Abstract
Alpha-Momorcharin (α-MMC) is a ribosome inactivating protein from Momordica charantia with anti-tumor activity. Previously, we had observed that modification of α-MMC with polyethylene glycol (PEG) could reduce toxicity, but it also reduces its anti-tumor activity in vitro. This study aims to investigate whether the metabolism-extended properties of α-MMC resulting from PEGylation could preserve its anti-tumor efficacy in vivo through pharmacokinetics and antitumor experiments. The pharmacokinetics experiments were conducted in rats using the TCA (Trichloroacetic Acid) method. Antitumor activity in vivo was investigated in murine mammary carcinoma (EMT-6) and human mammary carcinoma (MDA-MB-231) transplanted tumor mouse models. The results showed that PEGylation increased the plasma half-life of α-MMC in rats from 6.2–7.5 h to 52–87 h. When administered at 1 mg/kg, α-MMC-PEG and α-MMC showed similar anti-tumor activities in vivo, with a T/C% of 38.56% for α-MMC versus 35.43% for α-MMC-PEG in the EMT-6 tumor model and 36.30% for α-MMC versus 39.88% for α-MMC-PEG in the MDA-MB-231 tumor model (p > 0.05). Importantly, at the dose of 3 mg/kg, all the animals treated with α-MMC died while the animals treated with α-MMC-PEG exhibited only moderate toxic reactions, and α-MMC-PEG exhibited improved anti-tumor efficacy with a T/C% (relative tumor growth rate) of 25.18% and 21.07% in the EMT-6 and MDA-MB-231 tumor models, respectively. The present study demonstrates that PEGylation extends the half-life of α-MMC and alleviates non-specific toxicity, thereby preserving its antitumor efficacy in vivo, and a higher lever of dosage can be used to achieve better therapeutic efficacy.
Introduction
Bitter melon (formally named Momordica charantia L.) has been widely planted in China, Southeast Asia and other areas for centuries as an herbal medicine. In recent years, active proteins that shared identical conserved sequences and similar anti-tumor and anti-virus effects were discovered, extracted and purified from the bitter melon seeds (Wang et al., Citation2000; Zhu et al., Citation2001; Park et al., Citation2002; Park et al., Citation2006; Li et al., Citation2009), such as Alpha-Momorcharin (α-MMC), β-Momorcharin, δ-Momorcharin, γ-Momorcharin and Momordica. These proteins belong to a family of ribosome-inactivating proteins (RIPs) that exert anti-tumor effects through the following mechanisms: (1) inhibiting protein synthesis in tumor cells through inactivating ribosomes by RNA N-glycosidase; (2) inducing cell apoptosis through RNase and DNase activity (Ng et al., Citation1994; Basch et al., Citation2003; Kaur et al., Citation2012). RIPs have attracted considerable attention because of their potential use as novel anti-tumor agents with mechanisms of action that differ from those of conventional chemotherapeutic drugs.
However, as proteins originating from plants, RIPs have a wide range of toxic side effects in our inchoate studies (Meng et al., Citation2012). These side effects include severe systemic anaphylaxis, immunogenicity and toxicity such as hepatotoxicity and bone marrow inhibition. The side effects of α-MMC severely hinder its use as a therapeutic in clinical practice. To alleviate these undesirable properties, we have employed one of the most effective ways to reduce the immunogenicity and toxicity of α-MMC, namely, chemical modification with polyethylene glycol (PEG). Following PEGylation, the immunogenicity and immunotoxicity of α-MMC were dramatically reduced in vivo (Zheng et al., Citation2012). However, the anti-tumor activity of PEGylated α-MMC was also significantly decreased in vitro. In fact, the PEGylated α-MMC only retained 50–70% tumor cytotoxicity compared with that of the naked protein (Bian et al., Citation2010). Whether the anti-tumor activity of PEGylated α-MMC would also be significantly decreased in vivo was unknown.
It is well known that PEGylation can improve the plasma half-life of certain proteins. Therefore, it is not surprising that the drug retention time of PEGylated proteins is often prolonged in vivo. For this reason, we speculated that the anti-tumor activity of α-MMC-PEG might not decline sharply in vivo. Consequently, we designed the present study to investigate the pharmacokinetic profiles of α-MMC-PEG and to examine whether its antitumor efficacy can be effectively preserved in vivo.
Materials and methods
Chemicals and reagents
Bitter melon seeds were obtained from the Institute of Agricultural Science and Technique of Sichuan Province, China. MacroCapTM SP, Superdex 75, Sephacryl S100 were purchased from Amersham Pharmacia Biotech (Piscataway, NJ). (mPEG)2-Lys-NHS (20 kDa) was obtained from Shearwater Polymers (Huntsville, AL). Na125I was purchased from Perkin Elmer Life Science (Boston, MA). DMEM was obtained from GIBCO (Grand Island, NY). Fetal bovine serum (FBS) was purchased from HYCLONE (Logan, UT). Chloramine T was obtained from Sigma Chemical Co. (St. Louis, MO).
Preparation of α-MMC and chemical synthesis of α-MMC-PEG conjugate
The α-MMC and α-MMC-PEG conjugate were prepared as reported previously (Bian et al., Citation2010). Briefly, the powder of bitter melon seeds was extracted with pH 6.5 0.1 M phosphate buffer containing 0.15 M NaCl. The supernatant was precipitated by 30–65% ammonium sulfate fractionation and then loaded onto SP-Sepharose FF, Superdex 75 and Macro-cap SP columns, successively. The purified α-MMC was characterized by SDS-PAGE, acidic PAGE, HPLC, isoelectric focusing (IEF) and electrospray ionization quadrupled mass spectrometry (ESI-QUAD-MS). In general, PEGylated α-MMC was obtained in the following optimal conditions: 10 mg/ml of α-MMC reacted with (mPEG)2-Lys-NHS (mass ratio of PEG:RIP was 2:1) in pH 8.5, 100 mM borate buffer at room temperature for 30 min. The reaction mixture was applied with Sephacryl S-200 column or Macro-Cap SP column. The PEGylated conjugate was assessed by SDS-PAGE, RP-HPLC and MALDI-TOF-MS. α-MMC-PEG conjugate solution was filtrated through a 0.22-μm membrane and stored at 4 °C for subsequent experiments.
Preparation of 125I-α-MMC and 125I-α-MMC-PEG
125I labeled α-MMC and α-MMC-PEG were prepared using the Chloramine-T method and purified using a Sephadex G-100 column (10.0 × 300 mm; elutriation: 0.05 M PBS, pH 7.4). The radiochemical purity was determined by Trichloroacetic Acid (TCA) precipitation.
Experimental animals
Sprague–Dawley rats (male, SPF-quality, 250–350 g), Balb/C nude mice (female, SPF, 16–18 g) and Balb/C mice (male, SPF, 16–18 g) were purchased from Shanghai Experimental Animal Center, Chinese Academy of Sciences (Shanghai, China). Animals were housed in SPF animal rooms maintained at 22–25 °C with a 12 h light-dark cycle and relative humidity of 50–70%. The animals were provided with fresh water and standardized food ad libitum. Animals were acclimatized for at least 6 d prior to entering the studies, and all of the animal studies were conducted in accordance with the Guideline for Animal Experimentation of Chengdu Medical College.
Pharmacokinetic study in rats
All animals were given intraperitoneal injections of 1% KI solution 12 h prior to the test administration. Thirty-two male SD rats were randomly divided into four groups. Groups 1 and 2 received a single iv bolus injection of 125I-α-MMC at 2 mg/kg and 0.5 mg/kg, respectively, via tail vein, while Groups 3 and 4 received 125I-α-MMC-PEG injections at the same doses. Serum samples were collected via retro-orbital bleeding at the following time points after injection and stored at 4 °C before use: 1, 5 and 30 min; 1, 4, 12 h; 1, 2, 3, 4, 5, 8 and 12 d. Serum and 2-fold volume of 20% TCA were mixed well and spun at ∼10 000 × g for 10 min, TCA-precipitation radioactivity (cpm) was determined by Gamma counter (Wallac 1480 WIZARD®3, PerkinElmer, Finland), and the concentrations of α -MMC/ α -MMC-PEG in a sample were obtained based on a calibration curve. Mean serum concentrations of all samples at the same time point for each group were used and the pharmacokinetic parameters were fitted by non-compartmental model using software DAS 2.0 (Mathematical Pharmacology Professional Committee of China, Shanghai, China). The terminal half-life (t1/2) was calculated as 0.693/kel, where kel was the slope of the terminal phase determined by the last 3–5 concentration time points that had the best linear regression coefficient. The areas under the curve (AUC) were estimated by a linear-trapezoidal method.
Cells
Mouse mammary carcinoma cells (EMT-6) and human mammary carcinoma cells (MDA-MB-231) were kindly provided by the Shanghai Research Center of Biotechnology, Chinese Academy of Sciences (Shanghai, China). EMT-6 or MDA-MB-231 were cultured in DMEM containing 10% heat-inactivated FBS, 2 mM L-glutamine, 100 U/ml penicillin and 100 μg/ml streptomycin at 37 °C under 5% CO2/95% air.
Antitumor efficacy study in vivo
The murine mammary carcinoma model was established in male Balb/C mice by subcutaneous injection of 2 × 106 EMT-6 cells per mouse (injection volume of 0.2 ml). The human mammary carcinoma model was established in female Balb/C nude mice by subcutaneous injection of 2 × 106 MDA-MB-231 cells per mouse. For each model, mice were randomized into seven groups (n = 8 for each group) when tumor volume reached 80 to 100 mm3. The mice in groups 1, 2 and 3 were injected with α-MMC at concentrations of 3 mg/kg, 1 mg/kg or 0.3 mg/kg by intraperitoneal injection, respectively. The mice in groups 4, 5 and 6 received α-MMC-PEG injections at the same doses at a rate of three times a week for 6 weeks. The mice in the control group were immunized with saline only. During administration, all groups of mice were monitored by daily condition observation as well as with weekly tumor size and body weight measurements. The tumor volume was calculated as Width2 × Length/2, and the individual relative tumor volume (RTV) was calculated as RTV = Vx/V0, where Vx was the volume at a given time and V0 at the start of the treatment. Relative tumor growth rate (T/C%) was then calculated as RTVtreated/RTVcontrol × 100. T/C(%) was an effective index for evaluating anti-tumor effect in vivo (Alley et al., Citation2004). Evaluation criteria were the following: T/C(%) > 40 indicating invalid treatment and T/C(%) ≤ 40 indicating valid treatment, with the treatment group showing statistically significant differences from the negative control group (p < 0.05) (Alley et al., Citation2004).
Statistical analysis in the antitumor study
Data are reported as the means ± SD of more than three experiments. Analysis of variance was used to test the statistical significance of difference among groups. Statistical significance was evaluated using Student’s t-test or Dunnett`s test for the single or multiple comparisons of experimental groups, respectively. p < 0.05 was considered to be statistically significant.
Results
Identification of α-MMC and α-MMC-PEG conjugate
The purity of α-MMC was assessed to be homogenous by a combination of SDS-PAGE, HPLC, PAGE and IEF-PAGE. The accurate molecular weight of α-MMC was determined to be 28 585.183 Da. by MALDI-TOF-MS and a representative mass spectrometry map, YLLMHLFNYDGK by ESI-QUAD-MS. α-MMC-PEG conjugate with one-mer, two-mer and very little three-mer PEG-RIPs was detected by MALDI-TOF-MS and gradient SDS-PAGE. The detailed data for identification have been shown in our previous study (Bian et al., Citation2010).
125I-α-MMC and 125I-α-MMC-PEG
The radiochemical purity of 125I-α-MMC and 125I-α-MMC-PEG, determined using the TCA precipitation method, were (98.86 ± 0.51) % (n = 4) and (96.46 ± 0.71) % (n = 4), respectively. When stored at 4 °C, the radiochemical purity could remain above 95% for 2 days for 125I-α-MMC and 4 days for 125I-α-MMC-PEG.
Pharmacokinetics study in rats
The serum concentration-time profiles of 125I-α-MMC and 125I-α-MMC-PEG in SD rats after a single iv dose are summarized in and the pharmacokinetic parameters of α-MMC and α-MMC-PEG in serum are shown in . The concentration–time curve of α-MMC after iv injection was in accordance with a two-compartment model. However, the concentration-time curve of α-MMC-PEG fit with a three-compartment model. The plasma clearance rate of α-MMC in rats decreased from 0.123 L/h/kg to 0.002 L/h/kg, and its plasma half-life was extended by 7–15-fold (from 6.2–7.5 h to 52–87 h), and its area under the curve (AUC) was increased by 40-fold compared with the unmodified α-MMC. These results showed that PEG modification could significantly improve the pharmacokinetics of α-MMC, indicating that α-MMC-PEG could maintain higher blood drug concentration in the body for a longer period of time.
Figure 1. Serum concentration–time profiles of α-MMC and α-MMC-PEG in SD rats after a single iv bolus injection.
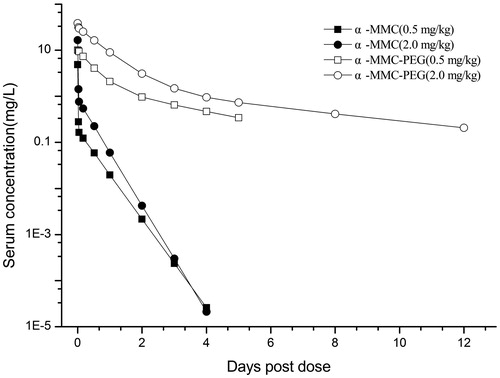
Table 1. Pharmacokinetic parameters of α-MMC and α-MMC-PEG in serum of SD rats after a single iv bolus injection.
Antitumor efficacy studies in vivo
The anti-tumor efficacy of α-MMC or α-MMC-PEG in the murine mammary carcinoma model was first tested. In the animals treated with the high dose of α-MMC (3 mg/kg), all mice exhibited serious toxic reactions, such as body weight loss (), reduced activeness, loss of appetite, piloerection, unresponsiveness and malaise. All of the mice treated with the high dose α-MMC died after the second week’s dose. In animals treated with the middle dose of α-MMC (1 mg/kg), all mice showed obvious toxic reactions but none of the animals died. In the animals treated with the low dose of α-MMC (0.03 mg/kg), only a mild toxic reaction was observed. These phenomena were consistent with our previous studies in rats (Meng et al., Citation2012; Zheng et al., Citation2012) and again verified that α-MMC has a strong toxic effect correlated with the dosage. However, following PEGylation, the toxicity of α-MMC was significantly reduced. The administration of 3 mg/kg of α-MMC-PEG only induced mild to moderate toxic reactions such as reduced activeness, loss of appetite and body weight loss. No such toxic reactions were observed in the groups of α-MMC-PEG administered at 1 mg/kg and 0.3 mg/kg.
Figure 2. Antitumor effects of repeated ip dose of α-MMC or α-MMC-PEG against EMT-6 mouse mammary carcinoma tumor in male Balb/C mice. Eight mice were used for each group. (A) body weight. (B) Tumor growth, expressed as the mean ± SD of relative tumor volume (%). ‘*’– p < 0.05, compared to control group.
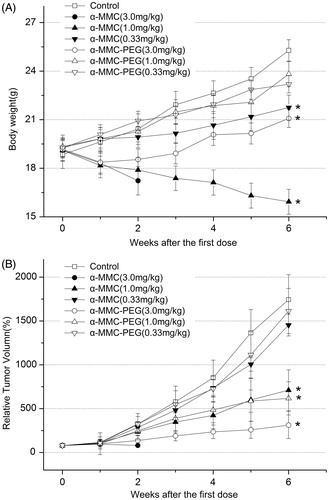
The tumor size was recorded and the RTV was calculated for each animal during administration (). The results in the saline control group showed that the EMT-6 tumor was very aggressive and grew over 17-fold in 6 weeks. In the groups given the dose of 0.03 mg/kg, the mean terminal RTV and relative tumor growth rate (T/C%) of α-MMC/α-MMC-PEG were 1452.40%/1613.22% and 83.3%/92.54%, respectively, which indicated that neither α-MMC nor α-MMC-PEG could inhibit the tumor growth at this level (). However, 1 mg/kg of α-MMC and α-MMC-PEG exhibited remarkable and similar tumor inhibition, with mean terminal RTVs of 710.20% and 617.64% and T/C% values of 40.74% and 35.43%, respectively. It was interesting that the animals in the group treated with 3.0 mg/kg of α-MMC all died after the second week’s dose, whereas α-MMC-PEG exhibited the best anti-tumor effect compared with other groups, with a mean terminal RTV of 312.45% and T/C% of 17.92%. We also noticed that the antitumor efficacy was not significantly different between groups treated with α-MMC and α-MMC-PEG at the same dose (p > 0.05), but there was a significant difference between the control group and the α-MMC- or α-MMC-PEG-treated groups (p < 0.05).
Table 2. Antitumor activity of α-MMC and α-MMC-PEG on EMT-6 rat breast cancer in vivo (x ± s; n = 8).
Similar results were observed in the human mammary carcinoma model (). High dosage of α-MMC (3.0 mg/kg) induced serious toxic reactions, and the anti-tumor effect was not observed due to all of the animals dying after the second week’s dose. However, at the same dosage, α-MMC-PEG only induced mild to moderate toxic reactions and exhibited the best anti-tumor effect with T/C% of 21.07% (). The middle dosage of α-MMC (1.0 mg/kg) could induce an obvious toxic reaction and exhibited remarkable anti-tumor activity with T/C% of 36.3%. α-MMC-PEG displayed equal anti-tumor effects with a T/C% of 39.88% but had no obvious toxic effect at this dosage. α-MMC and α-MMC-PEG could not inhibit the tumor growth at the low dosage of 0.03 mg/kg (with T/C% of 82.54% and 88.46%, respectively) (p > 0.05).
Figure 3. Antitumor effects of repeated ip dose of α-MMC or α-MMC-PEG against MDA-MB-231 human mammary carcinoma tumor in female Balb/C nude mice. Eight mice were used for each group. (A) body weight. (B) Tumor growth, expressed as the mean ± SD of relative tumor volume (%). ‘*’– p < 0.05, compared to control group.
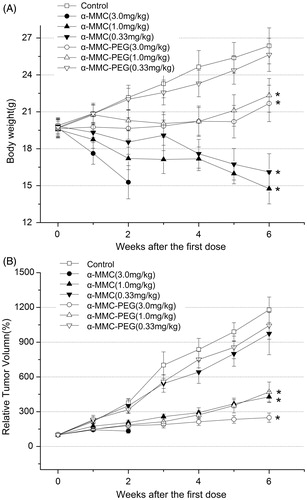
Table 3. Antitumor activity of α-MMC and α-MMC-PEG on MDA-MB-231 human breast cancer in vivo (x ± s; n = 8).
Discussion
The high immunogenicity, immunotoxicity and hepatotoxicity (Meng et al., Citation2012) of α-MMC and other RIPs strongly hinder their clinical application as antitumor or antiviral agents. We previously reported that PEGylation, a widely used chemical modification of protein therapeutics, of α-MMC effectively alleviated the immune response in vivo (Zheng et al., Citation2012) but at the same time reduced 50–70% of the antitumor activities in vitro (Bian et al., Citation2010). This phenomenon was attributed to the fact that the PEGylation occurs in or near the antigen-binding domain, which shares some identical peptide residues with the RIP active domain. For example, residues 71–136 were found to be in close proximity to the active site involved in ribosome inactivation, and treatment with a monoclonal antibody directed to this area was shown to be effective in inactivating the inhibitory effect of α-MMC on protein synthesis in vitro (Leung et al., Citation1997). However, we speculated that the reduced bioactivity in vitro did not necessarily lead to reduced efficacy in vivo because the circulation half-life of the PEGylated proteins may be extended. For instance, PEGylated α-interferon Pegasys® retained only 7% of the antiviral activity of the original protein but still displayed a greatly improved efficacy in vivo (Bailon et al., Citation2001). Other examples include the PEGylated trichosanthin mutant, which was a RIP (He et al., Citation1999; Wang et al., Citation2004), and the PEGylated growth hormone (Clark et al., Citation1996; Niv-Spector et al., Citation2012). Motivated by these examples, we designed the present study to investigate and compare the pharmacokinetic profiles and antitumor efficacy in vivo of α-MMC, and PEGylated-α-MMC.
For the detection of α-MMC in biofluids, a sensitive sandwich ELISA method has been previously developed by our group using a monoclonal antibody against α-MMC (unpublished data). However, this antibody could not be used for the detection of α-MMC-PEG because the antigen epitope of α-MMC might be blocked by PEG and thus strongly reduce the sensitivity and accuracy of this immunoanalytical method. Considering that the PEGylation of α-MMC occurs on a lysine residue, not the tyrosine or histidine residue where 125-iodine labeling occurs, a 125I radiolabeled quantitative method to detect the α-MMC and α-MMC-PEG in plasma was developed. This method uses gamma-counting based on TCA-precipitation, with a lower limit of quantification (LLOQ) of 5 ng/ml in plasma, an accuracy of RE (mean bias) <16%, and a precision of CV < 13.5%. Thus, this method qualifies for our pharmacokinetic study. Our pharmacokinetic study in rats showed that after PEGylation, the plasma half-life of α-MMC was extended by 7- to 15-fold (from 6.2–7.5 h to 52–87 h), and the drug exposure, calculated as the AUC, was increased by 40-fold compared with the unmodified α-MMC. These results were consistent with many published reports on PEGylated proteins. It was well accepted that the extended half-life can be attributed to the increased molecular size and steric hindrance which can reduce the proteolysis and restrict the tissue distribution of the drug, similar to glomerular filtration by the kidney and hepatic uptake.
As the mammary carcinoma cell lines were shown to be sensitive to α-MMC induced cytotoxicity in our previous in vitro studies (Alley et al., Citation2004), we established the EMT-6 and MDA-MB-231 tumor models in Balb/C mice and Balb/C nude mice, respectively, to compare the antitumor activities of α-MMC and PEGylated α-MMC. The results showed that when repeatedly dosed at 1 mg/kg, the relative tumor growth rate (T/C %) for α-MMC and α-MMC-PEG were 40.74% and 35.43% in the EMT-6 tumor model and 36.30% and 39.88% in the MDA-231 tumor model, respectively. It was illustrated that PEGylated and unmodified α-MMC exhibited similar antitumor activities in vivo at this dosage. Although the active sites of α-MMC were blocked by PEG, the activities were well retained in vivo. This was likely due to the extended plasma half-life, which allows more drug exposure to the tumor cells.
More significantly, PEGylation could alleviate the toxicity of α-MMC that allowed the experiment animals to endure high dosage (3.0 mg/kg). Animals dosed with the high level of α-MMC-PEG only exhibited mild to moderate toxic reactions, such as body weight loss and reduced activeness, and showed even better anti-tumor activity (17.92% (T/C %) in the EMT-6 model and 21.07% in MDA-231 model). These data strongly support the use of α-MMC at a higher dose after PEGylation, which could enhance the antitumor efficacy with tolerable toxic reactions in vivo.
In conclusion, PEGylation of α-MMC does not compromise its therapeutic potency as an antitumor agent due to (1) its increased plasma half-life, and (2) its reduced toxicity which allows the use of a higher dose. These results suggest a new finding of the PEGylation; that is, PEGylation may raise the tolerance of toxic reactions for patients and resulting in a greater therapeutic window for clinical applications.
Declaration of interest
This work was supported by the National Science Foundation of China (Grant No. 30770232) and the Natural Science Foundation of Department of Education of Sichuan Province (No. 14CZ023). All authors had reviewed and agree with the contents of this manuscript and report no conflicts of interest.
References
- Alley MC, Hollingshead MG, Dykes DJ, Waud WR. (2004). Human tumor xenograft models in NCI drug development. In: Teicher BA, Andrews PA, eds. Anticancer drug development guide: preclinical screening, clinical trials, and approval. 2nd ed. Totowa (NJ): Humana Press, Inc., 125–52
- Bailon P, Palleroni A, Schaffer CA, et al. (2001). Rational design of a potent, long-lasting form of interferon: a 40 kDa branched polyethylene glycol-conjugated interferon alpha-2a for the treatment of hepatitis C. Bioconjug Chem 12:195–202
- Basch E, Gabardi S, Ulbricht C. (2003). Bitter melon (Momordica charantia): a review of efficacy and safety. Am J Health Syst Pharm 60:356–9
- Bian X, Shen F, Chen Y, et al. (2010). PEGylation of alpha-momorcharin: synthesis and characterization of novel anti-tumor conjugates with therapeutic potential. Biotechnol Lett 32:883–90
- Clark R, Olson K, Fuh G, et al. (1996). Long-acting growth hormones produced by conjugation with polyethylene glycol. J Biol Chem 271:21969–77
- He XH, Shaw PC, Tam SC. (1999). Reducing the immunogenicity and improving the in vivo activity of trichosanthin by site-directed pegylation. Life Sci 65:355–68
- Kaur I, Yadav SK, Hariprasad G, et al. (2012). Balsamin, a novel ribosome-inactivating protein from the seeds of Balsam apple Momordica balsamina. Amino Acids 43:973–81
- Leung KC, Meng ZQ, Ho WK. (1997). Antigenic determination fragments of alpha-momorcharin. Biochim Biophys Acta 1336:419–24
- Li M, Chen Y, Liu Z, et al. (2009). Anti-tumor activity and immunological modification of ribosome-inactivating protein (RIP) from Momordica charantia by covalent attachment of polyethylene glycol. Acta Biochim Biophys Sin (Shanghai) 41:792–9
- Meng Y, Liu B, Lei N, et al. (2012). Alpha-momorcharin possessing high immunogenicity, immunotoxicity and hepatotoxicity in SD rats. J Ethnopharmacol 139:590–8
- Ng TB, Liu WK, Sze SF, Yeung HW. (1994). Action of alpha-momorcharin, a ribosome inactivating protein, on cultured tumor cell lines. Gen Pharmacol 25:75–7
- Niv-Spector L, Shpilman M, Boisclair Y, Gertler A. (2012). Large-scale preparation and characterization of non-pegylated and pegylated superactive ovine leptin antagonist. Protein Expr Purif 81:186–92
- Park SW, Lawrence CB, Linden JC, Vivanco JM. (2002). Isolation and characterization of a novel ribosome-inactivating protein from root cultures of pokeweed and its mechanism of secretion from roots. Plant Physiol 130:164–78
- Park SW, Prithiviraj B, Vepachedu R, Vivanco JM. (2006). Isolation and purification of ribosome-inactivating proteins. Methods Mol Biol 318:335–47
- Wang YX, Jacob J, Wingfield PT, et al. (2000). Anti-HIV and anti-tumor protein MAP30, a 30 kDa single-strand type-I RIP, shares similar secondary structure and beta-sheet topology with the A chain of ricin, a type-II RIP. Protein Sci 9:138–44
- Wang JH, Tam SC, Huang H, et al. (2004). Site-directed PEGylation of trichosanthin retained its anti-HIV activity with reduced potency in vitro. Biochem Biophys Res Commun 317:965–71
- Zheng JC, Lei N, He QC, et al. (2012). PEGylation is effective in reducing immunogenicity, immunotoxicity, and hepatotoxicity of alpha-momorcharin in vivo. Immunopharmacol Immunotoxicol 34:866–73
- Zhu G, Huang Q, Qian M, Tang Y. (2001). Crystal structure of alpha-momorcharin in 80% acetonitrile–water mixture. Biochim Biophys Acta 1548:152–8