Abstract
The objective of this work was to formulate paliperidone palmitate-loaded d-alpha-tocopheryl polyethylene glycol 1000 succinate (vitamin E TPGS or TPGS) micelles for improved antipsychotic effect during short-term management of psychotic disorders. Vitamin E TPGS micelles containing paliperidone palmitate were prepared by the solvent casting method and control paliperidone palmitate formulations were prepared by simple sonication method. The prepared micelles and control paliperidone palmitate formulations were evaluated for different parameters. Particle sizes of prepared micelles, control paliperidone palmitate formulations were determined at 25 °C by dynamic light scattering technique and external surface morphology was determined by transmission electron microscopy analysis. The encapsulation efficiency was determined by spectrophotometery. In-vitro release studies of micelles and control formulations were carried out by dialysis bag diffusion method. The particle sizes of the paliperidone palmitate-loaded TPGS micelles were 26.5 nm. About 92% of drug encapsulation efficiency was achieved with micelles. The drug release from paliperidone palmitate-loaded TPGS micelles was sustained for more than 24 h with 40% of drug release. The TPGS product, i.e. paliperidone palmitate-loaded micelles, resulted in nano-sized delivery, solubility enhancement and permeability of the micelles which provided an improved and prolonged anti-psychotic effect in comparison to control paliperidone palmitate formulation.
Introduction
For many years, it has been very difficult to treat brain disorder due to presence of the blood–brain barrier (BBB). To target the drugs into the brain is one of the most challenging areas of research for drug delivery scientists. The BBB protects the brain from exogenous materials and maintains the internal homeostasis of brain tissues, therefore direct transport of drug from peripheral blood flow to brain tissue is a major key problem for treating brain disorders (Béduneaua et al., Citation2007; Wilson et al., Citation2008; Kulkarni & Feng, Citation2011). A variety of central nervous system (CNS) drugs, especially antipsychotics, are unsuccessful as they cannot be effectively transported to the brain tissues (e.g. across the BBB). Only those drugs which are either highly lipophilic or small enough in size can penetrate the BBB. Since the efficiency of BBB penetration of antipsychotic drug is low, higher doses are needed to achieve a desired therapeutic effect, which usually produce unwanted side-effects (Kabanov et al., Citation1989; Alam et al., Citation2010; Gan et al., Citation2010). To enhance the BBB permeability of drug, many approaches have been used including osmotic opening of tight junctions, use of prodrugs or carrier systems such as antibodies, liposomes, and nanoparticles (Kreuter, Citation2001). Further, sustained release of antipsychotics by parenteral route provides more reliable delivery of medication and an improved pharmacokinetic profile (bioavailability, biodistribution, penetration across the BBB, etc.) (Kreuter, Citation2001; Muthu & Singh, Citation2008).
Now-a-days to overcome various problems associated with brain disorder treatment, researchers have focussed on development of a nanotechnology-based drug delivery system for targeting the drug into the brain. Nanotechnology, defined as the development of nano-sized systems in the size range of 1–1000 nm, is a fast growing field as a research area over worldwide (Sahoo & Labhasetwar, Citation2003; Paul & Robeson, Citation2008; Bharali et al., Citation2010). Application of nanotechnology in medicine for targeted drug delivery is spreading rapidly in neuroscience and neurological surgery specifically for therapy of brain injuries. They offer many advantages over other drug-delivery systems like enhanced stability of labile drugs, controlled as well as targeted drug release and enhanced drug bioavailability, and also due to their nanosized, they can easily cross permeability barriers. Currently, many nanocarriers are under investigation for drug delivery to the brain, e.g., polymeric nanoparticles, nanoconjugates, solid lipid nanoparticles, gold nanoparticles, carbon nanotubes, micelles and liposomes (Muthu & Singh, Citation2009; Muthu & Feng, Citation2009, Citation2010; Jalali et al., Citation2011).
Among all nanocarriers, micelles have attracted much attention as a targeted drug carrier in recent years. Micelles are amphiphilic spherical nanostructures consist of a hydrophobic core and a hydrophilic shell. It has many advantages over other drug carriers due to its dynamic structure which provide it much higher thermodynamic stability and kinetic stability. Its hydrophilic block stabilizes the micelle in an aqueous environment for intravenous delivery and the hydrophobic core stores a payload of drug for therapy (Muthu et al., Citation2009a; Kim et al., Citation2010). They cannot be easily detected and eliminated by reticuloendothelial system (RES) due to their small scale dimension (diameter less than 50 nm) and their hydrophilic shell, thus shows enhanced permeability and retention effect (EPR effect). Also, in comparison to other nanosystem, micelles allows easy encapsulation of poorly soluble drugs in the hydrophobic cores, thus increases the water-solubility, drug loading capacity and reduce toxicity due to prolonged circulation in blood and enhanced accumulation of drug in diseased tissue (Gao et al., Citation2008; Blanco et al., Citation2009; Mi et al., Citation2011).
d-alpha-tocopheryl polyethylene glycol 1000 succinate (TPGS) is as an amphiphilic copolymer for preparation of micelles because of its valuable advantages. TPGS is a water-soluble derivative of natural vitamin E, which has amphiphilic structure comprising lipophilic alkyl tail and hydrophilic polar head portion. Thus, it may act as micelle forming material for drug delivery. It is non-ionic and highly hydrophilic in nature. Due to its highly hydrophilic nature, it becomes highly saturated with water molecules which results in enhanced stability of TPGS-coated structures, enhanced cellular uptake, prolonged blood circulation and hence enhanced bioavailability. Its bulky structure and large surface area make it to be an excellent emulsifier, solubilizer, bioavailability enhancer of hydrophobic drugs (Repka & McGinity, Citation2000; Muthu et al., Citation2011, Citation2012a,Citationb; Mi et al., Citation2012; Zhang et al., Citation2012). The lipophilic portion of TPGS allows poorly solubilization of poorly soluble drug in the micelles which may lead to more effective treatment. It also increases the drug permeability across the BBB and enhances the absorption of the drug by inhibiting the P-glycoprotein (Mu et al., Citation2002; Zhang et al., Citation2007; Guo et al., Citation2013). In one study, Muthu et al., Citation2012a have prepared docetaxel-loaded TPGS micelles which showed three times increase of cytotoxicity than control docetaxel in brain cancer cells. Also, micelles of docetaxel were found to be present longer time in blood circulation in comparison to control docetaxel (Muthu et al., Citation2012a).
Paliperidone palmitate is the palmitate ester of paliperidone which is a major active metabolite of risperidone. Chemically, it is (±)-3-[2-[4-(6-fluoro-1,2-benzisoxazol-3-yl)-1-piperidinyl]ethyl] - 6,7,8,9-tetrahydro-2-methyl-4-oxo-4H-pyrido [1,2-a] pyrimidin-9-yl hexadecanoate. Paliperidone palmitate is a new generation antipsychotic. Therapeutic activity of paliperidone palmitate is mediated through a combination of central dopamine Type 2 (D2) and serotonin Type 2 (5HT2A) receptor antagonism. It is practically insoluble in aqueous media over a broad pH range. The paliperidone palmitate was found to have large volume of distribution (Vd) because it is the substrate of P-glycoprotein (P-gp) efflux pump, which caused lower drug accumulation in brain cells and significant toxicity to normal tissues (Nussbaum & Stroup, Citation2012). It is commercially available for the treatment of schizophrenia as a once monthly depot intramuscular injection (long-acting) in suspension form (trade-name is Invega Sustenna). However, it cannot be used during short-term management of psychotic disorders. The Invega Sustenna formulation contains solublizers such as polysorbate 20 and polyethylene glycol 4000 to solublize paliperidone palmitate (Nussbaum & Stroup, Citation2012). TPGS could be an alternative and efficient for this purpose (Muthu et al., Citation2012a).
Therefore, the purpose of present study was to prepare and evaluate paliperidone palmitate-loaded TPGS micelles for improved and prolonged antipsychotic effect in comparison to control paliperidone palmitate formulation during short-term management of psychotic disorders. These micelles were prepared by solvent casting (SC) method and characterized by various methods. The in-vivo efficacy of prepared micelles formulation and control paliperidone palmitate formulation were studied by administering them intramuscularly to apomorphine treated mice. The extrapyramidal side effects (EPS) (i.e. catalepsy) of the formulation and control paliperidone palmitate formulations were also studied.
Materials and methods
The drug of choice in the present investigation, paliperidone palmitate was obtained from Dr. Reddy's Laboratory, Hyderabad, India as gift sample. d-alpha-tocopheryl polyethylene glycol 1000 succinate (TPGS) was obtained from Antares Health Products Inc., St. Charles, IL, USA as gift sample. Dialysis bag with a 12 000 molecular weight cut-off was purchased from Sigma-Aldrich Chemicals., New Delhi, India. All other chemicals were of analytical grade.
Preparation of TPGS micelles
Solvent casting (SC) method
TPGS micelles were prepared according to the SC method (Muthu et al., Citation2012a) (). Paliperidone palmitate-loaded micellar nanosuspension was prepared with 150 mg of TPGS (PPT-150). A 3 mg of paliperidone palmitate and 150 mg of TPGS were dissolved in 4 ml of chloroform. From this mixture chloroform was evaporated using a Buchi Rotary Evaporator R-210 Advanced, Switzerland at 37 °C and formed a thin film of drug dispersed TPGS. Drug-loaded micelles were formed by resuspending the drug-loaded film in 0.1M phosphate buffered saline (PBS), pH 7.4. The mixture was incubated in orbital water bath shaker at 37 °C for 48 h. The excess non-incorporated drug was separated by filtration (using 0.22-µm filter) before characterization.
The two control formulations were prepared by dispersing paliperidone palmitate with TPGS (PPTPGS) or without TPGS (PPT) under bath sonication for 10 min ().
Table 1. Formulas of preparations.
Micelle characterization
Particle size and surface morphology
Particle sizes of control paliperidone palmitate formulations without (PPT) or with (PPTPGS) 150 mg of TPGS and paliperidone palmitate-loaded TPGS micelles (PPT-150) were determined at 25 °C by dynamic light scattering technique (Delsa Nano C-Beckmann Coulter). Samples were scattered (658 nm) at an angle of 165°. Data were fitted by the method of inverse “Laplace transformation” and CONTIN. Each value reported is the average of three measurements.
Surface morphology was studied by a transmission electron microscopy (TEM) system (Philips CM-12., Fullerton, CA, USA). Samples of TPGS micelles (paliperidone palmitate loaded) was prepared by placing one drop on a copper grid and dried under vacuum pressure. The dried micelles being stained with 1% phosphotungstic acid for 30 s was finally examined.
Determination of drug encapsulation of TPGS micelles
The encapsulation efficiency of micelle was determined by UV spectrophotometer (Shimadzu 1800, Japan). A 3-ml aliquot of micelles formulation was evaporated to dryness by rotary evaporator under reduced pressure at 35 °C. The residue was dissolved in 20 ml of methanol. Further 1 ml aliquot of this solution was further diluted to 10 ml with methanol. Then light absorption was measured at 278 nm using spectrophotometer. All samples were done in triplicate.
The calculation was performed as follows:
In-vitro drug release studies
The dialysis bag diffusion technique was used to study the in-vitro drug release of drug-loaded micelles (Muthu et al., Citation2012a). Totally, 2 ml of drug-loaded micellar nanosuspension or control paliperidone palmitate formulations (equivalent to 350 μg/ml of paliperidone palmitate) were placed in the dialysis bag (cellulose membrane, molecular weight cut-off 12 000 D), hermetically sealed and immersed into 50 ml of phosphate buffered saline (pH 7.4). The entire system was kept at 37 ± 0.5 °C with continuous shaking at 100 rpm/min. Samples were withdrawn from the receptor compartment at predetermined time intervals and replaced by fresh medium. The amount of drug released was determined by UV spectrophotometer at 278 nm.
Animals and in-vivo evaluation of the paliperidone palmitate formulations
Swiss albino mice (23±2 g) (Institute of Medical Sciences, Banaras Hindu University, India) were used to study the effect of paliperidone palmitate formulations on the controlled inhibition of apomorphine-induced climbing and sniffing. EPS liability of the paliperidone palmitate formulations were studied using catalepsy in mice. Before experimentation they were kept in groups of six and fed with standard diet with water ad libitum. All experimental procedures were reviewed and approved by the animal and ethics review committee of Institute of Medical Sciences, Banaras Hindu University, India (Dean/12-13/CAEC/38).
Apomorphine-induced climbing and sniffing
Animals were divided into three groups of five animals (n = 5). Two groups were administered with two test compounds, i.e. control paliperidone palmitate formulation (PP) and paliperidone palmitate-loaded micelles (PPT-150) at a dose of 4 mg/kg via intramuscular (i.m) route with a 0.5 cm3 U40 insulin syringe equipped with 28-g1/2 needle} and one group as a saline control. The paliperidone palmitate formulations were administered to mice for delivering approximately 4 mg/kg of drug dose per day. This dose was selected based on previous animal studies of paliperidone palmitate and risperidone (i.e. parent drug of paliperidone palmitate) (Muthu et al., Citation2009b; Hoy et al., Citation2010).
The method of Muthu et al., Citation2009b was used for behavioral observation, combined with a time-sampling procedure. Each animal was placed into cylindrical wire-mesh cages (diameter, 14 cm; height, 13 cm; mesh size, 3 mm) and allowed to adapt for 60 min. Thereafter, mice were injected six times, first with test compounds or saline via i.m. route, followed by subcutaneous (s.c.) injection of apomorphine (2.5 mg/kg) at different time intervals (1 h, 4 h, 8 h, 12 h and 24 h later to first injection). First injection was test compounds or saline and 2nd to 6th injections were apomorphine injection. Behavioral observations were made from 11 to 20 min after the each apomorphine injection: animals were observed for 10 s every minute for the presence or absence of climbing (i.e. all fours paws on the cage, above the floor). Sniffing was scored when the animal showed uninterrupted sniffing for at least 3 s during this 10 s sampling period. The mice were observed for climbing behavior and scored as follows: 0=absence of four paws on the cage; 1 = presence of four paws on the cage. The mice were observed for sniffing behavior and scored as follows: 0=interrupted sniffing; 1=uninterrupted sniffing for at least 3 s. Thus, the score for climbing or sniffing could vary from 0 to 10 for the entire observation period.
Catalepsy
Catalepsy studies were carried out in Swiss albino mice. They were divided into three groups of five animals (n = 5) and administered with two test compounds, i.e. control paliperidone palmitate formulation (PP) and paliperidone palmitate-loaded micelles (PPT-150) at a dose of 4 mg/kg via i.m. route with a 0.5 cm3 U40 insulin syringe equipped with 28-g1/2 needle} and one group as a saline control.
Catalepsy procedure was measured using the bar test 1 h, 4 h, 8 h, 12 h and 24 h after the i.m injection (test compounds or saline): the forelimbs were placed on a cylindrical bar (diameter, 0.4 cm; 3.5 cm above the table) and the time during which both forelimbs remained on the bar was recorded up to a maximum of 30 s.
Statistical analysis
Results are given as mean ± standard deviation (S.D). In-vivo data were analyzed with a one-way ANOVA followed by Dunnett's test (control and test compounds or between the test compounds). Differences are considered significant at a level of p < 0.05.
Results and discussion
Particle size, surface morphology and encapsulation efficiency of TPGS micelles
The sizes of control paliperidone palmitate formulation without TPGS (PP), with TPGS (PPTPGS) and paliperidone palmitate-loaded TPGS micelles (PPT-150) prepared by SC method were 2316.2 ± 597.0 nm, 1769.7 ± 525.3 nm and 26.5 ± 4.8 nm, respectively (). The particle size of the paliperidone palmitate in formulation PP was significantly reduced in formulation PPTPGS owing to the solubilization property of the TPGS (i.e. from 2316.2 nm to 1769.7 nm). During the micelle formation, the size was further reduced and micelles were formed in size less than 50 nm (i.e. formulation PPT-150). The particle size distribution of micelles is showed in . Here results of particle sizes are indicating that paliperidone palmitate-loaded TPGS micelles were formed by SC method.
Figure 2. Particle size results of control formulations of paliperidone palmitate and paliperidone palmitate-loaded TPGS micelles. PPT-150, Paliperidone palmitate micelles formulation; PPTPGS, Paliperidone palmitate control with free TPGS; PP, Paliperidone palmitate control; 150: TPGS concentration.
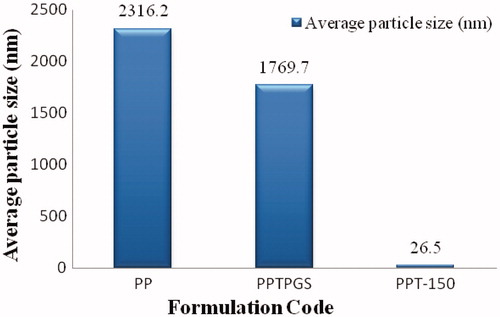
Figure 3. Histogram showing particle size and size distribution of paliperidone palmitate-loaded TPGS micelles (PPT-150). PPT-150, Paliperidone palmitate micelles formulation; PPT-150, Paliperidone palmitate micelles formulation.
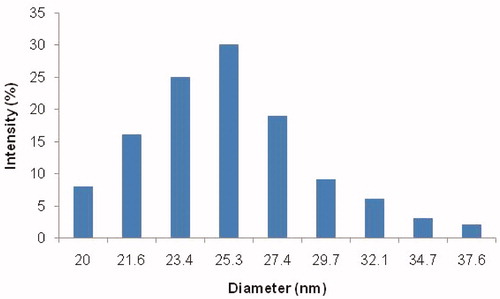
The external morphological study using TEM revealed that paliperidone palmitate-loaded TPGS micelles (PPT-150) were spherical in shape ().
Figure 4. TEM micrograph of (A) and (B) paliperidone palmitate-loaded TPGS micelles (PPT-150) showing multiple particles with 100-nm scale.
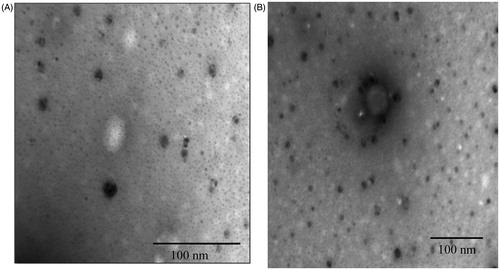
The drug encapsulation efficiency of paliperidone palmitate-loaded TPGS micelles prepared by SC method was found as 92.61 ± 2.5%.
In-vitro drug release studies
shows the percentage release of percentage release of control formulation of paliperidone palmitate without TPGS (PP), with TPGS (PPTPGS) and paliperidone palmitate-loaded TPGS micelles (PPT-150) in phosphate buffer saline (pH 7.4). The control formulation without TPGS (PP) showed less than 6% of drug release after 24 h. The percentage release of control formulation of paliperidone palmitate with TPGS (PPTPGS) showed release about 23% after 24 h. However, micellar nanosuspension (PPT-150) showed improvement in dissolution profile with sustained release for more than 24 h. After 24 h of dialysis in phosphate buffered saline (pH 7.4), the percentages of paliperidone palmitate released was 40 % (). The improvement in the dissolution profile of formulation PPT-150 is owing to the availability of larger surface area of nano-sized micelles in comparison to control paliperidone palmitate formulations (i.e. PP and PPTPGS).
Figure 5. In-vitro drug release study of control formulations of paliperidone palmitate and paliperidone palmitate-loaded TPGS micelles in phosphate buffered saline (pH 7.4) (n = 3). PPT-150, Paliperidone palmitate micelles formulation; PPTPGS, Paliperidone palmitate control with free TPGS; PP, Paliperidone palmitate control; 150, TPGS concentration.
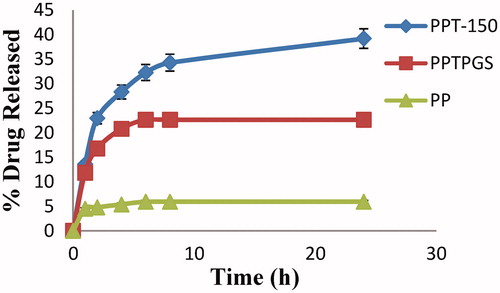
In-vivo evaluation of the paliperidone palmitate formulations
Antagonism of apomorphine-induced climbing and sniffing
In-vivo pharmacodynamic evaluation of paliperidone palmitate-loaded TPGS micelles was carried out with an animal model – Swiss albino mice (). The in-vivo effect of paliperidone palmitate administration as micelles (PPT-150) and the control paliperidone palmitate formulation (PP) are presented in ( and ).
Figure 6. Antipsychotic effect of paliperidone palmitate-loaded TPGS micelles (PPT-150) (A) mice showing normal behavior (B) apomorphine-induced climbing and sniffing behavior.
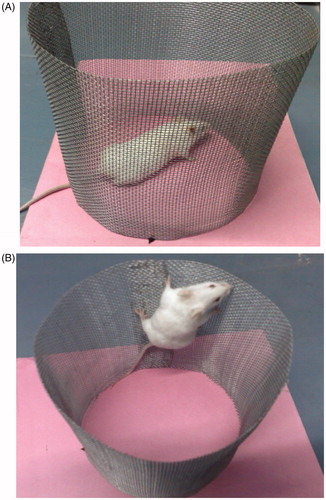
Table 2. Effect of paliperidone palmitate-loaded TPGS micelles on inhibition of apomorphine-induced climbing in mice.
Table 3. Effect of paliperidone palmitate-loaded TPGS micelles on inhibition of apomorphine-induced sniffing in mice.
The paliperidone palmitate control did not significantly (p > 0.05) inhibited apomorphine induced climbing and sniffing behavior up to 8 h when compared to saline control ( and ). However, slight inhibition of apomorphine-induced climbing and sniffing behavior was observed after drug treatment from 1 h and it was significant at 24 h (p < 0.05).
Paliperidone palmitate-loaded TPGS micelles significantly (p < 0.05) inhibited apomorphine induced climbing and sniffing behavior up to 24 h compared to saline control ( and ). Compared with paliperidone palmitate control, paliperidone palmitate-loaded TPGS micelles showed significant (p < 0.05) inhibition (from 1 h to 24 h) of climbing and sniffing ( and ). Here, paliperidone palmitate control showed lesser antipsychotic efficacy due to its poor solubility profile. The solubilized delivery of paliperidone palmitate from TPGS micelles sustained the activity with higher bioavailability during 1 h to 24 h which showed its improvement in performance over the paliperidone palmitate control.
Catalepsy
The EPS liability of paliperidone palmitate administration as micelles (PPT-150) and the control paliperidone palmitate formulation (PP) are presented in ( and ). The paliperidone palmitate control showed significant (p > 0.05) catalepsy at 8 h and 24 h when compared to saline control (). Further, paliperidone palmitate-loaded TPGS micelles (PPT-150) showed significant (p < 0.05) catalepsy at 1 h, 4 h and 8 h compared to paliperidone palmitate control (). The paliperidone palmitate control did not show any antipsychotic activity (during 1 h to 8 h) and catalepsy (during 1 h to 4 h) owing to the poor absorption/release of formulation from the site of administration (i.e. i.m. site). There was significant (p > 0.05) reduction in the catalepsy (using Dunnett's test) for paliperidone palmitate-loaded TPGS micelles (PPT-150) compared to paliperidone palmitate control at 24 h (). The possible reason for the reduction of catalepsy at 24 h owing to modified release pattern of paliperidone palmitate as TPGS micelles. Also, reduction in catalepsy at 24 h from paliperidone palmitate-loaded TPGS micelles (PPT-150) shows a fewer toxic potential of the micellar formulation in comparison to control paliperidone palmitate formulation (PP).
Figure 7. EPS of paliperidone palmitate-loaded TPGS micelles (PPT-150) (A) mice showing normal behavior (B) drug-induced catalepsy behavior.
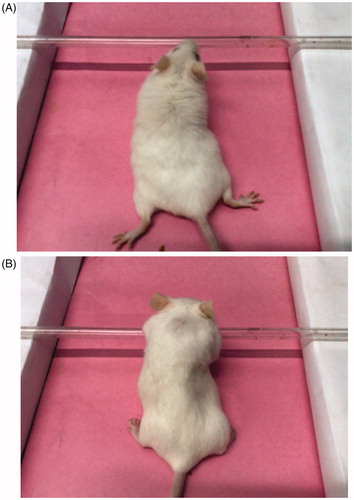
Table 4. Effect of paliperidone palmitate-loaded TPGS micelles on reduction in the catalepsy in mice.
Here, the pharmacodynamic studies of paliperidone palmitate and its formulations were carried out to find antipsychotic effect and any adverse effect after the i.m. administration. Atypical antipsychotic drugs are tested for the inhibition of apomorphine-induced climbing and sniffing (for antipsychotic activity) and catalepsy test (for EPS liability) as these tests have high predictive accuracy (Muthu et al., Citation2009b). This model is predictive of efficacy against the positive symptoms of psychosis (Protais et al., Citation1976) and demonstrates prolonged in-vivo antagonist activity at dopamine D2 receptors.
Conclusion
The paliperidone palmitate-loaded TPGS micelles showed great advantage over control formulation of paliperidone palmitate. This study confirms that SC method is easy, reproducible and low-cost method for the preparation of paliperidone palmitate-loaded TPGS micelles. The drug-loaded micelle has improved antipsychotic effect and modified the adverse effect as miceller formulation. The co-dispersion of paliperidone palmitate with TPGS in micelles leads to maximum encapsulation as the TPGS self-assembled into nano containers of paliperidone palmitate. It shows a significant reduction in particle size of drug-loaded micelles when compared to paliperidone palmitate with TPGS as simple suspension. TEM analysis showed the nano range of TPGS micelles. In-vitro drug release profiles revealed the continuous sustain release property of TPGS micelles. Finally, sustained antipsychotic effect and modified adverse release pattern of paliperidone palmitate micelles have showed the utility of the micelles for the effective and safer treatment of psychosis during short-term management of psychotic disorders. To treat brain disorders using nanocarriers will become more effective with fewer side effects. Nanomicelles could be one of the most promising candidates to solve the problems related to brain disorder, which may include sustained release, safety and targeted delivery. In future, TPGS micelles with brain targeting/imaging can be developed for potential antipsychotic therapy with fewer adverse effects (Muthu et al., Citation2009a; Muthu et al., 2014; Muthu & Wilson, Citation2010).
Acknowledgements
Author Dr. M. S. Muthu acknowledges the Banaras Hindu University, Varanasi, India, for providing XI Plan Research Grant (Scheme No. 4121) and DST-PURSE Grant for the research work during years of 2011–2012 and 2013–2014, respectively. We also acknowledge Electron Microscopy Facility, Department of Anatomy, Institute of Medical Sciences, Banaras Hindu University, Varanasi, India, in carrying out TEM analysis of samples.
Declaration of interest
This study was supported by XI Plan Research Grant (Scheme No. 4121) and DST-PURSE Grant (Banaras Hindu University) for the research work during years of 2011–2012 and 2013–2014, respectively. The authors report no conflicts of interest. The authors alone are responsible for the content and writing of the article.
References
- Alam MI, Bega S, Samad A, et al. (2010). Strategy for effective brain drug delivery. Eur J Pharm Sci 40:385–403
- Béduneaua A, Saulnier P, Benoit JP. (2007). Active targeting of brain tumors using nanocarriers. Biomaterials 28:4947–67
- Bharali DJ, Mousa SA. (2010). Emerging nanomedicines for early cancer detection and improved treatment: current perspective and future promise. Pharmacol Ther 128:324–35
- Blanco E, Kessinger CW, Sumer BD, Gao J. (2009). Multifunctional micellar nanomedicine for cancer therapy. Exp Biol Med 234:123–31
- Gan CW, Feng SS. (2010). Transferrin-conjugated nanoparticles of Poly (lactide) - d-α-tocopheryl polyethylene glycol succinate diblock copolymer for targeted drug delivery across the blood brain barrier. Biomaterials 31:7748–57
- Gao Y, Li LB, Zhai G. (2008). Preparation and characterization of Pluronic/TPGS mixed micelles for solubilization of camptothecin. Coll Surf B: Biointerf 64:194–9
- Guo Y, Luo J, Tan S, et al. (2013). The applications of Vitamin E TPGS in drug delivery. Eur J Pharm Sci 49:175–86
- Hoy SM, Scott LJ, Keating GM. (2010). Intramuscular paliperidone palmitate. CNS Drugs 24:227–44
- Jalali N, Moztarzadeh F, Mozafari M, et al. (2011). Surface modification of poly (lactide-co-glycolide) nanoparticles by d-α-tocopheryl polyethylene glycol 1000 succinate as potential carrier for the delivery of drugs to the brain. Coll Surf A: Physicochem Eng Aspects 392:335–42
- Kabanov AV, Chekhonln VP, Alakhov VY, et al. (1989). The neuroleptic activity of haloperidol increases after its solubilization in surfactant micelles: micelles as microcontainers for drug targeting. FEBS Lett 258:343–5
- Kim S, Shi Y, Kim JY, et al. (2010). Overcoming the barriers in micellar drug delivery: loading efficiency, in vivo stability, and micelle–cell interaction. Expert Opin Drug Deliv 7:49–62
- Kreuter J. (2001). Nanoparticulate systems for brain delivery of drugs. Adv Drug Deliv Rev 47:65–81
- Kulkarni SA, Feng SS. (2011). Effects of surface modification on delivery efficiency of biodegradable nanoparticles across the blood–brain barrier. Nanomedicine (Lond) 6:377–94
- Mi Y, Liu Y, Feng SS. (2011). Formulation of docetaxel by folic acid-conjugated D-a-tocopheryl polyethylene glycol succinate 2000 (Vitamin E TPGS2k) micelles for targeted and synergistic chemotherapy. Biomaterials 32:4058–66
- Mi Y, Zhao J, Feng SS. (2012). Vitamin E TPGS prodrug micelles for hydrophilic drug delivery with neuroprotective effects. Int J Pharm 438:98–106
- Mu L, Feng SS. (2002). Vitamin E TPGS used as emulsifier in the solvent evaporation / extraction technique for fabrication of polymeric nanospheres for controlled release of paclitaxel (Taxol). J Control Rel 80:129–44
- Muthu MS, Feng SS. (2009). Pharmaceutical stability aspects of nanomedicines. Nanomedicine (Lond) 4:857–60
- Muthu MS, Feng SS. (2010). Nanopharmacology of liposomes developed for cancer therapy. Nanomedicine (Lond) 5:1017–19
- Muthu MS. Leong DT, Mei L, Feng SS. (2014). Nanothernaostics: Application and further development of nanomedicine strategies for advanced theranostics. Theranostics 4: 660–77
- Muthu MS, Kulkarni SA, Xiong J, Feng SS. (2011). Vitamin E TPGS coated liposomes enhanced cellular uptake and cytotoxicity of docetaxel in brain cancer cells. Int J Pharm 421:332–40
- Muthu MS, Kulkarni SA, Liu Y, Feng SS. (2012a). Development of docetaxel-loaded vitamin E TPGS micelles: formulation optimization, effects on brain cancer cells and biodistribution in rats. Nanomedicine (Lond) 7:353–64
- Muthu MS, Kulkarni SA, Raju A, Feng SS. (2012b). Theranostic liposomes of TPGS coating for targeted co-delivery of docetaxel and quantum dots. Biomaterials 33:3494–501
- Muthu MS, Rajesh CV, Mishra A, Singh S. (2009a). Stimulus responsive targeted nanomicelles for effective cancer therapy. Nanomedicine (Lond) 4:657–67
- Muthu MS, Rawat MK, Mishra A, Singh S. (2009b). PLGA nanoparticle formulations of risperidone: preparation and neuropharmacological evaluation. Nanomedicine 5:323–33
- Muthu MS, Singh S. (2008). Studies on biodegradable polymeric nanoparticles of risperidone: in vitro and in vivo evaluation. Nanomedicine (Lond) 3:305–19
- Muthu MS, Singh S. (2009). Targeted nanomedicines: effective treatment modalities for cancer, AIDS and brain disorders. Nanomedicine (Lond) 4:105–18
- Muthu MS, Wilson B. (2010). Multifunctional radionanomedicine: a novel platform for effective cancer imaging and therapy. Nanomedicine (Lond) 5:169–71
- Nussbaum AM, Stroup TS. (2012). Paliperidone palmitate for schizophrenia. Cochrane Database Syst Rev 6:CD008296
- Paul DR, Robeson LM. (2008). Polymer nanotechnology: nanocomposites. Polymer 49:3187–204
- Protais P, Costentin J, Schwartz JC. (1976). Climbing behaviour induced by apomorphine in mice: a simple test for the study of dopamine receptors in striatum. Psychopharmacology 50:1–6
- Repka MA, McGinity JW. (2000). Influence of Vitamin E TPGS on the properties of hydrophilic films produced by hot-melt extrusion. Int J Pharm 202:63–70
- Sahoo SK, Labhasetwar V. (2003). Nanotech approaches to drug delivery and imaging. Drug Discov Today 8:1112–20
- Wilson B, Samanta MK, Santhi K, et al. (2008). Poly(n- butylcyanoacrylate) nanoparticles coated with polysorbate80 for the targeted delivery of rivastigmine into the brain to treat Alzheimer's disease. Brain Res 1200:159–68
- Zhang Z, Lee SH, Feng SS. (2007). Folate-decorated poly(lactide-co-glycolide)- vitamin E TPGS nanoparticles for targeted drug delivery. Biomaterials 28:1889–99
- Zhang Z, Tan S, Feng SS. (2012). Vitamin E TPGS as a molecular biomaterial for drug delivery. Biomaterials 33:4889–906