Abstract
In this study, for the first time, glycol chitosan (GC) nanoparticles (NPs) were prepared and evaluated to obtain systemic and mucosal immune responses against nasally administered hepatitis B surface antigen (HBsAg). Size, zeta potential and morphology of the NPs were investigated as a function of preparation method. NPs with high loading efficacy ( > 95%) and positively charged surface were obtained with an average particle size of approximately 200 nm. The structural integrity of HBsAg in NPs was evaluated by sodium dodecyl sulfate polyacrylamide gel electrophoresis analysis and further confirmed by measuring the in vitro antigenicity using an enzyme immunoassay. During in vivo studies, GC NPs showed the lowest nasal clearance rate and better mucosal uptake when compared with chitosan (CS) NPs. The immunogenicity of NPs-based delivery system(s) was assessed by measuring anti-HBsAg antibody titer in mice serum and secretions after intranasal administration. The alum-based HBsAg vaccine injected subcutaneously was used as positive control. Results indicated that alum-based HBsAg induced strong humoral but negligible mucosal immunity. However, GC NPs induced stronger immune response at both of the fronts as compared to generated by CS NPs. This study demonstrates that this newly developed system has potential for mucosal administration of vaccines.
Introduction
The development of vaccine delivery systems remains a major challenge in the field of immunology. The majority of the currently licensed vaccines are recommended to be administered through parenteral route. Parenteral immunization has obvious disadvantages of high production costs, low compliance and lack of mucosal immune response. Consequently, non-invasive mucosal routes of immunization are being explored, which are known to elicit the mucosal as well as systemic immune response (Slütter et al., Citation2008; Jain et al., Citation2011). In particular, the nasal mucosa represents a potential portal for vaccine administration. Nasal epithelium layer consist of specialized antigen sampling microfold-cells (M-cells) overlaying the nasal-associated lymphoid tissue (NALT), which results into antigen uptake and subsequently the mucosal and systemic immune induction (Vajdy & O'Hagan, Citation2001). However, variety of factors limits nasal delivery of biomacromolecules such as peptides, proteins and vaccines including, mucociliary clearance, low permeation and metabolic degradation (Borges et al., Citation2010).
Biodegradable and mucoadhesive nanoparticles (NPs) seems to be the most promising candidate for nasal vaccine delivery system due to their inherent immune adjuvant property and ability to increase the residence time of the delivered content in nasal cavity (Illum et al., Citation1994; Felt et al., Citation1998). It has been widely documented that NPs are preferentially taken up by M cells. After uptake, M cells present the content of NPs to the underlying professional antigen presenting cells (APCs) for the immune induction (Felt et al., Citation1998).
Chitosan (CS) has attracted sizeable attention for mucosal vaccine delivery systems as it possesses the favorable biological properties such as biocompatibility, biodegradability, mucoadhesive properties and permeation-enhancing ability (Artursson et al., Citation1994; Felt et al., Citation1998). In spite of all its superior properties, CS has a major drawback that at physiological pH, CS loses its ability to enhance drug permeability and absorption (Kotzé et al., Citation1999). Therefore, various chemical modifications have been introduced to increase the water solubility of CS (Mourya & Inamdar, Citation2008; Arca et al., Citation2009; Werle et al, Citation2009). Glycol chitosan (GC) is a CS derivative conjugated with ethylene glycol branches, water soluble at a neutral/acidic pH values where the pendant glycol branches on the polymer increase both the aqueous solubility of the native CS and provide steric stabilization (Gogev et al., Citation2004; Trapania et al., Citation2009). Based on these outcomes, the development of a novel nanocarrier system consisting of GC intended for mucosal delivery of biomacromolecules seemed of interest.
In a previous study, we showed that trimethyl CS (quaternized CS) NPs had interesting features suitable for nasal antigen delivery (Mangal et al., Citation2011). The main objective of this study is to explore the GC NPs and compare its efficacy with CS NPs for nasal vaccine delivery application. The CS and GC NPs were prepared and characterized based on their particles size, zeta potential, antigen entrapment and mucin adhesion ability. The nasal residence time and uptake of CS and GC NPs was also compared. In addition, immune adjuvant ability of NPs-based formulation was investigated by measuring hepatitis B surface antigen (HBsAg)-specific systemic and secretory antibody titers in serum and secretions, respectively.
Materials and methods
Materials
CS with molecular weight (Mw) 110–150 kDa, GC (Mw: 250 kDa), mucin type III and FITC–bovine serum albumin (BSA) were procured from Sigma Chemical Co. (St. Louis, MO). Recombinant HBsAg (Mw: 24 kDa) was from Shantha Biotechnics Ltd. BCA protein estimation kit and protein Mw markers were purchased from Genei, Bangalore, India. MONOLISA™ HBsAg Ultra kit, enzyme-linked immunosorbent assay (ELISA) kit and cytokines (interleukin [IL]-2, IL-4, IL-6 and interferon gamma [IFN-γ]) estimation kit was procured from Bio-Rad Laboratories (Hercules, CA), Sigma-Aldrich Chemical Co. and eBioscience (San Diego, CA), respectively. All other chemicals and reagents were of analytical grade and purchased from commercial vendors.
Preparation of NPs
CS and GC NPs were prepared using polyelectrolyte complexation method (Mao et al., Citation2005, Citation2006; Sadeghi et al., Citation2008). Briefly, CS was dissolved in 0.25% (v/v) acetic acid and GC was dissolved in distilled water to obtain a final concentration of 1 mg/mL. The pH of the polymer solution was adjusted to 5.5 using 1 M NaOH. The HBsAg solution (125 µg/mL) was added to the polymer solution in equal volume in a beaker under gentle magnetic stirring at room temperature for 2 h. Once an opalescent suspension was formed, the samples were centrifuged (Allegra 64R, Beckman Coulter, Pasadena, CA) at 22 000 rpm for 30 min at 4 °C. The supernatant was separated and used for the determination of entrapment efficiency. The pellet was subjected to freeze-drying (VirTis AdVantage). The freeze-dried powder was used for further studies.
Characterizations of NPs
Size, zeta potential and morphology
The morphology and surface appearance of the particles were examined by scanning electron microscopy (SEM). One drop of the particles suspension was placed on a gold-coated plate and maintained at least 12 h at room temperature in desiccators for complete dryness of the sample. The stub was then coated with gold using sputter coater. The sample was randomly scanned using SEM (LEO-435 VP, Cambridge, UK) and photomicrograph was developed. The particle size and zeta potential of NPs were recorded in 10 mM N-[2-hydroxyethyl] piperazine-N-[2-ethanesulfonic acid] (HEPES) buffer (pH 7.4) by Zetasizer Nano ZS (Malvern, UK) before and after freeze drying. HBsAg was checked for its size using DLS (Dynapro Plate Reader, Wyatt, Germany), and zeta potential was determined using Zetasizer Nano ZS.
Antigen-loading efficiency
The amount of HBsAg associated with NPs was calculated by determining the amount of antigen remained unassociated to the NPs. The supernatant was separated as described in section “preparation of NPs” in this article, and quantity of free HBsAg remaining in supernatant was estimated using BCA method. Blank samples were also subjected to the same conditions, which was used as a control to normalize the background interference for the correction of the optical density value by BCA protein assay:
Sodium dodecyl sulfate polyacrylamide gel electrophoresis and antigen ELISA
The structural integrity of HBsAg-loaded in NPs was evaluated by sodium dodecyl sulfate polyacrylamide gel electrophoresis (SDS-PAGE) analysis. Briefly, HBsAg-loaded CS and GC NPs were incubated overnight in phosphate buffered saline (PBS; pH 7.4) at 37 °C under mild agitation (50 rpm). The antigen released from NPs was separated by centrifugation (Allegra 64 R, Beckman Coulter) at 22 000 rpm for 30 min. Supernatant was separated and suspended in SDS-loading buffer. The samples were run on 10% SDS-PAGE gel at 200 V until the dye band reached the gel bottom. Proteins were visualized by coomassie blue staining. The same sample was also used to measure the in vitro antigenicity using an enzyme immunoassay (EIA) (Jaganathan et al., Citation2004; Pawar et al., Citation2013). Each sample (HBsAg extracted from the NPs) was diluted with 0.2% (w/v) BSA in PBS (pH 7.4) to get three different concentrations and examined against a linear fitting to the response of control standard sample. The in vitro antigenicity of HBsAg was evaluated by determining the ratio of EIA response and protein concentration (EIA/protein).
In vitro studies
In vitro release
HBsAg release was determined by incubating NPs with PBS (pH 7.4) at 37 °C under mild agitation (50 rpm). Samples were withdrawn at predetermine time points, centrifuged at 22 000 rpm for 30 min, and the supernatant was quantified for the amount of free HBsAg by micro BCA method. The background value was neutralized using the blank samples to correct for the intrinsic absorption of the polymer (Borges et al., Citation2005).
In vitro toxicity studies
The cytotoxicity of the CS and GC NPs was tested in vitro on the differentiated human, mucus-producing, submucosal gland carcinoma cell line, Calu-3, using the procedure described by Amidi et al. (Citation2006). Calu-3 cells were seeded at a density 5 × 105 cells/well into 96-well culture plates in DMEM supplemented with 10% fetal calf serum and 50 µg/mL penicillin and streptomycin. The cells were incubated for two days at 37 °C in 95% air and 5% CO2. The formulations were prepared in Hank's balanced salt solution (HBSS) buffered with 30 mM HEPES, adjusted with 0.1 M NaOH to pH 7.2. Then, the cells were exposed to HBSS–HEPES buffer, a suspension of different concentration (0.2, 2.0 and 20 mg/mL) of NPs (CS and GC) and incubated for 2 h at 37 °C. Polyethyleneimine (PEI) in HBSS–HEPES was also incubated at different concentrations (0.2, 2.0 and 20 mg/mL) with calu-3 cell line for the purpose of comparison as PEI (cationic molecule) is accepted to possess high cell toxicity (Mathia et al., Citation2002). Thereafter, the formulations (CS and GC NPs), PEI and control(s) were replaced by 100 µL of DMEM and 20 µL cell proliferation reagent [3-(4,5-dimethylthiazole-2-yl)-5-(3-carboxymethoxyphenyl)-2-(4-sulfophenyl)-2H-tetrazolium], and the cells were incubated for 2 h at 37 °C. Finally, the absorbance was measured at 490 nm using a 96-well microplate reader (Mosmann, Citation1983). The cells incubated with HBSS–HEPES buffer was considered as negative control, and the cells incubated with SDS (0.01%, w/v) in NaOH (1%, w/v) for 10 min were considered as positive control.
Adsorption of mucin on NPs
The mucin adsorption ability of the different nanoparticulate formulations was determined following the procedure described in our previous study (Pawar et al., Citation2010). Briefly, equal volumes of NPs (2 mg/mL) and an aqueous solution of mucin (0.5 mg/mL) were mixed and vortexed at room temperature for 60 min. The suspension was then centrifuged and the supernatant was quantified for free mucin content using colorimetric assay (He et al., Citation1998). The mucin adsorbed on to the NPs surface was calculated by subtracting the amount of free mucin from the total mucin content in the test mixture.
In vivo studies
The Institutional Animals Ethical Committee of Jawaharlal Nehru Technical University approved the protocols, which were carried out by following the guidelines of the Council for the Purpose of Control and Supervision of Experiments on Animals, Ministry of Social Justice and Empowerment, Government of India.
Nasal clearance and nasal uptake studies
Nasal clearance study was performed in rabbits, and the radiolabeling was performed as described in our previous study (Mangal et al., Citation2011). Briefly, 50 mg of blank NPs was suspended in a labeling medium containing 1.5 mL of normal saline, 1 mL SnCl2·2H2O (5 mg/mL) and 200 µL sodium pertechnetate containing approximately 20 MBq of activity. The NPs suspension was stirred continuously for a period of 5 min, centrifuged and washed with acetone twice. Then, the NPs were dried in fume-hood overnight. The labeled NPs were suspended in PBS and administered in suspension form (5 mg/50 µL PBS). Lactose powder was labeled and used as a negative control. Lactose powder (50 mg) was dissolved in the above-mentioned labeling media and incubated for 10 min, followed by the addition of 10 mL acetone. The labeled lactose was precipitated in the presence of acetone. Supernatant was decanted and powder was washed with acetone and finally dried in fume hood. The activities were adjusted so that each 5 mg NPs would have 2 MBq activity at the time of administration.
The male New Zealand white rabbits were used. The animals were taken care throughout the period of the experiment undertaken in the nuclear medicine department of the Jawaharlal Nehru Cancer Hospital and Research Center, Bhopal, India. They were housed individually in stainless steel cages, fed with a commercial laboratory rabbit diet and had free access to water. The deposition and subsequent clearance of labeled CS and GC NPs and plain HBsAg antigen were evaluated by gamma scintigraphy, using a gamma camera (Seimens, E-Cam, Erlangen, Germany) fitted with a low-energy (140 keV) collimator at different time intervals. The clearance of NPs from the nasal cavity was evaluated as the decrease in percentage radioactivity against time.
The fluorescence microscopy was performed to confirm deposition of NPs in NALT following nasal administration. FITC–BSA-labeled NPs were prepared using the procedure described earlier in this manuscript. Dye-loaded NPs were administered to mice intranasally (i.n.) using the procedure described elsewhere in this article. The mice were sacrificed after 30 min of vaccine administration. Nasal cavity containing nasal mucosa was cut into pieces and washed with Ringer's solution. The tissues were fixed in Carnoy's fluid, and tissue blocks were prepared with paraffin wax. Then microtomy was done, and the tissues were mounted on slides. The slides were then analyzed under fluorescence microscope (Nikon Eclips E 200, Tokyo, Japan). FITC–BSA in PBS (pH 7.4) was also administered i.n. following the same protocol to mice and was kept as control group.
Immunization studies
Female BALB/c mice 8–10 weeks old, weighing 20–25 g, were used for the immunization studies. Mice were housed in the groups of six with free access to food and water with 12-h light/dark cycle one week for acclimatization. Animals were deprived of food 2 h before the formulations were administered. Nasal dosing was done to non-anesthetized mouse by inserting small piece of polyethylene tubing (inserting), attached to a Hamilton syringe, 0.2 cm into nostril, of the mice (supine position) and ejecting formulation into the nasal cavity. The tube was inserted at least 0.2 cm into the nostril to deposit the formulations into the nasal cavity. The amount of NPs representing 10 µg of HBsAg was resuspended in the PBS (pH 7.4) and administered through nasal route. The formulation was administered alternatively through each nostril in small drops in equal amount. Care was taken that a new drop was only given when the former had been entirely inhaled. Plain HBsAg solution (10 µg) was also administered nasally following the similar protocol. All groups received booster dose on day 21. Mice nasally administered with PBS (pH 7.4) were used as negative control. Alum-adsorbed HBsAg (10 µg) was administered subcutaneously and kept as control.
Sample collection
Blood samples were collected from the retro-orbital sinus under mild ether anesthesia at days 0, 14, 28 and 42. The blood samples were allowed to clot overnight and then centrifuged at 8000 × g for 5 min at room temperature to collect serum, which was stored at –40 °C until tested. The nasal, vaginal and salivary secretions were collected on 0 and 42 d of primary vaccination. Vaginal washes were obtained according to the method given by Debin et al. (Citation2002). Briefly, the vaginal tracts of non-anaesthetized mice were flushed by reintroducing 50 µL of PBS containing 1% (w/v) BSA using a Gilson pipette. These 50 µL aliquots were withdrawn and reintroduced nine times. The nasal wash was collected by cannulation by trachea of sacrificed mice (Jain et al., Citation2005). The nasal cavity of sacrificed mice was flushed three times with 0.5 mL of 1% BSA containing PBS (BSA–PBS, pH 7.4). Salivary secretion was collected using the method developed by Jain et al. (Citation2005). Briefly, salivation was induced by injecting 0.2 mL of pilocarpine intraperitoneally (i.p.). Saliva was collected from mice after 20 min using capillary tube. All secretions were stored with 100 mM phenylmethyl sulfonyl fluoride as a protease inhibitor at –40 °C until tested by ELISA for secretory antibody (sIgA) levels.
Measurement of anti-HBsAg IgG and IgA antibody titer
Anti-HBsAg antibodies in serum samples were determined using ELISA. Briefly, each well of microtiter plates (Nunc-Immuno Plate® Fb 96 Mexisorp, NUNC) was coated with 100 µL of 2 µg/mL HBsAg in carbonate buffer (pH 9.6) and incubated overnight at 2–8 °C. The plates were washed three times with PBS–Tween 20 (0.05%, v/v) (PBS-T) and blocked with PBS–BSA (3%, w/v) for 2 h at 37 °C, followed by washing with PBS-T. The serum/secretion samples were serially diluted with PBS. One hundred microliters of the serially diluted serum, and secretion samples were added to the wells of coated ELISA plates. The plates were incubated for 1 h at room temperature and washed three times with PBS-T. One hundred microliters of horse reddish peroxidase-labeled goat anti-mouse IgG and IgA (1:1000 dilution, Sigma) antibodies were added to well for the determination of IgG and IgA titer, respectively. The plates were kept for 1 h at room temperature and then washing was repeated. One hundred microliters of tetramethylbenzidine–H2O2 solution was added to each well. Color development was stopped after 30 min by adding 50 µL of 1 N H2SO4 to each well, and absorbance was taken at 490 nm using a plate reader (Bio-Rad). The end-point titers were expressed as the log reciprocal of the last dilution, which gave the absorbance value three time or above the absorbance of negative control (serum and secretion samples of mice received PBS, pH 7.4 nasally) at a wavelength of 490 nm.
Estimation of cytokine level
Endogenous levels of cytokines in mice were determined by splenocyte proliferation assay (Sachdeva et al., Citation2004). For the determination of cytokine production, 5 × 106 splenocytes/mL was cultured in a final volume of 200 µL in 96-well flat-bottom plates in the presence or absence of HBsAg. Culture supernatants were collected after 48 h for estimation of cytokine concentration (IL-2, IL-4, IL-6 and IFN-γ). All of these cytokines were measured by using a murine cytokine immunoassay kit (eBioscience) by following the procedure recommended by the manufacturer.
Statistical analysis
The results were expressed as mean ± standard deviation. Multiple comparisons were made using one way analysis of variance followed by post hoc analysis by Tukey test using Graphpad Prism software. Asterisk over bars indicated degree of significance, where *p < 0.05; **p < 0.01; ***p < 0.001; ns = not significant.
Results
Characterization of NPs
Size, zeta potential and morphology
HBsAg was characterized for particles size and zeta potential, which was found to be 26.7 ± 4.3 nm and − 15.4 ± 2.2 mV, respectively. CS and GC NPs were prepared by self-assembly, utilizing the electrostatic interactions between the positively charged polymers and negatively charged HBsAg as a driving force. represents the particles size, zeta potential and the antigen loading. The NPs obtained from different polymers were found to be around 200 nm in size. It was also noted that lyophilization did not affect the particles size of the CS and GC NPs. SEM images () showed that the NPs had smooth surface and almost spherical shape.
Table 1. Average particles size, PDI, zeta potential and entrapment efficiency of developed formulations.
Confirmation of the structural integrity and antigenicity
The integrity of the encapsulated antigen was evaluated by SDS-PAGE analysis. SDS-PAGE analysis followed by coomassie blue staining revealed identical bands for the native and entrapped antigen (). This indicates that the preparation conditions did not cause any irreversible aggregation or cleavage of the antigen. In addition, in vitro immunogenicity was also measured by EIA/protein ratio (relative potency) determination as described in our previous studies (Jaganathan et al., Citation2004; Pawar et al., Citation2013). The CS and GC NPs showed an EIA/protein ratio of 1.0 ± 0.2 and 1.0 ± 0.1, respectively.
Figure 2. SDS-PAGE analysis: SDS-PAGE showing stability of antigen isolated from formulations. (Lane 1) marker proteins (66.0 kDa: bovine serum albumin, 45.0 kDa: ovalbumin, 35.0 kDa: lactate dehydrogenase, 25 kDa: REase Bsp981, 18.4 kDa: β-galactoglobulin and 14.4 kDa: lysozyme); (lane 2) native HBsAg (24 kDa); (lane 3 and 4) HBsAg isolated from CS and GC NPs, respectively (24 kDa).
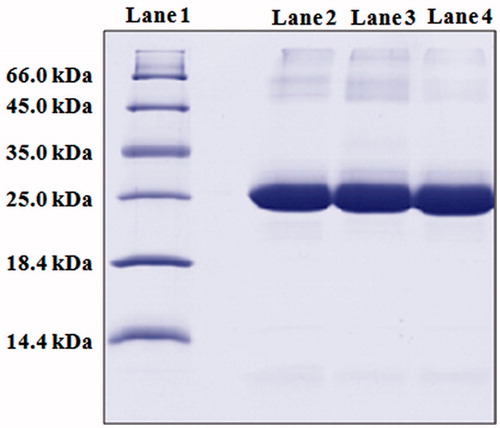
In vitro studies
In vitro release
In vitro release studies carried out in PBS (pH 7.4) and the release data of HBsAg from CS and GC NPs are displayed in . The overall release process of HBsAg was biphasic, with an initial burst effect, followed by a sustained release phase (Prego et al., Citation2010). The burst effect may have been related to desorption of the HBsAg close to the surface during preparation of the NPs (Amidi et al., Citation2007). Later, HBsAg was released slowly due to swelling or degradation of the polymer. The remaining HBsAg in NPs was not completely released into the medium, which might have been due to the interaction between the remaining HBsAg and the CS and GC. The particle integrity was monitored by turbidity transmission measurements at 500 nm, and it was observed that the particles could retain its suspension behavior during the release studies.
In vitro toxicity studies
Calu-3 cells are known as a relevant model for respiratory epithelium, since they form tight monolayers and secrete components of mucus and surfactant (Mathia et al., Citation2002). The effect of CS and GC NPs on the mitochondrial dehydrogenase activity of Calu-3 cells is shown in . Calu-3 cell line was incubated with different concentration of CS and GC NPs to determine the relative toxicity of particles at higher particles concentration. PEI (Florea et al., Citation2002) and SDS were included in this experiment as positive control. It was observed that CS and GC NPs exhibited no toxicity, which was comparable to the negative control (p > 0.05). In contrast, PEI demonstrated substantially higher cell toxicity as compared to CS and GC NPs at all concentrations (p < 0.05). The toxicity of CS and GC at higher concentrations (2 mg/mL and 20 mg/mL) was relatively higher when compared to lower concentration (0.2 mg/mL) but was not substantially different from that of the negative control (p > 0.05).
Figure 4. In vitro cell cytotoxicity: graph showing toxicity of different concentration of CS, GC NPs and PEI on Calu-3 cell line. The cells incubated with HBSS–HEPES buffer was considered as negative control, whereas the cells incubated with SDS was used as positive control. The results obtained were compared with CS NPs. Asterisk over bars indicated degree of significance. Where *p < 0.05; **p < 0.01; ***p < 0.001; ns = not significant.
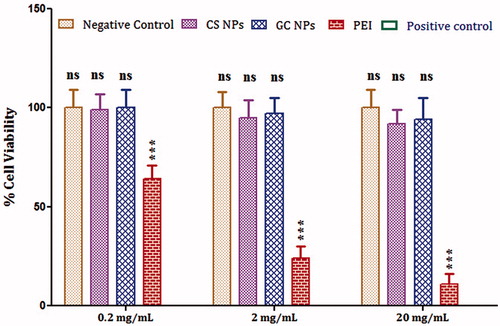
Adsorption of mucin on NPs
The ability of NPs to bind with mucin was measured as a function of their binding ability to the mucosal surfaces of the body (mucoadhesiveness). It was investigated that positive surface charge may be favorable in nasal vaccination due to their better mucoadhesive ability (Jaganathan & Vyas, Citation2006; Pawar et al., Citation2010). It was noticed that GC NPs demonstrated higher mucin retention ability (0.447 mg mucin/2 mg NPs) when compared with CS NPs (0.338 mg mucin/2 mg NPs).
In vivo studies
Nasal clearance and nasal uptake studies
It has been reported that the use of mucoadhesive polymers can increase the residence time in nasal cavity substantially (Soane et al., Citation2001; Illum, Citation2003). NPs were evaluated for their clearance rate as a measure of their muco-adhesiveness. Our results clearly indicated that the rate of clearance of the CS NPs and GC NPs was substantially low as compared to lactose and Plain HBsAg (), which were used as control. It was observed that GC demonstrated a substantially lower rate of clearance as compare to CS NPs (p < 0.05). This may be attributed to the better mucodheiveness observed in case of GC NPs.
Figure 5. Nasal clearance studies: graph showing the % clearance of nanoparticles from nasal mucosa. Results are expressed as mean ± SD (n = 3).
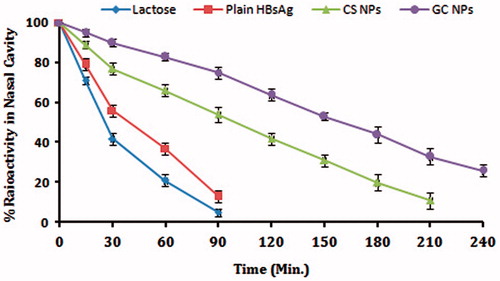
Particulate vaccine delivery systems are reported to be taken up by the M-cells and epithelial cells based upon their size (Eldridge et al., Citation1990). In our study, the particles size of CS and GC nanoparticulate formulation was approximately 200 nm. Results indicated that nasal administration of direct FITC–BSA solution did not produce any fluorescence. However, fluorescent-labeled CS and GC NPs demonstrated deposition into NALT (). It was also observed that GC NPs demonstrated comparatively higher fluorescence intensity and better deposition of FITC–BSA in NALT. It has also been accepted that CS and its derivatives possess bioadhesive properties and decrease the clearance of formulations from the nasal cavity, which eventually may lead to its uptake by M cells. Our results indicated that GC NPs demonstrated better muco-adhesiveness as compared to CS particles (). Hence, it was concluded that better muco-adhesiveness and slower mucociliary clearance observed in case of GC NPs results into the better uptake of FITC–BSA.
Immunological studies
HBsAg-loaded CS and GC NPs were administered i.n. in order to compare the immune-adjuvant effect. All the groups received a primary dose on day 0 and a booster dose on day 21. Serum IgG and secretory IgA were determined by using ELISA before and after booster immunization (). It is observed that intranasal immunization with plain HBsAg resulted into the induction of minimal serum and secretory anti-HBsAg titer (). However, HBsAg associated with NPs demonstrated substantially high antibody titer as compared to the plain HBsAg (p < 0.05). The extent of immune induction in the animals immunized with antigen-loaded CS and GC NPs were substantially different from each other. GC NPs demonstrated much higher antibody titer as compared to CS NPs (p < 0.05). It was also observed that alum-based HBsAg demonstrated strongest serum IgG titer. The magnitude of IgG titer was found in the order of alum-based HBsAg > GC > CS > plain HBsAg ().
Figure 7. Specific anti-HBsAg antibody titer: graph showing (A) anti-HBsAg antibody titer in serum (IgG) and (B) secretion (sIgA). End-point titer is expressed as the reciprocal dilution titers ± SD (n = 6), which gave an optical density (OD) above negative control. The results obtained were compared with CS NPs. Asterisk over bars indicated degree of significance. Where *p < 0.05; **p < 0.01; ***p < 0.001.
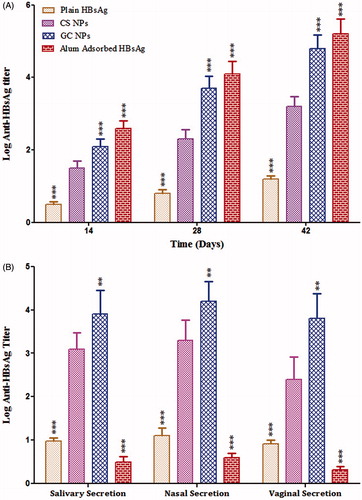
Antibodies play a major role in immunological defense at mucosal surfaces, the surfaces that constitute the largest area of the body exposed to external pathogens. Mucosally applied antibodies have been documented to be protective against virus, bacteria, fungi and parasites that infect humans (Zeitlin et al., Citation1999; Neutra & Kozlowski Citation2006). shows the specific anti-HBsAg sIgA titers in the various secretions of the mice immunized with different formulations. The results of sIgA responses of the various groups of mice were almost similar to the results of the IgG responses. It was observed that sIgA titer was significantly high following i.n. immunization with the antigen-loaded NPs as compared to antigen following primary dose. The immune response was found to rise further on booster dosing with faster kinetics in groups immunized with NPs associated HBsAg as compared to plain HBsAg. Our results indicated that the level of sIgA was substantially higher in case of GC NPs as compared to CS NPs in both local and distal secretions (p < 0.05). In contrast, alum-based HBsAg could induce low sIgA titer in local and distal mucosal secretions.
Estimation of cytokine level
For the complete eradication of viral infection like hepatitis B, a vaccine should generate the complete spectrum of immunity including humoral, mucosal as well as cellular immune response. For confirmation of cellular immunity, the endogenous cytokine levels (IFN-γ, IL-2, IL-4 and IL-6) were determined after 42 d of immunization. IFN-γ and IL-2 are secreted by TH1 cells leading to sequential events for the generation of IgG2a, whereas IL-4 and IL-6 are secreted by TH2 cells generating IgG1 isotype. The CS and GC NPs exhibited significantly higher IL-2 and IFN-γ response as compared to the alum-adsorbed HBsAg, whereas IL-4 and IL-6 responses were dominated by alum-adsorbed HBsAg () indicating the induction of TH2-biased immune response. This indicated that the nasal immunization using NPs-based formulation resulted into the induction of both arms of immunity, i.e. TH1 (cell mediated) and TH2 (humoral).
Figure 8. Cytokine levels of mice immunized with different formulations: graph showing (A) interferon-γ, (B) interleukin-2 (C), interleukin-4 and (D) interleukin-6. Values are expressed as mean ± SD (n = 6). The results obtained were compared with CS NPs. Asterisk over bars indicated degree of significance, where *p < 0.05; **p < 0.01; and ***p < 0.001.
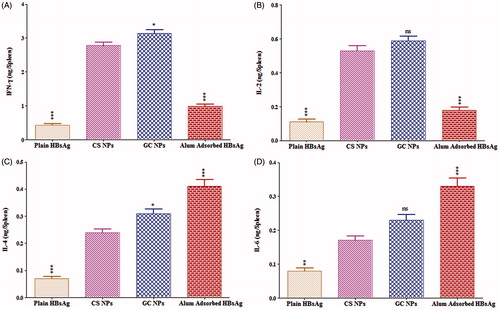
Discussion
Our results showed that HBsAg was successfully associated with GC and CS NPs. SDS-PAGE analysis and relative potency studies indicated that the structure of the incorporated antigen remained intact upon formulation. The particle size has been described to possess effect on the immune adjuvant properties of the vaccine carriers (Köping-Höggård et al., Citation2005; Vila et al., Citation2005). The CS and GC NPs were of similar size, i.e., approximately 200 nm. Therefore, the possibility of role of particles size leading to difference in the immunogenicity can be excluded in our study. Our results indicated that GC NPs exhibited higher surface charge density (Zeta potential) as compared to CS NPs (). It is well-known fact that CS lose its cationic character near to neutral pH range, hence lower zeta potential value was observed for CS NPs when compared with GC NPs (Sadeghi et al., Citation2008). It has been reported that the particles with high positive charge density are able to interact more intensely with the negatively charged sites on the cell membranes and they are able to open the tight junctions between the epithelial cells in NALT (Artursson et al., Citation1994).
Focusing on the various aspects of nasal vaccination; like the clearance from the nasal cavity and the uptake by NALT as function of particle characteristics was also determined to evaluate the immune-adjuvant activity of CS and GC NPs. Our studies indicated that GC NPs demonstrated lower rate of clearance from nasal cavity as compared to CS NPs. This may be explained in terms of the difference in the surface charge densities of GC and CS NPs (). It has been reported that higher Mw polymer efficiently interpenetrate into the mucus glycoproteins and improve the mucoadhesion (Peppas & Buri Citation1985; Smart, Citation2005), the relatively higher Mw of GC can also contribute to the lower rate of clearance of GC NPs from nasal cavity (Synman et al., Citation2002). Nasal uptake studies also confirm that GC NPs demonstrated better nasal uptake as compared to CS NPs ().
The normal mucociliary clearance time in healthy human's nose is about 20 min (Soane et al., Citation1999). It was observed that the mucoadhesive CS and GC NPs could slow down the nasal clearance of antigen from nasal cavity (Delie, Citation1998; Soane et al., Citation1999). Therefore, it is important that the antigen is not released to a large extent before the antigen-loaded NPs pass the nasal mucosal barrier during their residence in the nasal cavity (Slütter et al., Citation2010). The in vitro release studies indicated that CS and GC NPs demonstrated biphasic release behavior, where an initial burst release followed by a sustained release was recorded. The adjuvant effect of particles may be attributed to their ability to form slow release depots, to enhance antigen uptake and presentation by APCs or to enhance the activation of APCs (Slütter et al., Citation2010).
It is generally known that mucosal IgA plays an important role in protection against enteropathogens and viruses both in human and animal models (McNabb & Tomasi, Citation1981; Renegar & Small Citation1991). Moreover, production of HBsAg-specific mucosal IgA antibodies must be important for protection from mucosally transferred HBV (Isaka et al., Citation2001). Immunological studies indicated that primary i.n. dose of HBsAg-loaded GC NPs resulted in strong systemic and mucosal immune responses as compared to CS. In contrast, plain HBsAg antigen failed to induce immune strong immune response at systemic and mucosal immune response. This indicated that Plain HBsAg was poorly immunogenic following nasal delivery. The particulate nature of GC presumably contributes to improve uptake and processing of the encapsulated antigen by APCs as well as a more efficient delivery to peripheral lymph nodes (Jenkins et al., Citation1995; Coombes et al., Citation1996; Jabbal-Gill et al., Citation1999). It has been reported that carriers, which possess better mucoadhesive ability prolongs the nasal residence time of antigen and elicit better immune adjuvant ability (Jaganathan et al., Citation2004; Köping-Höggård et al., Citation2005). Therefore, it was concluded that the strong immune adjuvant ability as observed in case of GC NPs may be a function of its better mucoadhesive ability. In addition to the localization of the antigen in the nasal cavity, the safety profile of i.n. administered CS and GC NPs was assessed, which is of crucial importance in the development of vaccine adjuvants.
It was observed that CS and GC particles demonstrated minimal toxicity to Calu-3 cell line in vitro. It is considered that a cellular immune response may improve vaccine efficacy against viral infections. Therefore, the effect of GC and CS NPs on generation of cytokines was investigated in immunized mice. Our study indicated that i.n. immunization antigen-loaded GC NPs did not affect the sub-typing profile compared to CS NPs.
In summary, GC NPs showed better mucoadhesion, which could prolong the residence time of loaded antigen in the nasal cavity and subsequently resulted into better uptake by M-cells. This consequently results into the prolonged activation of professional APCs in NALT and much efficient immune induction. Based on the above results, it is concluded that GC NPs possess better immune-adjuvant activity as compared to CS NPs.
Conclusion
The physicochemical properties of the polymer such as Mw, surface charge and mucoadhesive ability greatly influence the extent and the type of immune response elicited after nasal vaccination. In our study, GC NPs were found to elicit relatively stronger immune response as compared to that of CS NPs after nasal administration. This may be due to the higher zeta potential and better mucoadhesive ability of the particles, which consequently may result into prolongation of the nasal residence time and promote the uptake by B-cells and activate DCs.
Acknowledgements
We are thankful to All India Institute of Medical Sciences and Punjab University for providing SAIF facility. We are also thankful to Cancer hospital and Research Center, Bhopal, for providing Gamma Scintigraphy facility.
Declaration of interest
The authors report no conflicts of interest. The authors alone are responsible for the content and writing of this article.
References
- Slütter B, Hagenaars N, Jiskoot W. (2008). Rational design of nasal vaccines. J Drug Target 16:1–17
- Jain S, Khomane K, Jain AK, Dani P. (2011). Nanocarriers for transmucosal vaccine delivery. Current Nanoscience 7:160–77
- Vajdy M, O'Hagan DT. (2001). Microparticles for intranasal immunization. Adv Drug Del Rev 51:127–41
- Borges O, Lebre F, Bento D, et al. (2010). Mucosal vaccines: recent progress in understanding the natural barriers. Pharm Res 27:211–23
- Felt O, Buri P, Gurny R. (1998). Chitosan: a unique polysaccharide for drug delivery. Drug Dev Ind Pharm 24:979–93
- Illum L, Farraj NF, Davis SS. (1994). Chitosan as a novel nasal delivery system for peptide drugs. Pharm Res 11:1186–9
- Artursson P, Lindmark T, Davis SS, Illum L. (1994). Effect of chitosan on the permeability of monolayers of intestinal epithelial cells (Caco-2). Pharm Res 11:1358–61
- Kotzé AF, Thanou MM, Luessen HL, et al. (1999). Effect of the degree of quaternization of N-trimethyl chitosan chloride on the permeability of intestinal epithelial cells (Caco-2). Eur J Pharm Biopharm 47:269–74
- Arca HC, Günbeyaz M, Senel S. (2009). Chitosan-based systems for the delivery of vaccine antigens. Expert Rev Vaccines 8:937–53
- Mourya VK, Inamdar NN. (2008). Chitosan-modifications and applications: opportunities galore. React Funct Polymer 68:1013–51
- Werle M, Takeuchi H, Bernkop-Schnürch A. (2009). Modified chitosans for oral drug delivery. J Pharm Sci 98:1643–56
- Gogev S, de Fays K, Versali MF, et al. (2004). Glycol chitosan improves the efficacy of intranasally administrated replication defective human adenovirus type 5 expressing glycoprotein D of bovine herpesvirus 1. Vaccine 22:1946–53
- Trapania A, Sitterberg J, Bakowsky U, Kissel T. (2009). The potential of glycol chitosan nanoparticles as carrier for low water soluble drugs. Int J Pharm 375:97–106
- Mangal S, Pawar D, Garg NK, et al. (2011). Pharmaceutical and immunological evaluation of mucoadhesive nanoparticles based delivery system(s) administered intranasally. Vaccine 29:4953–62
- Sadeghi AM, Dorkoosh FA, Avadi MR, et al. (2008). Preparation, characterization and antibacterial activities of chitosan, N-trimethyl chitosan (TMC) and N-diethylmethyl chitosan (DEMC) nanoparticles loaded with insulin using both the ionotropic gelation and polyelectrolyte complexation methods. Int J Pharm 355:299–306
- Mao S, Bakowsky U, Jintapattanakit A, Kissel T. (2006). Self-assembled polyelectrolyte nanocomplexes between chitosan derivatives and insulin. J Pharm Sci 95:1035–48
- Mao S, Germershaus O, Fischer D, et al. (2005). Uptake and transport of PEG-graft-trimethyl-chitosan copolymer-insulin nanocomplexes by epithelial cells. Pharm Res 22:2058–68
- Jaganathan KS, Singh P, Prabakaran D, et al. (2004). Development of a single-dose stabilized poly(d,l-lactic-co-glycolic acid) microspheres-based vaccine against hepatitis B. J Pharm Pharmacol 56:1243–50
- Pawar D, Mangal S, Goswami R, Jaganathan KS. (2013). Development and characterization of surface modified PLGA nanoparticles for nasal vaccine delivery: effect of mucoadhesive coating on antigen uptake and immune adjuvant activity. Eur J Pharm Biopharm 85:550–9
- Borges O, Borchard G, Verhoef JC, et al. (2005). Preparation of coated nanoparticles for a new mucosal vaccine delivery system. Int J Pharm 299:155–66
- Amidi M, Romeijn SG, Borchard G, et al. (2006). Preparation and characterization of protein-loaded N-trimethyl chitosan nanoparticles as nasal delivery system. J Control Release 111:107–16
- Mathia NR, Timoszyk J, Stetsko PI, et al. (2002). Permeability characteristics of calu-3 human bronchial epithelial cells: in vitro-in vivo correlation to predict lung absorption in rats. J Drug Target 10:31–40
- Mosmann T. (1983). Rapid colorimetric assay for cellular growth and survival: application to proliferation and cytotoxicity assays. J Immunol Methods 65:55–63
- Pawar D, Goyal AK, Mangal S, et al. (2010). Evaluation of mucoadhesive PLGA microparticles for nasal immunization. AAPS J 12:130–7
- He P, Davis SS, Illum L. (1998). In vitro evaluation of the mucoadhesive properties of chitosan microspheres. Int J Pharm 166:75–88
- Debin A, Kravtzoff R, Santiago JV, et al. (2002). Intranasal immunization with recombinant antigens associated with new cationic particles induces strong mucosal as well as systemic antibody and CTL responses. Vaccine 20:2752–63
- Jain S, Singh P, Mishra V, Vyas SP. (2005). Mannosylated niosomes as adjuvantcarrier system for oral genetic immunization against hepatitis B. Immunol Lett 101:41–9
- Sachdeva S, Ahmad G, Malhotra P, et al. (2004). Comparison of immunogenicities of recombinant Plasmodium vivax merozoite surface protein 119 and 42 kDa fragments expressed in Escherichia coli. Infect Immun 70:5775–85
- Prego C, Paolicelli P, Díaz B, et al. (2010). Chitosan-based nanoparticles for improving immunization against hepatitis B infection. Vaccine 28:2607–14
- Amidi M, Romeijn SG, Verhoef JC, et al. (2007). N-trimethyl chitosan (TMC) nanoparticles loaded with influenza subunit antigen for intranasal vaccination: biological properties and immunogenicity in a mouse model. Vaccine 25:144–53
- Florea BI, Meaney C, Junginger HE, Borchard G. (2002). Transfection efficiency and toxicity of polyethylenimine in differentiated Calu-3 and nondifferentiated COS-1 cell cultures. AAPS PharmSci 4:E12. doi: 10.1208/ps040312
- Jaganathan KS, Vyas SP. (2006). Strong systemic and mucosal immune responses to surface-modified PLGA microspheres containing recombinant hepatitis B antigen administered intranasally. Vaccine 24:4201–11
- Soane RJ, Hinchcliffe M, Davis SS, Illum L. (2001). Clearance characteristics of chitosan based formulations in the sheep nasal cavity. Int J Pharm 217:183–91
- Illum L. 2003. Nasal drug delivery–possibilities problems and solutions. J Control Release 87:187–98
- Eldridge JH, Hammond CJ, Meulbroek JA, et al. (1990). Controlled vaccine release in the gut associated lymphoid tissues, I. Orally administered biodegradable microspheres target the Peyer's patches. J Control Release 11:205–14
- Neutra MR, Kozlowski PA. (2006). Mucosal vaccines: the promise and the challenge. Nat Rev Immunol 6:148–58
- Zeitlin L, Cone RA, Whaley KJ. (1999). Using monoclonal antibodies to prevent mucosal transmission of epidemic infectious diseases. Emerg Infect Dis 5:54–64
- Köping-Höggård M, Sánchez A, Alonso MJ. (2005). Nanoparticles as carriers for nasal vaccine delivery. Expert Rev Vaccines 4:185–96
- Vila A, Sánchez A, Evora C, et al. (2005). PLA-PEG particles as nasal protein carriers: the influence of the particle size. Int J Pharm 292:43–52
- Smart JD. (2005). The basics and underlying mechanisms of mucoadhesion. Adv Drug Deliv Rev 57:1556–68
- Peppas NA, Buri PA. (1985). Surface, interfacial and molecular aspects of polymer bioadhesion on soft tissues. J Control Release 2:257–75
- Synman D, Hamman JH, Kotzé JS, et al. (2002). The relationship between the absolute molecular weight and the degree of quaternization of N-trimethyl chitosan chloride. Carbohydr Polym 50:145–50
- Soane RJ, Frier M, Perkins AC, et al. (1999). Evaluation of the clearance characteristics of bioadhesive systems in humans. Int J Pharm 178:55–65
- Delie F. (1998). Evaluation of nano- and microparticle uptake by the gastrointestinal tract. Adv Drug Deliv Rev 34:221–33
- Slütter B, Bal S, Keijzer C, et al. (2010). Nasal vaccination with N-trimethyl chitosan and PLGA based nanoparticles: nanoparticle characteristics determine quality and strength of the antibody response in mice against the encapsulated antigen. Vaccine 28:6282–91
- Slütter B, Bal SM, Que I, et al. (2010). Antigen adjuvant nanoconjugates for nasal vaccination: an improvement over the use of nanoparticles? Mol Pharm 7:2207–15
- McNabb PC, Tomasi TB. (1981). Host defense mechanisms at mucosal surfaces. Annu Rev Microbiol 35:477–96
- Renegar KB, SmallJr PA. (1991). Passive transfer of local immunity to influenza virus infection by IgA antibody. J Immunol 146:1972–8
- Isaka M, Yasuda Y, Mizokami M, et al. (2001). Mucosal immunization against hepatitis B virus by intranasal co-administration of recombinant hepatitis B surface antigen and recombinant cholera toxin B subunit as an adjuvant. Vaccine 19:1460–6
- Coombes AG, Lavelle EC, Jenkins PG, Davis SS. (1996). Single dose, polymeric, microparticle-based vaccines: the influence of formulation conditions on the magnitude and duration of the immune response to a protein antigen. Vaccine 14:1429–38
- Jabbal-Gill I, Lin W, Jenkins P, et al. (1999). Potential of polymeric lamellar substrate particles (PLSP) as adjuvants for vaccines. Vaccine 18:238–50
- Jenkins PG, Coombes AG, Yeh MK, et al. (1995). Aspects of the design and delivery of microparticles for vaccine applications. J Drug Target 3:79–81