugs in non-small cell lung cancer cells. Lung Cancer 50:9–18
- Ji JG, Wu DJ, Liu L, et al. (2011). Preparation, characterization, and in vitro release of folic acid-conjugated chitosan nanoparticles loaded with methotrexate for targeted delivery. Polym Bull 68:1707–20
- Kakran M, Shegokar R, Sahoo NG, et al. (2012). Fabrication of quercetin nanocrystals: comparison of different methods. Eur J Pharm Biopharm 80:113–21
- Kocbek P, Baumgartner S, Kristl J. (2006). Preparation and evaluation of nanosuspensions for enhancing the dissolution of poorly soluble drugs. Int J Pharm 312:179–86
- Li PW, Wang YC, Zeng FB, et al. (2011). Synthesis and characterization of folate conjugated chitosan and cellular uptake of its nanoparticles in HT-29 cells. Carbohydr Res 346:801–6
- Li Y, Zhang Y, Hill J, et al. (2008). The rexinoid, bexarotene, prevents the development of premalignant lesions in MMTV-erbB2 mice. Br J Cancer 98:1380–8
- Lu YJ, Low PS. (2012). Folate-mediated delivery of macromolecular anticancer therapeutic agents. Adv Drug Deliv Rev 64:342–52
- Malik SM, Collins B, Pishvaian M, et al. (2011). A phase I trial of bexarotene in combination with docetaxel in patients with advanced solid tumors. Clin Lung Cancer 12:231–6
- Mansouri S, Cuie Y, Winnik F, et al. (2006). Characterization of folate-chitosan-DNA nanoparticles for gene therapy. Biomaterials 27:2060–5
- Mehta N, Wayne AS, Kim YH, et al. (2012). Bexarotene is active against subcutaneous panniculitis-like T-cell lymphoma in adult and pediatric populations. Clin Lymphoma Myeloma Leukemia 12:20–5
- Morris VB, Sharma CP. (2010). Folate mediated in vitro targeting of depolymerised trimethylated chitosan having arginine functionality. J Colloid Interface Sci 348:360–8
- Pardeike J, Müller RH. (2010). Nanosuspensions: a promising formulation for the new phospholipase A2 inhibitor PX-18. Int J Pharm 391:322–9
- Pardeike J, Strohmeier DM, Schrödl N, et al. (2011). Nanosuspensions as advanced printing ink for accurate dosing of poorly soluble drugs in personalized medicines. Int J Pharm 420:93–100
- Paulos CM, Turk MJ, Breur GJ, Low PS. (2004). Folate receptor-mediated targeting of therapeutic and imaging agents to activated macrophages in rheumatoid arthritis. Adv Drug Deliv Rev 56:1205–17
- Quan P, Shi K, Piao HZ, et al. (2012). A novel surface modified nitrendipine nanocrystals with enhancement of bioavailability and stability. Int J Pharm 430:366–71
- Sun B, Yeo Y. (2012). Nanocrystals for the parenteral delivery of poorly water-soluble drugs. Curr Opin Solid State Mater Sci 16:295–301
- Sun W, Tian W, Zhang YY, et al. (2012). Effect of novel stabilizers – cationic polymers on the particle size and physical stability of poorly soluble drug nanocrystals. Nanomed Nanotechnol Biol Med 8:460–7
- Wan AJ, Sun Y, Li HL. (2008). Characterization of folate-graft-chitosan as a scaffold for nitric oxide release. Int J Biol Macromol 43:415–21
- Wu L, Zhang J, Watanabe W. (2011). Physical and chemical stability of drug nanoparticles. Adv Drug Deliv Rev 63:456–69
- Yen WC. (2004). A selective retinoid X receptor agonist bexarotene (targretin) prevents and overcomes acquired paclitaxel (taxol) resistance in human non-small cell lung cancer. Clin Cancer Res 10:8656–64
- Zhang H, Hollis CP, Zhang Q, Li TL. (2011). Preparation and antitumor study of camptothecin nanocrystals. Int J Pharm 415:293–300
- Zhang Q, Pan J, Zhang J, et al. (2010). Aerosolized bexarotene inhibits lung tumorigenesis without increasing plasma triglyceride and cholesterol levels in mice. Cancer Prevent Res 4:270–6
- Zhang ZP, Lee SH, Feng SS. (2007). Folate-decorated poly(lactide-co-glycolide)-vitamin E TPGS nanoparticles for targeted drug delivery. Biomaterials 28:1889–99
- Zhu HY, Liu F, Guo J, et al. (2011). Folate-modified chitosan micelles with enhanced tumor targeting evaluated by near infrared imaging system. Carbohydr Polym 86:1118–29