Abstract
The aim of the present research work was to investigate the potential of polyamidoamine (PAMAM) dendrimers as oral drug delivery carriers for quercetin, a Biopharmaceutical Classification System (BCS) class II molecule. The aqueous solubility of quercetin was investigated in different generations of dendrimers, i.e. G0, G1, G2 and G3, with varying concentrations (0.1, 0.5, 1, 2 and 4 µM). Then, it was successfully incorporated in PAMAM dendrimers and they were characterized for incorporation efficacy, nature of nanoformulations, size, size distribution, surface morphology and stability. In vitro release characteristics of quercetin from all quercetin-PAMAM complexes were studied at 37 °C in phosphate buffer saline (PBS; pH 7.4). Furthermore, the efficacy of quercetin-loaded PAMAM dendrimer was assessed by pharmacodynamic experiment, namely, a carrageenan-induced paw edema model to evaluate the acute activity of this nanocarrier in response to inflammation. It was observed that both generation and the respective concentrations of PAMAM dendrimers showed potential positive effects on solubility enhancement of quercetin. All the quercetin-PAMAM complexes were found to be in nanometeric range (<100 nm) with narrow polydispersity index. In vitro study revealed a biphasic release pattern of quercetin which was characterized by an initial faster release followed by sustained release phase and pharmacodynamic study provided the preliminary proof of concept about the potential of quercetin-PAMAM complexes. The study concludes that the dendrimer-based drug delivery system for quercetin has enormous potential to resolve the drug delivery issues associated with it.
Introduction
Plant-derived products have been preferred throughout human history for treatment of various ailments since ancient times. Their great therapeutic potential and fewer adverse effects have increased the usage of herbal products widely all over the world (Aqil et al., Citation2013). Flavonoids are one of those essential phytochemicals that has drawn the considerable interest in research community because of their potential beneficial effects on human health. These secondary metabolites occur ubiquitously in foods of plant origin and are chemically polyhydroxylated, i.e. “polyphenols” which are known to exhibit antioxidant property essentially and they are mainly responsible for various therapeutic and biological effects (Masella et al., Citation2001; Kris-Etherton & Keen, Citation2002).
Quercetin (3, 5, 7, 3′, 4′-pentahydroxyflavone) is a major representative of flavonoid family that has been categorized under flavonols and is a common dietary component. Humans have been exposed to quercetin since ancient times, at least as long as the availability of onion, apple, black tea and grapes are known. This molecule is reported to exhibit antioxidant (Arora et al., Citation1998; Silva et al., Citation2002), cardioprotective (Shoskes, Citation1998; Hubbard et al., Citation2004), anti-ulcer (Mizui et al., Citation1987; Alarcon de la Lastra et al., Citation1994; Beil et al., Citation1995), gene regulation (Carluccio et al., Citation2007) and anti-inflammatory (Kim et al., Citation1998; Lee et al., Citation2010) effects. Recent reports on the potential of quercetin to act as anti-cancer and neuroprotective agent have generated a considerable scientific interest in this molecule (Dajas, Citation2012). Despite the wide pharmacological profile, the only thing that limits the usage of this phytochemical is low solubility and bioavailability subsequently. Several nanocarriers, namely liposomes (Priprem et al., Citation2008), cyclodextrins (Jullian et al., Citation2007), solid lipid nanoparticles (Wu et al., Citation2008; Li et al., Citation2009; Kumari et al., Citation2012), polymeric micelles (Khonkarn et al., Citation2011) and solid dispersions (Kakran et al., Citation2011), have been developed to resolve the delivery issues of quercetin. However, the desirable characteristics such as tissue specificity, product stability and solubility for quercetin delivery have not been attained simultaneously with these reported nanosystems. In view of same, there is an urgent need for the development of suitable carriers for this phytochemical with stable structure and high drug loading.
Dendrimers, owing to the exclusive properties of nano-scale size (Cheng et al., Citation2008b), polyvalancy (Patton et al., Citation2006), biocompatibility (Kobayashi et al., Citation2001) and monodispersity (Jackson et al., Citation1998), have been recognized as efficient drug delivery carriers. These moieties comprise three distinct domains, i.e. central core, surrounding generations and terminal functional groups (Tomalia et al., Citation1985). They have been considered as highly branched monomolecular micelles that are quite stable as well as monodisperse in physiological medium (Tomalia, Citation2005). The drug molecule can be encapsulated into the interior hydrophobic cavity or can bind to terminal groups through covalent conjugates or non-covalent interactions such as ionic and hydrogen-bond interactions (D'Emanuele & Attwood, Citation2005). The terminal groups are amenable for specific functionality and allow the attachment of molecules with drug moiety that can produce targeted, specific and controlled drug delivery. Of all the dendrimers, polyamidoamine (PAMAM) family is the most exploited and first commercialized family of dendrimers (Klajnert & Bryszewska, Citation2001). They have been reported to possess good transepithelial permeation characteristics across Caco-2 monolayers (El-Sayed et al., Citation2002; Kitchens et al., Citation2006) and everted rat intestinal sac models (Wiwattanapatapee et al., Citation2000) that renders them to be used as carriers for oral drug delivery. The studies demonstrated that they are transported by combination of paracellular pathway, transcellular route and an energy-dependent endocytosis process which is further influenced by generation and surface charge of PAMAM dendrimers (El-Sayed et al., Citation2003). Moreover, PAMAM dendrimers have been recognized as effective penetration enhancers recently that enhance the absorption of poorly water soluble drugs in rats (Lin et al., Citation2011). The authors investigated the influence of G0–G3 PAMAM dendrimers on the absorption of hydrophilic dye molecule 5(6)-carboxyfluorescein. PAMAM dendrimers showed concentration and generation-dependent enhancement in absorption of this dye molecule with the maximum of 11.1 fold that was examined in G2 PAMAM at 0.5 % w/v concentration. However, they observed membrane disruption at this concentration but it was reversible. Furthermore, the toxicity issues have been a major constraint in administration of the cationic PAMAM dendrimers orally. It was demonstrated earlier that PAMAM dendrimers produce concentration and generation-dependent cytoxocity (Chen et al., Citation2004) and hemolysis (Domanski et al., Citation2004). But in vitro (Kitchens et al., Citation2007) and in vivo (Roberts et al., Citation1996) studies have revealed that PAMAM dendrimers are non-toxic on Caco-2 cells at lower concentration of 0.01 mM and were non-toxic when administered to mice at a dose of 10 mg/kg up to fifth generation of PAMAM dendrimers, respectively. Considering these parameters of toxicity, transportation and biocompatibility, as discussed above, they can be used as effective nanocarriers for both hydrophobic and hydrophilic drugs. PAMAM dendrimers have already been used as oral drug delivery carriers for various drugs, namely naproxen (Najlah et al., Citation2007), SN 38 (Goldberg et al., Citation2011), doxorubicin (Ke et al., Citation2008) and silybin (Huang et al., Citation2011), etc.
The present work employs the use of PAMAM dendrimers as carriers for quercetin that may offer new opportunities to alleviate the difficulties encountered in its delivery. The work has been designed to improve the aqueous solubility of quercetin by using PAMAM dendrimers (generation 0, 1, 2, 3, i.e. G0, G1, G2 and G3, respectively). Quercetin has been incorporated and characterized by analyzing various parameters, namely particle size and size distribution, incorporation efficacy, investigation of nature of quercetin-PAMAM complexes, morphology of quercetin-loaded dendrimers and in vitro release studies. Moreover, the efficacy of quercetin-loaded dendrimer was assessed by a simple pharmacodynamic experiment taking inflammatory activity of quercetin into consideration. This experiment was performed to check the ability of PAMAM dendrimers to deliver the quercetin in response to inflammation.
Materials and methods
Materials
PAMAM dendrimers (G0–G3 with –NH2 end groups, ethylenediamine core) and quercetin were purchased from Sigma Aldrich (India). Acetonitrile (ACN) (HPLC: high-performance liquid chromatography grade) and o-phosphoric acid (HPLC grade) were purchased from MERCK and Spectrochem Pvt. Ltd. Mumbai, respectively. Biotech Cellulose Ester dialysis membrane with MWCO of 1000 Da was obtained from Spectrum laboratories, India. Ethanol, methanol and other chemicals were of analytical reagent grade.
Solubility studies
The water solubility of quercetin was determined by equilibrium solubility (the shake flask method) (Baka et al., Citation2008). The excess amount of quercetin was added to 1 mL of each test solution comprising 0.1, 0.5, 1, 2 and 4 µM concentrations of G0–G3 PAMAM dendrimers to ensure it reaching saturation. The solution was mechanically shaken for 24 h at 37 °C and centrifuged at 10 000 rpm for 10 min (Cheng et al., Citation2008a). Three repeats were conducted for each sample. The obtained saturated solutions were diluted accordingly with the dilution media, i.e. ACN: water (50:50) and samples were analyzed by HPLC.
Incorporation of quercetin in dendrimer
For incorporation of quercetin, initial molar ratios of quercetin/dendrimer were taken as 7, 23 and 40 for all generations of PAMAM dendrimer, keeping the amount of dendrimer constant, i.e. 1 µM. Briefly, quercetin was dissolved in 1 mL of methanol and then 1 mL of G0 PAMAM dendrimer (1 µM) was added to it. The sample was stirred overnight and methanol was dried under vacuum. The triple distilled water was added to obtain the traces and the mixture was stirred for another 24 h to extract the quercetin-PAMAM complexes and then filtered through 0.22 µm of cellulose acetate membrane filter. The non-encapsulated quercetin was removed at 4 °C against triple distilled water using 1 kDa dialysis membrane. Finally, the filterate was lyophilized to obtain quercetin-PAMAM complexes. Same procedure was repeated for other three generations (Huang et al., Citation2011).
HPLC analysis of quercetin
Quercetin was analyzed using an integrated HPLC system (LC-2010 CHT, Shimadzu, Japan) equipped with low pressure quaternary gradient pump along with dual wavelength UV (Ultraviolet) detector, autosampler and column oven. The column was RP C18 column (Luna; particle size 5 µm; 250 × 4.6 mm), maintained at 25 °C. The mobile phase comprises ACN: 0.1% phosphoric acid in water (76:24 v/v); was delivered at flow rate of 0.5 mL/min. The mobile phase was filtered through 0.45 µm membrane filter, degassed prior to use and detection was made at λmax 255 nm with UV detector (Curcio et al., Citation2012). shows the HPLC chromatogram of quercetin.
Characterization of quercetin-PAMAM complexes
Incorporation efficacy
Incorporation efficacy of PAMAM dendrimers was measured by saturation solubility of quercetin. It is calculated as the number of quercetin molecules incorporated in one molecule of dendrimer. The initial molar ratios of quercetin/dendrimer were known and ratio of quercetin/dendrimer complexes was obtained by estimating the amount of quercetin by HPLC analysis (Huang et al., Citation2011).
Fourier transform infrared spectroscopy
The structural changes in quercetin-PAMAM complexes were investigated using Fourier transform infrared (FTIR) spectrophotometer, Shimadzu Corporation (IR solution software). The samples were prepared by the potassium bromide disk technique and FTIR spectra of pure quercetin and all quercetin-PAMAM complexes were obtained (Pralhad & Rajendrakumar, Citation2004).
Particle size and size distribution analysis
The size and size distribution of the complexes were measured by using Malvern Zetasizer NanoZS using disposable sizing cuvette at 25 °C. One mL sample was taken for particle size analysis and for polydispersity index. All the measurements were done in triplicate.
Differential scanning calorimetery
Differential scanning calorimetery (DSC) is a technique that measures the temperature and heat flow associated with transition in metals in a controlled atmosphere. In order to investigate the physical state of quercetin in PAMAM dendrimers, thermograms of quercetin, PAMAM dendrimer and quercetin-PAMAM complexes were obtained using DSC. For this, the small amount (5 mg) of sample was sealed in the aluminum pan and the temperature was raised at 10 °C/min from 0 to 400 °C temperatures in the presence of nitrogen (20 mL/min) atmosphere. The DSC thermograms were recorded from 0 °C to 400 °C, as a plot of enthalpy (m/w) versus temperature (Pralhad & Rajendrakumar, Citation2004).
Morphology examination
The morphological studies were carried out by transmission electron microscopy (TEM) (Morgagni 268D, FEI Co., The Netherlands) using 3 mm Forman-coated copper grid. The samples negative stained by 2% phosphotungstic acid were analyzed with digital TEM image analysis system at 10 000 × magnification (Milenkovic et al., Citation2010).
In vitro release studies
The studies for in vitro release behavior of quercetin from its dendrimer complexes were performed by using the dialysis method. Quercetin-PAMAM complexes were dissolved in triple distilled water at a concentration equivalent to 2 mg/mL quercetin. Pure quercetin (control) was dissolved in 1 mL of methanol followed by dilution with triple distilled water to get a concentration of 2 mg/mL and used as a control. One milliliter of sample was transferred immediately to dialysis bag (MWCO 1 kDa). Then, dialysis bag was promptly placed in 500 mL glass beakers containing 400 mL of PBS of pH 7.4 maintained at 37 °C. The outer phase was stirred continuously with magnetic stirrer and samples (1 mL) were taken at specific time intervals 0.5, 1, 2, 4, 6, 8, 10, 12 and 24 h followed by replenishment with 1 mL of fresh dissolution medium. The amount of quercetin in the samples withdrawn from outer phase over a 24-h period was determined by HPLC. To know precisely about the mechanism by which the quercetin was released from PAMAM dendrimers, various release kinetic models such as zero order, first order, Higuchi and Korsmeyer–Peppas were applied (Huang et al., Citation2011).
Stability studies
The lyophilized quercetin-PAMAM complexes were subjected to stability studies as per ICH (International conference on harmonization) guidelines. We observed the effects of storage condition at 4 ± 2 °C and 25 ± 2 °C/60 ± 5% relative humidity (RH) for a period of 5, 10, 15, 20, 25 and 30 days in a stability chamber maintained at respective temperatures and RH, on the remaining quercetin concentration which was further estimated by HPLC (Pandita et al., Citation2009).
Pharmacodynamic study
Pharmacodynamic study was performed using adult female Wistar rats, weighing 220 ± 20 g, procured from Lala Lajpat Ray sadan, Vetenary University, Hissar and the protocol was approved by the Institutional Animal Ethics Committee, Jan Nayak Ch. Devi Lal Memorial College of Pharmacy, India (approval no.: JCDMCOP/IAEC/06/13/20). The animals were housed six per cage with 12 h light/dark cycles. They had free access to diet and water and were acclimatized for more than 7 days. Anti-inflammatory activity of orally administered quercetin suspension and quercetin-PAMAM complexes was determined in rats using a carrageenan-induced paw edema model. The dose of quercetin used for oral administration was 20 mg/kg body weight throughout the study (Date et al., Citation2011). For oral administration of pure quercetin, the stock solutions were prepared by dissolving 50 mg of pure quercetin in 10 mL of 0.2% of sodium carboxy methyl cellulose solution in water and used within 8 h. Quercetin-G3 PAMAM complexes used for oral administration were freshly prepared. All the rats were fasted for 12 h before the experiments but had free access to water. Animals were randomly divided into three groups: Group A was treated as control (no treatment); Group B received quercetin suspension in 0.2% sodium carboxy methyl cellulose solution; and Group C was given quercetin-G3 PAMAM complexes by oral gavage. After 30 min, rats of all three groups were challenged by a subcutaneous injection of 0.1 mL of a 1% (w/v) carrageenan solution, into the plantar site of the left-hind paw. The paw volumes were measured using Plethysmometer (PLM-01 PLUS ORCHID™ SCIENTIFICS), just before and after 1, 1.5, 2 and 3 h of carrageenan administration. The percent inhibition of edema at any time for each rat was calculated as
where A is the paw volume after the administration of carrageenan at time t, B is the mean paw volume of control rats after administration of carrageenan at time t, X is the paw volume before the administration of carrageenan and y is the mean paw volume of control rats before the administration of carrageenan.
Statistical analysis
Student's paired t-test was used to analyze the results (GraphPad InStat demo version). Differences were considered statistically significant at p < 0.05.
Results and discussion
Solubility studies
The influence of dendrimer concentration and generation on the solubility of quercetin was measured at 37 °C. As shown in , the apparent solubility of quercetin increased linearly and significantly as a function of PAMAM dendrimer over the whole concentration range. Several interaction mechanisms could be attributed to the enhancement of its solubility. First, the large number of amine groups present on surface of PAMAM dendrimers could electrostatically interact with hydroxyl groups of quercetin. Also, the internal tertiary amine groups could also interact with quercetin molecules via hydrogen bonding. Moreover, PAMAM dendrimers possess large internal cavities that are able to entrap the guest molecules in it and render the hydrophobic molecules to get solubilized in the aqueous medium.
The different generations of PAMAM dendrimer studied affected the solubility of quercetin significantly. The solubility of quercetin was found to enhance 145, 161, 181 and 206 times at 4 µM concentration of G0, G1, G2 and G3 PAMAM dendrimers, respectively, with respect to its water solubility that was found to be 5 µg/mL. It has already been reported for the NSAID's and various anti-cancer drugs like camptothecin that concentration as well as generation of PAMAM dendrimer has enhanced the solubility of these hydrophobic compounds effectively (Devarakonda et al., Citation2004; Yiyun & Tongwen, Citation2005; Cheng et al., Citation2008a). As the number of primary amines, tertiary amines and the internal cavity increases with generation of PAMAM dendrimer, the tendency to interact, i.e. adsorb/entrap more hydrophobic compounds increases, respectively. In this way, the obtained solubility results could be explained and justified.
Characterization of quercetin-PAMAM dendrimer complexes
The ability of different generations of PAMAM dendrimer to incorporate the quercetin molecules was estimated by HPLC and incorporation efficacy was determined by calculating the number of quercetin molecules incorporated per molecule of PAMAM dendrimer (). The number of moles/molecules of quercetin incorporated into PAMAM dendrimers was determined from its concentration obtained by HPLC. Since the amount of dendrimer was known, the results were expressed as number of quercetin molecules incorporated per dendrimer molecule. Although we used high quercetin/dendrimer ratio, i.e. 23 and 40 for G0, the number of quercetin molecules incorporated was found to be only 3. Similar was the case with G1 PAMAM, the number of quercetin molecules entrapped was found to be 7 in all the three quercetin/dendrimer molar ratios studied. The number increased to 19 and 34 for G2 and G3 PAMAM, respectively. Here, the numbers of molecules incorporated are greater than the number of primary amines present in G2, G3 PAMAM, respectively. It could possibly be due to strong electrostatic interactions of quercetin molecules with the core tertiary amine groups so that more molecules could get compacted in the dendrimer moiety. The results revealed that the number of molecules incorporated per molecule of dendrimer was equivalent to the number of free amines in the dendrimer structure. Similar results were observed in two separate studies where the number of silybin (Huang et al., Citation2011) and ibuprofen (Kolhe et al., Citation2003) molecules incorporated in PAMAM dendrimer was in accordance with the number of free amine terminal groups, respectively. Furthermore, it has already been stated that the encapsulation of the quercetin molecule in dendrimers increased with increase in its generation (Kojima et al., Citation2000). Similar results were reproduced in the present study in accordance with the previous studies.
Table 1. Number of quercetin molecules incorporated per molecule of PAMAM dendrimer.
FTIR spectra of all quercetin-dendrimer complexes (G0–G3) showed similar peaks. shows the FTIR spectra of pure quercetin, G0 PAMAM dendrimer and all the quercetin-PAMAM complexes. G0 PAMAM dendrimers showed N–H deformed vibrations at around 1631 cm−1 due to free terminal NH2 groups and C–N stretching vibration around 1029 cm−1. All other generations of pure PAMAM dendrimers followed similar pattern due to the presence of similar groups. Pure quercetin shows a broad band appearing at 3381 cm−1 due to O–H stretching vibrations of bonded polymeric hydroxyl groups. The ketonic C = O stretch conjugated with phenyl ring was observed at 1654 cm−1. Other C = C skeletal vibrations due to phenyl nucleus, C–O–C stretching of asymmetric dialkyl ether and O–H in-plane bending in quercetin were observed at 1371, 1093 and 1244 cm−1, respectively. In the spectral analysis of formulations, the ketonic C = O stretch (1700–1680 cm−1) due to quercetin, amidic C = O stretch (1680–1630 cm−1) due to PAMAM could be seen from all spectra but the N–H bending vibrations and C = C phenyl bending vibrations did not appear where the sharp pure quercetin C–O–C stretching peak of aryl ether at 1093 cm−1 was shifted to 1107, 1114, 1101 and 1112 cm−1 in G0, G1, G2 and G3 formulations, respectively, that confirm the formation of complex.
Figure 3. FTIR spectra of (a) quercetin, (b) G0 PAMAM dendrimer, and (c) quercetin PAMAM complexes: A – quercetin-GO PAMAM complexes, B – quercetin-G1 PAMAM complexes, C – quercetin-G2 PAMAM complexes, D – quercetin-G3 PAMAM complexes.
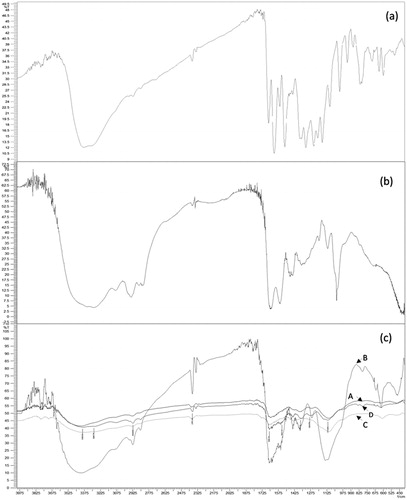
Quercetin-loaded dendrimers were subjected to particle size analysis and the results are shown in . All the quercetin-PAMAM dendrimer complexes were in the nano-size range, i.e. 34.39, 46.23, 59.72, and 100.3 nm and the polydispersity indices were found to be 0.238, 0.245, 0.293 and 0.321 for quercetin-G0, quercetin-G1, quercetin-G2 and quercetin-G3 PAMAM complexes, respectively. The lower polydispersity index suggested the narrow particle size distribution of the complexes. The DSC thermogram of quercetin showed sharp melting peak at 312 °C that provides evidence of its crystalline nature. But the quercetin-PAMAM complexes displayed no peak of quercetin suggesting its amorphous nature, when present in the quercetin-dendrimer complexes ().
Figure 4. Particle size and size distribution of (a) quercetin-G0 PAMAM complexes, (b) quercetin-G1 PAMAM complexes, (c) quercetin-G2 PAMAM complexes and (d) quercetin-G3 PAMAM complexes.
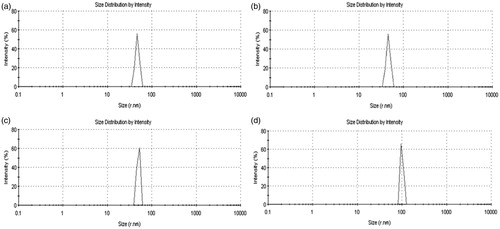
Figure 5. DSC thermograms of (a) quercetin-G0 PAMAM Complexes, (b) quercetin, (c) quercetin-G1 PAMAM complexes (d) quercetin-G2 PAMAM complexes and (e) quercetin-G3 PAMAM complexes.
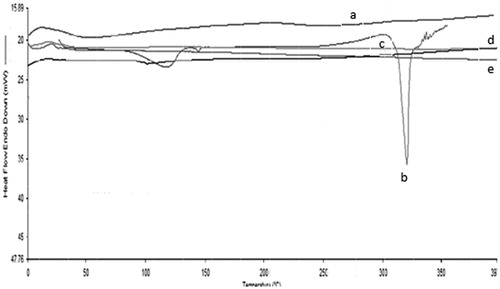
The complexes further characterized for surface morphology by TEM were found to be almost spherical. shows a representative TEM image of quercetin-G2 PAMAM complexes. The image revealed that the G2 PAMAM complexes were approximately 60 nm in size and uniform in shape with smooth surfaces without any aggregation.
In vitro release studies
The in vitro release kinetics of quercetin from PAMAM complexes in PBS (pH 7.4) was evaluated by the dialysis method. The release of quercetin was found to be biphasic which was characterized by an initial faster release followed by sustained release phase (). In the first hour, 31.2%, 26.3% and 21.1% of the quercetin was released from quercetin-G1, quercetin-G2 and quercetin-G3 PAMAM complexes, respectively. After 6 h, 96% of the quercetin was released from quercetin control whereas 46.8%, 43.1% and 38.2% of the quercetin was released from quercetin-G1, G2 and G3 PAMAM complexes, respectively. The slow quercetin release from G3 complexes could be due to the much stronger interaction of quercetin with interior tertiary amines of PAMAM dendrimers. To determine the release behavior of quercetin-PAMAM complexes, the release data were fitted into various kinetic models. It was found to follow Korsmeyer–Peppas kinetics from all the complexes. As the external electrostatic interactions were expected to be the major mechanism for drug complexation by PAMAM dendrimers, it could be suggested that the strength of electrostatic interactions determines the drug release behavior from dendrimers. In this case, it was assumed that the release of quercetin from PAMAM dendrimers was dependent on the strength of external electrostatic interactions between hydroxyl groups of quercetin and amine groups of PAMAM dendrimers.
Furthermore, the quercetin-PAMAM complexes showed no significant difference in drug concentration when subjected to stability studies at 4 ± 2 °C for one month. More than 99% of quercetin was retained in all the complexes when stored at 4 ± 2 °C while it was about 95% at 25 ± 2 °C/60 ± 5% RH after 30 days (). The obtained results indicated that quercetin-PAMAM complexes remained stable at 4 ± 2 °C.
Pharmacodynamic study
The anti-inflammatory activity of flavonoids including quercetin has been explained by Rotelli et al. in the various models of inflammation, including rat paw edema (Rotelli et al., Citation2003). The present study investigated the in vivo anti-inflammatory potential of quercetin-G3 PAMAM complexes as compared with pure quercetin. The results revealed that these complexes showed significant anti-inflammatory action (p < 0.05) in comparison to quercetin suspension (). The complexes were found to produce its maximum activity at 1 h whereas the suspension showed its highest activity at 1.5 h. The results indicated that PAMAM dendrimers helped in solubilizing the quercetin that resulted in quick onset of action when compared with that of the suspension. Although the quercetin-G3 PAMAM complex showed the highest anti-inflammatory activity (45%) at 1 h, the complex still showed significant activity (23%) at 3 h, meaning that the complex contributed to enhancing longer biological half-life of quercetin. Hence, this study provided the preliminary proof of concept about the potential of quercetin-PAMAM complexes.
Conclusion
PAMAM dendrimers have been reported as effective drug delivery systems. The present research work focused on the incorporation of quercetin, a poorly water soluble flavonoid in PAMAM dendrimers. PAMAM dendrimers of different generations G0–G3 were found to have an appreciable effect on water solubility of quercetin. Morphological studies by TEM revealed that the quercetin-loaded PAMAM complexes were spherical in shape and particle size analysis confirmed the nano-size range of complexes, i.e. from 34.39 to 100.3 nm. Furthermore, the FTIR spectral analysis confirmed the chemical nature of the formulation and quercetin was found to produce complexes with all generations of PAMAM dendrimers. Moreover, the prepared complexes showed sustained in vitro quercetin release following biphasic release pattern and were stable at 4 ± 2 °C for one month with respect to quercetin concentration. Furthermore, the complexes were found to improve the anti-inflammatory activity in rats as compared with quercetin suspension when administered orally. The problems associated with the delivery issue of quercetin could be overcome by incorporating it into PAMAM dendrimers and it is suggested that they can open up new avenues for a miracle molecule like quercetin, and further confirms PAMAM-based dendrimers as a suitable polymeric nanocarrrier for extensive applications, including oral drug delivery.
Acknowledgements
The authors would like to thank the Science and Engineering Research Board, Department of Science and Technology, Government of India for funding through the project SR/FT/LS-145/2011. The authors are also thankful to JCDM College of Pharmacy, Sirsa, India, for providing necessary facilities.
Declaration of interest
The authors report no conflicts of interest. The authors alone are responsible for the content and writing of this article.
References
- Alarcon de la Lastra C, Martin MJ, Motilva V. (1994). Antiulcer and gastroprotective effects of quercetin: a gross and histologic study. Pharmacology 48:56–62
- Aqil F, Munagala R, Jeyabalan J, Vadhanam MV. (2013). Bioavailability of phytochemicals and its enhancement by drug delivery systems. Cancer Lett [Epub ahead of print]. doi: 10.1016/j.canlet.2013.02.032
- Arora A, Nair MG, Strasburg GM. (1998). Structure-activity relationships for antioxidant activities of a series of flavonoids in a liposomal system. Free Radic Biol Med 24:1355–63
- Baka E, Comer JE, Takacs-Novak K. (2008). Study of equilibrium solubility measurement by saturation shake-flask method using hydrochlorothiazide as model compound. J Pharm Biomed Anal 46:335–41
- Beil W, Birkholz C, Sewing KF. (1995). Effects of flavonoids on parietal cell acid secretion, gastric mucosal prostaglandin production and Helicobacter pylori growth. Arzneimittelforschung 45:697–700
- Carluccio MA, Ancora MA, Massaro M, et al. (2007). Homocysteine induces VCAM-1 gene expression through NF-kappaB and NAD(P)H oxidase activation: protective role of Mediterranean diet polyphenolic antioxidants. Am J Physiol Heart Circ Physiol 293:H2344–54
- Chen HT, Neerman MF, Parrish AR, Simanek EE. (2004). Cytotoxicity, hemolysis, and acute in vivo toxicity of dendrimers based on melamine, candidate vehicles for drug delivery. J Am Chem Soc 126:10044–8
- Cheng Y, Li M, Xu T. (2008a). Potential of poly(amidoamine) dendrimers as drug carriers of camptothecin based on encapsulation studies. Eur J Med Chem 43:1791–5
- Cheng Y, Xu Z, MA M, XU T. (2008b). Dendrimers as drug carriers: applications in different routes of drug administration. J Pharm Sci 97:123–43
- Curcio M, Cirillo G, Parisi OI, et al. (2012). Quercetin-imprinted nanospheres as novel drug delivery devices. J Funct Biomater 3:269–82
- Dajas F. (2012). Life or death: neuroprotective and anticancer effects of quercetin. J Ethnopharmacol 143:383–96
- Date AA, Nagarsenker MS, Patere S, et al. (2011). Lecithin-based novel cationic nanocarriers (Leciplex) II: improving therapeutic efficacy of quercetin on oral administration. Mol Pharm 8:716–26
- D'Emanuele A, Attwood D. (2005). Dendrimer-drug interactions. Adv Drug Deliv Rev 57:2147–62
- Devarakonda B, Hill RA, De Villiers MM. (2004). The effect of PAMAM dendrimer generation size and surface functional group on the aqueous solubility of nifedipine. Int J Pharm 284:133–40
- Domanski DM, Klajnert B, Bryszewska M. (2004). Influence of PAMAM dendrimers on human red blood cells. Bioelectrochemistry 63:189–91
- El-Sayed M, Ginski M, Rhodes C, Ghandehari H. (2002). Transepithelial transport of poly(amidoamine) dendrimers across Caco-2 cell monolayers. J Control Release 81:355–65
- El-Sayed M, Rhodes CA, Ginski M, Ghandehari H. (2003). Transport mechanism(s) of poly (amidoamine) dendrimers across Caco-2 cell monolayers. Int J Pharm 265:151–7
- Goldberg DS, Vijayalakshmi N, Swaan PW, Ghandehari H. (2011). G3.5 PAMAM dendrimers enhance transepithelial transport of SN38 while minimizing gastrointestinal toxicity. J Controlled Release 150:318–25
- Huang X, Wu Z, Gao W, et al. (2011). Polyamidoamine dendrimers as potential drug carriers for enhanced aqueous solubility and oral bioavailability of silybin. Drug Dev Ind Pharm 37:419–27
- Hubbard GP, Wolffram S, Lovegrove JA, Gibbins JM. (2004). Ingestion of quercetin inhibits platelet aggregation and essential components of the collagen-stimulated platelet activation pathway in humans. J Thromb Haemost 2:2138–45
- Jackson CL, Chanzy HD, Booy FP, et al. (1998). Visualization of dendrimer molecules by transmission electron microscopy (TEM): staining methods and cryo-TEM of vitrified solutions. Macromolecules 31:6259–65
- Jullian C, Moyano L, Yanez C, Olea-Azar C. (2007). Complexation of quercetin with three kinds of cyclodextrins: an antioxidant study. Spectrochim Acta A Mol Biomol Spectrosc 67:230–4
- Kakran M, Sahoo NG, Li L. (2011). Dissolution enhancement of quercetin through nanofabrication, complexation, and solid dispersion. Colloids Surf B 88:121–30
- Ke W, Zhao Y, Huang R, et al. (2008). Enhanced oral bioavailability of doxorubicin in a dendrimer drug delivery system. J Pharm Sci 97:2208–16
- Khonkarn R, Mankhetkorn S, Hennink WE, Okonogi S. (2011). PEG-OCL micelles for quercetin solubilization and inhibition of cancer cell growth. Eur J Pharm Biopharm 79:268–75
- Kim HP, Mani I, Iversen L, Ziboh VA. (1998). Effects of naturally-occurring flavonoids and biflavonoids on epidermal cyclooxygenase and lipoxygenase from guinea-pigs. Prostaglandins Leukot Essent Fatty Acids 58:17–24
- Kitchens KM, Foraker AB, Kolhatkar RB, et al. (2007). Endocytosis and interaction of poly (amidoamine) dendrimers with Caco-2 cells. Pharm Res 24:2138–45
- Kitchens KM, Kolhatkar RB, Swaan PW, et al. (2006). Transport of poly(amidoamine) dendrimers across Caco-2 cell monolayers: influence of size, charge and fluorescent labeling. Pharm Res 23:2818–26
- Klajnert B, Bryszewska M. (2001). Dendrimers: properties and applications. Acta Biochim Pol 48:199–208
- Kobayashi H, Kawamoto S, Saga T, et al. (2001). Positive effects of polyethylene glycol conjugation to generation-4 polyamidoamine dendrimers as macromolecular MR contrast agents. Magn Reson Med 46:781–8
- Kojima C, Kono K, Maruyama K, Takagishi T. (2000). Synthesis of polyamidoamine dendrimers having poly(ethylene glycol) grafts and their ability to encapsulate anticancer drugs. Bioconjug Chem 11:910–17
- Kolhe P, Misra E, Kannan RM, et al. 2003. Drug complexation, in vitro release and cellular entry of dendrimers and hyperbranched polymers. Int J Pharm 259:143–60
- Kris-Etherton PM, Keen CL. (2002). Evidence that the antioxidant flavonoids in tea and cocoa are beneficial for cardiovascular health. Curr Opin Lipidol 13:41–9
- Kumari A, Kumar V, Yadav SK. (2012). Plant extract synthesized PLA nanoparticles for controlled and sustained release of quercetin: a green approach. PLoS One 7:1
- Lee KM, Hwang MK, Lee DE, et al. (2010). Protective effect of quercetin against arsenite-induced COX-2 expression by targeting PI3K in rat liver epithelial cells. J Agric Food Chem 58:5815–20
- Li H, Zhao X, Ma Y, et al. (2009). Enhancement of gastrointestinal absorption of quercetin by solid lipid nanoparticles. J Control Release 133:238–44
- Lin Y, Fujimori T, Kawaguchi N, et al. (2011). Polyamidoamine dendrimers as novel potential absorption enhancers for improving the small intestinal absorption of poorly absorbable drugs in rats. J Control Release 149:21–8
- Masella R, Giovannini C, Vari R, et al. (2001). Effects of dietary virgin olive oil phenols on low density lipoprotein oxidation in hyperlipidemic patients. Lipids 36:1195–202
- Milenkovic M, Arsenovic-Ranin N, Stojic-Vukanic Z, et al. (2010). Quercetin ameliorates experimental autoimmune myocarditis in rats. J Pharm Pharm Sci 13:311–19
- Mizui T, Sato H, Hirose F, Doteuchi M. (1987). Effect of antiperoxidative drugs on gastric damage induced by ethanol in rats. Life Sci 41:755–63
- Najlah M, Freeman S, Attwood D, D'Emanuele, A. (2007). In vitro evaluation of dendrimer prodrugs for oral drug delivery. Int J Pharm 336:183–90
- Pandita D, Ahuja A, Velpandian T, et al. (2009). Characterization and in vitro assessment of paclitaxel loaded lipid nanoparticles formulated using modified solvent injection technique. Pharmazie 64:301–10
- Patton DL, Cosgrove Sweeney YT, Mccarthy TD, Hillier SL. (2006). Preclinical safety and efficacy assessments of dendrimer-based (SPL7013) microbicide gel formulations in a nonhuman primate model. Antimicrob Agents Chemother 50:1696–700
- Pralhad T, Rajendrakumar K. (2004). Study of freeze-dried quercetin-cyclodextrin binary systems by DSC, FT-IR, X-ray diffraction and SEM analysis. J Pharm Biomed Anal 34:333–9
- Priprem A, Watanatorn J, Sutthiparinyanont S, et al. (2008). Anxiety and cognitive effects of quercetin liposomes in rats. Nanomedicine 4:70–8
- Roberts JC, Bhalgat MK, Zera RT. (1996). Preliminary biological evaluation of polyamidoamine (PAMAM) Starburst dendrimers. J Biomed Mater Res 30:53–65
- Rotelli AE, Guardia T, Juarez AO, et al. (2003). Comparative study of flavonoids in experimental models of inflammation. Pharmacol Res 48:601–6
- Shoskes DA. (1998). Effect of bioflavonoids quercetin and curcumin on ischemic renal injury: a new class of renoprotective agents. Transplantation 66:147–52
- Silva MM, Santos MR, Caroco G, et al. (2002). Structure-antioxidant activity relationships of flavonoids: a re-examination. Free Radic Res 36:1219–27
- Tomalia DA. (2005). Birth of a new macromolecular architecture: dendrimers as quantized building blocks for nanoscale synthetic polymer chemistry. Progress Polym Sci 30:294–324
- Tomalia DA, Baker H, Dewald J, et al. (1985). A new class of polymers: starburst-dendritic macromolecules. Polym J 17:117–32
- Wiwattanapatapee R, Carreno-Gomez B, Malik N, Duncan R. (2000). Anionic PAMAM dendrimers rapidly cross adult rat intestine in vitro: a potential oral delivery system? Pharm Res 17:991–8
- Wu TH, Yen FL, Lin LT, et al. (2008). Preparation, physicochemical characterization, and antioxidant effects of quercetin nanoparticles. Int J Pharm 346:160–8
- Yiyun C, Tongwen X. (2005). Dendrimers as potential drug carriers. Part I. Solubilization of non-steroidal anti-inflammatory drugs in the presence of polyamidoamine dendrimers. Eur J Med Chem 40:1188–92