Abstract
Objective: The objective of present study was to develop and evaluate paliperidone (PALI) loaded microemulsion (PALI-ME) for intranasal delivery in the treatment of schizophrenia.
Material and methods: The PALI-ME was formulated by the spontaneous microemulsification method and characterized for physicochemical parameters. Pharmacodynamic assessments (apomorphine-induced compulsive behavior and spontaneous motor activity) were performed using mice. All formulations were tagged with 99mTc (technetium). Pharmacokinetic evaluation of PALI in the brain was investigated using Swiss albino rats. Brain scintigraphy imaging was performed in rabbits.
Results and discussion: PALI-ME was found stable with average droplet size of 20.01 ± 1.28 nm. In pharmacodynamic studies, significant (p < 0.05) deference in parameters estimated, were found between the treated and control groups. 99mTc-tagged PALI solution (PALI-SOL)/PALI-ME/PALI muco-adhesive ME (PALI-MME) was found to be stable and suitable for in vivo studies. Brain-to-blood ratio at all sampling points up to 8 h following intranasal administration of PALI-MME compared to intravenous PALI-ME was found to be 6–8 times higher signifying greater extent of distribution of the PALI in brain. Rabbit brain scintigraphy demonstrated higher intranasal uptake of the PALI into the brain.
Conclusion: This investigation demonstrates a prompt and larger extent of transport of PALI into the brain through intranasal PALI-MME, which may prove beneficial for treatment of schizophrenia.
Introduction
Schizophrenia is a major psychotic disorder carrying a high risk of suicide and other life-threatening behaviors. Key issue in the schizophrenia management is adherence with the treatment. The reasons for non-adherence to medication include the lack of efficacy, intolerability and patient decision (Canuso et al., Citation2008). The need for treatment options tailored to improved delivery of the existing treatment class has been emphasized by the recent Clinical Antipsychotic Trials of Intervention Effectiveness study, until new drugs with novel mechanisms of action were available (Nasrallah et al., Citation2005; Zacher & Grady, Citation2007; Canuso et al., Citation2008; Patel et al., Citation2013a,Citationb).
First-generation antipsychotics are effective against psychosis but they do not improve and may even worsen the negative symptoms of schizophrenia and are linked with dose-limiting extrapyramidal symptoms (Patel et al., Citation2013b). Paliperidone (PALI) represents the second-generation most recent atypical antipsychotics which is chemically benzisoxazole derivative and commonly referred to as 9-hydroxyrisperidone. PALI is indicated for the short- and long-term treatment of schizophrenia. It has dual-action of blocking dopamine and serotonine D2/5-HT2A receptor. Reportedly, PALI is practically insoluble in water (0.003 mg/100 mL) (Patel et al., Citation2010). Presently, PALI is available in a form of an extended release tablet which has oral bioavailability of 28% (Zacher & Grady, Citation2007). Disadvantages to this dosage form include complex manufacturing, use of harsh solvents in their preparation and slow transport along gastrointestinal tract, and poor bioavailability (Nasrallah et al., Citation2005; Patel et al., Citation2013a).
Oral route of drug administration is most convenient and well accepted. However, oral administration is inappropriate when drug undergoes significant degradation in the gastrointestinal tract or is metabolized to a high degree via the first pass effect in liver. Moreover, expression of efflux transporter in certain tissue compartments such as the gastrointestinal tract and brain capillary endothelial cells limits oral absorption and central nervous system (CNS) entry of many drugs (Wolfgang & Heidrun, Citation2005). Further, patients who are unable to swallow cannot be medicated by oral route which limits its utility in this particular population of patients. Hence, an alternative route of administration should be preferable. Reportedly, nasal mucosal membrane offers a practical non-invasive route of administration for the therapeutic effect of many drugs (Li et al., Citation2002; Vyas et al., Citation2006; Jogani et al., Citation2008; Kaur & Kim, Citation2008; Elshafeey et al., Citation2009; Patel et al., Citation2013b). Nasal route has advantages of fast absorption of drug, enhanced bioavailability even at lower doses, rapid onset of therapeutic action, circumvent liver or gastrointestinal metabolism, reduced risk of overdose, non-invasive administration, convenient, self-medication and better patient compliance (Li et al., Citation2002; Vyas et al., Citation2006; Jogani et al., Citation2008; Kaur & Kim, Citation2008; Elshafeey et al., Citation2009; Patel et al., Citation2013b). Scientists are making many attempts to find efficient and new ways to prevent extensive first pass metabolism and target the drug to the receptor site in brain for treating brain disorders (Wolfgang & Heidrun, Citation2005; Porecha et al., Citation2009; Fazil et al., Citation2012; Md et al., Citation2013; Mahajan et al., Citation2014).
However, the limitation for intranasal drug delivery is the fact that most drugs diffuse poorly and slowly through the nasal mucosa, and thus the therapeutically effective levels of drug cannot be achieved. Further, the nasal cavity accommodate limited volume of formulation, approximately 150 µL/nostril and excess formulation will drain out into the pharynx and be swallowed (Shinde et al., Citation2011; Patel et al., Citation2013a,Citationb).
The present study solves the above-mentioned problem and explores the suitable drug delivery vehicle, microemulsion (ME) which is able to dissolve the PALI, in required concentration, yet which is not irritating to the nasal mucosa. The intranasal administration of drug can be increased by co-administering a permeation enhancer (Patel et al., Citation2013b). ME have been thoroughly studied as a drug carrier due to their special features, such as ease of preparation due to spontaneous formation, thermodynamic stability, high-solublization capacity for the hydrophilic and lipophilic drugs, transparent and elegant appearance, enhanced penetration through the biological membranes, increased bioavailability, and less inter- and intra-individual variability in drug pharmacokinetics (Li et al., Citation2002; Zhang et al., Citation2004; Wolfgang & Heidrun, Citation2005; Kaur & Kim, Citation2008; Elshafeey et al., Citation2009; Porecha et al., Citation2009; Shinde et al., Citation2011; Fazil et al., Citation2012; Md et al., Citation2013; Mahajan et al., Citation2014).
An additional constraint concerning nose-to-brain drug delivery is the natural defense mechanism – nasal mucociliary clearance. Therefore it is essential to localize the formulation on a mucosal layer of nasal cavity to enhance drug absorption and prevent rapid nasal clearance. Addition of a well-designed muco-adhesive polymer to ME can prevent rapid nasal clearance of formulation (Patel et al., Citation2013b). This paper describes the development, characterization and pharmacodynamic–pharmacokinetic evaluation of PALI-loaded MME designed using Generally Regarded As Safe (GRAS) listed ingredients and polycarpophil AA-1 as a muco-adhesive component.
Materials and methods
Drug and reagents
PALI pure powder was obtained as gratis sample from Torrent Pharmaceutical Ltd. (Ahmedabad, India) with 99.9% purity. Caprylocaproyl polyoxylglycerides and diethylene glycol monoethyl ether (Gattefosse, Saint-Priest, France) were procured as gratis samples from Gattefosse Asia Ltd. (Mumbai, India). Polyoxyl 40 hydrogenated castor oil was procured as gratis sample form BASF (Mumbai, India). Polycarbophil (AA-1, pharmagrade, molecular weight approximately 3.5 million) was procured as gratis sample from Lubrizol Advanced Material India Pvt. Ltd. (Mumbai, India). Oleic acid, potassium dihydrogen phosphate, methanol and propylene glycol were purchased from SDfine Chemicals (Ahmedabad, India). Ethanol was purchased from Baroda Chemical Ind. Ltd. (Dabhoi, India). Double-distilled water was used throughout the study. All other chemicals and solvents were of analytical reagent grade and used as-received without further purification.
Preparation and characterization of formulations
The ME for PALI was formulated by the spontaneous microemulsification method (Patel et al., Citation2013a). The predetermined quantity of PALI (5 mg/mL) was added to the oily phase (oleic acid) of ME and magnetically stirred until dissolved followed by the addition of emulsifier mixture (Emix) [caprylocaproyl polyoxylglycerides and polyoxyl 40 hydrogenated castor oil (non-ionic emulsifiers) (1:1) to diethylene glycol monoethyl ether (coemulsifier)] in a fixed proportion (3:1) to produce clear mixture. Then a defined proportion of water was added to produce crystal clear ME of PALI (PALI-ME). The muco-adhesive ME of PALI (PALI-MME) was formulated by initially preparing ME of the PALI using minimum volume of external phase and then adding the required volume of polymer solution (1%, w/v) so that the final concentration of polymer in the MME was 0.5% (w/w). After the addition of polymer solution the MME was allowed to homogenize for 10 min. The PALI solution (PALI-SOL) meant for comparative evaluation of MME-based systems was formulated by dissolving PALI (50 mg) in 10 mL of propylene glycol resulting in a solution of 5 mg/mL.
PALI content in the formulations was assayed using a high-performance thin layer chromatography (TLC, Patel et al., Citation2010). The average droplet size and polydispersity index (PDI) of ME was determined using photon correlation spectroscopy (PCS) with in-built Zetasizer (Model: Nano ZS, Malvern Instruments, Worcestershire, UK WR141XZ) at 633 nm. Helium–neon gas laser having an intensity of 4 mW was the light source. The droplet size was calculated using the Stokes–Einstein relationship by Zetasizer Software. Electrophoretic mobility (µm/s) was measured using small volume disposable zeta cell and converted to zeta potential by in-built software using the Helmholtz–Smoluchowski equation (Vyas et al., Citation2006; Patel et al., Citation2013a). The pH value of ME was determined using digital pH meter (HI 98107, Hanna Instruments, TX, USA), standardized using pH 4 and 7 buffers before use (Patel et al., Citation2013a). The viscosity of ME was measured using a Brookfield Viscometer LVDV – IIIU (Brookfield Engineering LABS, Stoughton, MA, USA) with spindle SC 18 at 100 rpm using interval of 30 s. All aspects of testing were controlled using Rheocalc Software (Patel et al., Citation2013a).
Stability studies
The formulations, PALI-ME and PALI-MME, were subjected to stability studies for a period of 6 months at room temperature and refrigerated conditions (4 °C). After 6 months of storage, the formulations were characterized for physical stability (creaming, phase separation or flocculation), accelerated centrifugation cycle (3000g for 15 min), PALI content, particle size and zeta potential determinations (Patel et al., Citation2013a).
Pharmacodynamic study
The protocol for animal experimentations was approved by the Committee for the Purpose of Control and Supervision of Experiments on Animals (CPCSEA) and the Institutional Animal Ethics Committee (IAEC) of A.R. College of Pharmacy & G.H. Patel Institute of Pharmacy, Vallabh Vidyanagar, Gujarat (Protocol No.: CPCSEA/IAEC/ARCP/09-10/01).
Apomorphine-induced compulsive behavior
The study was performed in six groups of six mice in each of either sex with an average weight of 25 g. One group received distilled water as control while other groups received PALI-ME (IV), PALI-SOL (IN), PALI-ME (IN), and PALI-MME (IN), and the remaining group received haloperidole (1 mg/kg BW) (Kulkarni & Jisheph, Citation1998) as positive control. The intranasal formulations were administered in mice using micropipette (5 µL/nostril) at the dose of 0.05 mg PALI (equivalent to 2 mg/kg of BW). After 1 h of treatment, apomorphine hydrochloride (2 mg/kg of BW) was administered intraperitoneally (IP) to all animals. The mice were individually placed in glass containers of 250 mL capacity and the sign of stereotyped behavior, which include sniffing, rearing, licking and gnawing, were observed at 0, 15, 30, 45, 60 min after apomorphine administration. The intensity of stereotypy was recorded by the following scoring system (Shibuya & Nishimori, Citation1982; Kulkarni & Jisheph, Citation1998, Patel et al., Citation2013b).
Discontinuous sniffing, constant exploratory activity.
Continuous sniffing, periodic exploratory activity, small head movements.
Continuous sniffing, small body and head movements, discontinuous gnawing, biting and licking the container wall, brief spurts of spontaneous motor activity.
Continuous gnawing, biting and licking the cage wall, no ambulation except for occasional backward movement.
For each mouse, a global score was calculated by averaging the five stereotype scores obtained at the mentioned time interval.
Spontaneous motor activity
Mice of either sex with average weight of 25 g were selected and deprived of food and water for 24 h before the experiment. To avoid any effect of the circadian rhythm, the study was performed during 8.00 and 12.00 am. Six mice were selected per time point. The intranasal formulations (PALI-SOL, PALI-ME and PALI-MME) were administered in mice with the help of micropipette (5 µL/nostril) at the dose of 0.05 mg PALI (equivalent to 2 mg/kg of BW) followed by intraperitoneal injection of L-dopa (13 mg/kg of BW) and carbidopa (3.25 mg/kg of BW) after 30 min. The spontaneous motor activity was measured for 10 min by placing the animals in digital photoactometer (INCO, Ambala, India). For the formulation PALI-ME, given by intravenous route, the spontaneous motor activity was measured after 2 min for a period of 10 min (Patel et al., Citation2013b).
Pharmacokinetic study
The protocol for animal experimentation was approved by the Committee for the Purpose of Control and Supervision of Experiments on Animals (CPCSEA) and the Institutional Animal Ethics Committee (IAEC) of Veterinary Nuclear Medicine Centre, Bombay Veterinary College, Mumbai (Protocol No.: IAEC/BVC/42/09).
Preparation of technetium-tagged formulations
PALI in PALI-SOL, PALI-ME and PALI-MME, was tagged with radioactive substance, technetium-99 (99mTc) by the direct labeling method (Eckelman, Citation1995; Babbar et al., Citation2000; Li et al., Citation2002; Zhang et al. Citation2004; Vyas et al. Citation2006; Kaur & Kim, Citation2008; Patel et al. Citation2013b). To 1.0 mL of PALI formulation, 200 µL of stannous chloride dehydrate (2 mg/mL in 10% acetic acid) was added, and the pH was adjusted to 6.0–6.5 using 50 mM sodium bicarbonate solution. The resultant mixture was filtered through 0.22µ nylon 66 membrane and the required volume of sterile 99mTc-pertechnetate (5 mCi) was added over a period of 60 s with continuous mixing, such that the resultant solution had a radioactivity of 5 mCi/mL and was incubated at 30 ± 5 °C for 30 min with continuous nitrogen purging. The final volume was made up to 2.5 mL using 0.9% (w/v) sterile sodium chloride solution. The resultant formulations obtained had 100 µCi/20 µL activity.
The radiochemical purity (Eckelman, Citation1995; Babbar et al., Citation2000; Patel et al., Citation2013b) of 99mTc-tagged PALI-SOL (99mTc-PALI-SOL), 99mTc-tagged PALI-ME (99mTc-PALI-ME) and 99mTc-tagged PALI-MME (99mTc-PALI-MME) was determined by ascending instant TLC using silica gel-coated fiberglass sheets and acetone as the mobile phase. The effects of incubation time, pH and stannous chloride concentration on radiolabeling efficiency were studied to achieve optimum conditions. All 99mTc-tagged formulations were challenged to assess bonding strength at different molar concentrations (25–100 mM) of diethylene triamine penta acetic acid (Eckelman, Citation1995; Babbar et al., Citation2000; Patel et al., Citation2013b). The optimized 99mTc-tagged formulations were evaluated for in vitro stability in 0.90% (w/v) sodium chloride (normal saline) and in plasma (Eckelman, Citation1995; Babbar et al., Citation2000; Patel et al., Citation2013b). The optimized, stable 99mTc-tagged formulations of PALI were used for biodistribution study in rats.
Bio-distribution studies
Wistar rats (male, aged 4–5 months), weighing between 200 and 250 g, were selected for the study. Four rats for each formulation per time point were used in the study. The 99mTc-tagged complex of 99mTc-PALI-ME (100 µCi/20 µL) containing 0.035–0.040 mg PALI (equivalent to 0.18–0.20 mg/kg body weight (BW)) was injected through tail vein of Wistar rats. Similarly, 99mTc-tagged complex of 99mTc-PALI-SOL/PALI-ME/PALI-MME (100 µCi/20 µL) containing 0.035–0.040 mg PALI (equivalent to 0.18–0.20 mg/kg BW) was administered (10 µL) in each nostril. Prior to nasal administration of the formulations, the rats were anaesthetized using 50 mg/kg ketamine intramuscular injection and the formulations were instilled into the nostrils with the help of micropipette (10–100 µL) attached with low-density polyethylene tube having 0.1 mm internal diameter at the delivery site. The rats were held from the back in slanted position during nasal administration of the formulations. The rats were sacrificed at predetermined time intervals and blood was collected using cardiac puncture. Subsequently, different tissues/organs including brain and spinal cord were dissected, washed twice using normal saline solution, and made free from adhering tissue/fluid and weighed. The radioactivity present in each tissue/organ was measured using shielded well-type gamma scintillation counter. The radiopharmaceutical uptake per gram in each tissue/organ was calculated as a fraction of administered dose by the following equation (Li et al., Citation2002; Zhang et al., Citation2004; Vyas et al., Citation2006; Jogani et al., Citation2008; Kaur & Kim, Citation2008):
To evaluate the brain-targeting efficiency, two indexes drug (PALI) targeting efficiency (DTE, %) and nose-to-brain direct transport percentage (DTP, %) of PALI were adopted as mentioned below. DTE (%) represents time average partitioning ratio, which has been derived from Equation (Equation1(1) ). In order to define nose–brain direct transport clearly, “the brain drug direct transport percentage (DTP, %)” was calculated; which has been derived from Equation (Equation2
(2) ).
(1)
(2)
where AUC is the area under the curve; Bx is the brain AUC fraction contributed by systemic circulation through the blood–brain barrier (BBB) following intranasal administration; Bi.v. is the AUC0 → 480 (brain) following intravenous administration; Pi.v. –is the AUC0 → 480 (blood) following intravenous administration; Bi.n. is the AUC0 → 480 (brain) following intranasal administration; Pi.n. is the AUC0 → 480 (blood) following intranasal administration.
Reports in the literature reveal that the drug uptake into the brain from the nasal mucosa mainly occurs via three different pathways (Vyas et al., Citation2006; Jogani et al., Citation2008; Porecha et al., Citation2009). One is the systemic pathway by which some of the drug is absorbed into the systemic circulation and subsequently reaches the brain by crossing BBB. The others are the olfactory pathway and the trigeminal neural pathway by which partly the drug travels directly from the nasal cavity to CSF and brain tissue. We can conclude that the amount of drug reaching the brain tissue after nasal administration is attributed to these three pathways. Thus, we can assume that the brain AUC fraction contributed by systemic circulation through BBB (represented by Bx), divided by plasma AUC from nasal route is equal to that of IV route (Equation (Equation1(1) )). Therefore, DTP (%) represents the percentage of drug directly transported to the brain via the olfactory pathway and the trigeminal neural pathway. DTP (%) and DTE (%) were calculated using tissue/organ distribution data following intranasal and intravenous administrations (Vyas et al., Citation2006; Jogani et al., Citation2008; Fazil et al., Citation2012; Md et al., Citation2013; Patel et al., Citation2013b).
Gamma scintigraphy imaging
The New Zealand rabbits (2.00–2.50 kg) were selected for the study. The 99mTc-tagged complex of 99mTc-PALI-SOL (100 mCi/100 µL) containing 0.20–0.25 mg PALI (equivalent to 0.1–0.13 mg/kg BW) was IV injected through the ear vein of the rabbit. Similarly, the 99mTc-tagged complex of 99mTc-PALI-SOL/99mTc-PALI-ME/99mTc-PALI-MME (100 mCi/100 µL) containing 0.20–0.25 mg PALI (equivalent to 0.1–0.13 mg/kg BW) was administered IN (50 µL in each nostril). The rabbits were held from the back in slanted position during nasal administration of formulations. The rabbits were anesthetized using 1 mL ketamine hydrochloride intramuscular injection (50 mg/mL) and placed on the imaging platform. Imaging was performed using Single Photon Emission Computerized Tomography (SPECT, LC 75-005, Diacam, Siemens AG; Erlanger, Germany) gamma camera (Vyas et al., Citation2006; Jogani et al., Citation2008; Fazil et al., Citation2012; Md et al., Citation2013; Patel et al., Citation2013b).
Statistical analysis
All data are reported as mean ± SEM and the difference between the groups were tested using Student’s t-test at the level of p < 0.05. More than two groups were compared using ANOVA, with p < 0.05 considered statistically significant.
Results and discussion
Formulation development and characterization
ME formulations containing oleic acid as an oil phase, were formulated at caprylocaproyl polyoxylglycerides and polyoxyl 40 hydrogenated castor oil–diethylene glycol monoethyl ether (1:1) fixed Emix ratios of 3:1 (). The ME containing 4% w/w oleic acid, 30% w/w Emix and 66% w/w distilled water showed highest solubilizing capacity for PALI. Polycarbophil (0.5% w/w) was used as a muco-adhesive polymer and incorporated in ME formulation to obtained PALI-loaded MME. All formulations were formulated and characterized for the various physicochemical parameters (). The PALI content (%) of PALI-SOL, PALI-ME and PALI-MME was found to be 99.56 ± 1.85, 99.89 ± 2.66 and 99.12 ± 2.28, respectively, of the theoretical value (5 mg/mL). The narrow droplet size range of 20.01 ± 1.28 and 27.31 ± 1.86 nm and PDI of 0.117 ± 0.034 and 0.241 ± 0.049 for PALI-ME and PALI-MME, respectively, indicated that the ME approached a mono-dispersed stable drug delivery system and could deliver the PALI effectively due to larger surface area. The presence of zeta potential to the tune of −36.59 ± 2.03 and −38.65 ± 2.39 mV on the droplets of PALI-ME, and PALI-MME, respectively, conferred physical stability to the formulations. PALI-ME showed net negative charge and incorporation of muco-adhesive polymer in PALI-ME further increases negative charge of the ME formulation. This may be attributed to the fact that the increase in emulsifier level decreases surface tension and surface free energy of the formed micelles. Therefore, net negative charge (anionic) of the ME increased (Li et al., Citation2002; Zhang et al., Citation2004; Vyas et al., Citation2006; Jogani et al., Citation2008; Kaur & Kim, Citation2008; Elshafeey et al., Citation2009; Shinde et al., Citation2011; Patel et al., Citation2013a,Citationb). The MEs were expected to have good physical stability (phase separation) as zeta potential is between −30 and −40 mV (Vyas et al., Citation2006; Jogani et al., Citation2008; Patel et al., Citation2013b). Moreover, addition of muco-adhesive polymer (polycarbophil) further increases negative charge of ME formulation and therefore improves its physical stability (Vyas et al., Citation2006; Jogani et al., Citation2008; Patel et al., Citation2013b;). A percentage transmittance of 99.97% for PALI-ME indicated clear dispersion, whereas PALI-MME was not clear due to the presence of muco-adhesive polymer. The ME formulations were examined in a cross polarizer for sample homogeneity and birefringence. The PALI-ME appeared completely dark when observed under cross polarizer which confirmed its optically isotropic nature. The pH of all the PALI-SOL, PALI-ME and PALI-MME was found in the range of 5.85–5.98, approximating the normal pH range of nasal fluids (Vyas et al., Citation2006; Jogani et al., Citation2008; Patel et al., Citation2013b), which is one of the formulation considerations that may help reducing the irritation during intranasal administration of PALI formulation. It was observed that the viscosity of the ME formulations generally was very low. This was expected, because one of the characteristics of ME formulations is of lower viscosity (Vyas et al., Citation2006; Jogani et al., Citation2008; Patel et al., Citation2013b). Low viscosity values of PALI-ME (77 ± 3.64 cp) and PALI-MME (96 ± 5.11 cp), ensure easy handling, packing and hassle-free nasal administration of formulations.
Table 1. Composition of PALI-ME and PALI-MME.
Table 2. Characterization parameters of optimized PALI-ME and PALI-MME (n = 3).
Results of stability studies reveled that all formulations were found to be stable. The results showed that there are negligible changes in the physicochemical parameters such as PALI content, % transmittance, droplet size and zeta potential of PALI-ME and PALI-MME after 6 months of storage at room temperature and refrigerated condition, thus substantiating the stability of formulations for 6 months (data not shown).
Pharmacodynamic evaluation
Compulsive behavior is defined as purposeless activity exhibited by the animal. This purposeless activity is supposed to be identical to the behavioral disorder seen in schizophrenia (psychotic) patients who also show repetitive purposeless activity. This behavioral abnormality in schizophrenia is due to the excessive neuronal activity of dopamine in the limbic system. Apomorphine, a dopamine receptor agonist, through its dopaminergic activity induces compulsive stereotyped behavior in mice. The stereotyped behavior includes rearing (repetitive standing), continuous sniffing (touching the nose to the wall of the container) and licking the wall of container (Patel et al., Citation2013b).
As shown in , all animals administered PALI formulations by either routes exhibited significant difference (p < 0.05) in inhibition of apomorphine-induced stereotype behavior. Absence of apomorphine induced stereotype behavior was observed in mice pretreated with haloperidole. Increased passage of PALI via paracellular or transcellular mechanism to the brain via the olfactory region of the nasal cavity was demonstrated by lower value of score for stereotype behavior for PALI-ME when compared with PALI-SOL. Furthermore, PALI-MME showed lower score for stereotype behavior () when compared with PALI-ME, both administered intranasally, indicating the superiority of intranasal PALI-MME over PALI-ME and clearly demonstrates the role of polycarbophil (muco-adhesive polymer) that prevent nasociliary clearance thereby prolonging the residence time, and is also suggested to play a role in the enhancement of penetration of the formulation across the cells (Braso et al., Citation2003). PALI-MME formulation administered by intranasal routes showed a lower value of score for stereotype behavior than PALI-ME administered intravenously, suggesting better brain uptake of the PALI by intranasal route as MME when compared with other PALI formulations.
Table 3. Pharmacodynamic study (apomorphine-induced compulsive behavior) of PALI formulation in normal mice (n = 6).
In CNS, the neurotransmitter dopamine mediates a wide array of physiological functions including regulation of spontaneous motor activity. It is generally believed that spontaneous motor activity results from brain activation, which is manifested as a result of excitation of central neurons and an increase in cerebral metabolism. While different neurochemical mechanisms are involved in brain activation, dopamine appears to play an essential role; pharmacological blockade of dopamine transmission inhibits spontaneous motor activity (Le & Simon, Citation1991; Chow et al., Citation1999; Salamone et al., Citation2005; Patel et al., Citation2013b).
The animals of the control group (not administered PALI formulation) showed maximum spontaneous motor activity due to the D2 receptor stimulation by L-dopa and carbidopa that were used to develop the behavior model of schizophrenia in mice by improving dopamine concentration in the mesolimbic system where the drug(s) acts on 5HT2 and D2 receptor (Le & Simon, Citation1991; Chow et al., Citation1999; Salamone et al., Citation2005; Patel et al., Citation2013b); whereas the groups which were administered formulations containing PALI (PALI-SOL, PALI-ME and PALI-MME) exhibited significant reduction (p < 0.05) in the spontaneous motor activity (). The reduction in spontaneous motor activity by intranasal administration of PALI-SOL, PALI-ME and PALI-MME was better when compared with PALI-ME administered intravenously, signifying superiority of nose-to-brain delivery of PALI over intravenous route of administration. The results demonstrate superior delivery of PALI to the brain via intranasal route from the formulation containing muco-adhesive polymer polycarbophil (0.50% by weight). The muco-adhesive polymer used in the formulation not only prevents the nasociliary clearance but also suggested to play a role in the enhancement of penetration of the drug across the cells. These pharmacodynamic reports are consistent with the earlier findings reported in literature (Patel et al., Citation2013b).
Table 4. Pharmacodynamic study (spontaneous motor activity) of PALI formulation in normal mice (n = 6).
Pharmacokinetic evaluation
PALI-SOL, PALI-ME and PALI-MME formulations were effectively tagged with 99mTc and optimized for maximum tagging efficiency and stability. Radiochemical purity achieved was 98.10%, 98.90% and 99.20% for PALI-SOL, PALI-ME and PALI-MME, respectively, when evaluated for reduced/hydrolyzed (R/H) 99mTc and free 99mTc. The optimal SnCl2·2H2O concentration was found to be 200 µL (2 mg/mL) at pH 6.0–7.0 with an incubation time of 30 min. 99mTc-PALI-SOL/PALI-ME/PALI-MME were found to be stable in normal saline solution and plasma up to 24 h (degradation <5% w/w). Bonding strength of 99mTc-PALI-SOL/PALI-ME/PALI-MME was evaluated by the DTPA challenging test, and the percent trans-chelation of the tagged complex was 1.62% w/w at 25 mM DTPA concentration, while at 100 mM, it increased to 3.83% w/w. The results suggested high bonding strength and stability of 99mTc-PALI-SOL/PALI-ME/PALI-MME. Thus, these formulations were found suitable for pharmacokinetic study of the PALI in rats.
Biodistribution studies (Chow et al., Citation1999; Vyas et al., Citation2006; Jogani et al., Citation2008; Patel et al., Citation2013b) of 99mTc-PALI formulations following IV administration (PALI-ME) and intranasal (PALI-SOL, PALI-ME and PALI-MME) administration on Wistar rats were performed and the radioactivity was estimated at predetermined time intervals up to 8 h. The brain/blood ratio of the PALI at all time points for different formulations were also calculated and recorded in . The pharmacokinetic parameters were calculated from and recorded in .
Figure 1. (A) PALI concentration in rat blood at different time intervals in the following: 99mTc-PALI-ME (IV), 99mTc-PALI-ME (IN), 99mTc-PALI-MME (IN) and 99mTc-PALI-SOL (IN) administrations. (B) PALI concentration in rat brain at different time intervals in the following: 99mTc-PALI-ME (IV), 99mTc-APLI-ME (IN), 99mTc-PALI-MME (IN) and 99mTc-PALI-SOL (IN) administrations.
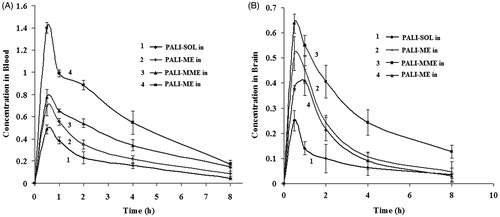
Table 5. Compartmental distribution of 99mTc-PALI-ME (IV), 99mTc-PALI-ME (IN), 99mTc-PALI-MME (IN), and 99mTc-PALI-SOL (IN) at different time intervals in normal wistar ratsa.
Table 6. Pharmacokinetics of 99mTc-PALI-ME (IV), 99mTc-PALI-ME (IN), 99mTc-PALI-MME (IN) and 99mTc-PALI-SOL (IN) at different time intervals in normal wistar ratsa.
PALI concentrations in brain following intranasal administrations of PALI-ME and PALI-MME were found to be significantly higher at all sampling time points compared with IV administration of PALI-ME. The brain-to-blood ratio at 30 mins for PALI-ME (IN) and PALI-MME (IN) was found to be 2–3 times higher as compared with PALI-ME (IV). This finding may be attributed to direct nose-to-brain transport. Reports in the literature revealed that following intranasal administration, preferential nose-to-brain transport bypassing the BBB occurred due to the unique connection between the nose and the CNS (Vyas et al., Citation2006; Jogani et al., Citation2008; Patel et al., Citation2013b). The substantially higher uptake in the brain with intranasal administration signifies a larger extent of selective transport of PALI from nose-to-brain. Many researchers (Vyas et al., Citation2006; Jogani et al., Citation2008; Patel et al., Citation2013b) have reported a unique connection between the nose and the brain and intranasal delivery of drugs to the brain bypassing the BBB (Vyas et al., Citation2006; Jogani et al., Citation2008; Patel et al., Citation2013b). The T1/2 of 2.50–3.28 h (blood), 2.20–4.03 h (brain) and Kel 0.21–0.27 (blood), 0.17–0.32 (brain) were observed irrespective of the routes of administration and the type of the formulations ().
When PALI-ME (IV) was compared to PALI-ME (IN) and PALI-SOL (IN), significantly lower Cmax and AUC were observed. The mucociliary clearance under normal circumstances rapidly clears the instilled formulation. However, when muco-adhesive polymer was incorporated in the formulation (PALI-MME), significant improvement in Cmax and AUC was observed. Comparable AUC to PALI-ME (IV) was achieved with PALI-MME (IN). This demonstrates the value of the muco-adhesive polymer in prolonging the contact time of the formulation with the nasal mucosa. Significantly higher AUC and Cmax for PALI-ME (IN) nasal compared to PALI-SOL (IN) are attributed to ME formulation (Li et al., Citation2002; Zhang et al., Citation2004; Kaur & Kim, Citation2008).
The drug (PALI) targeting efficiency (DTE, %) and brain drug (PALI) direct transport percentage (DTP, %) were also calculated for nasally administered formulations and are shown in . The PALI-MME showed the highest DTE (%) and DTP (%) values among all the three formulations followed by PALI-ME and then PALI-SOL. The 1.74-fold higher DTE (%) and 1.5-fold higher DTP (%) for PALI-MME compared to PALI-SOL show the benefit of the PALI-MME formulation. The higher DTE (%) and DTP (%) suggest that PALI-MME has better brain-targeting efficiency mainly because of substantial direct nose-to-brain transport. These findings are in congruence with the observations reported in literature (Li et al., Citation2002; Zhang et al., Citation2004; Vyas et al., Citation2006; Jogani et al., Citation2008; Kaur & Kim, Citation2008; Patel et al., Citation2013b) that ME increases nose-to-brain uptake of the drugs.
Table 7. Brain targeting efficiency and direct nose-to-brain transport following intranasal administration of 99mTc-PALI-ME, 99mTc-PALI-MME and 99mTc-PALI-SOL.a.
In order to visualize brain uptake following IN and IV administrations of 99mTc-PALI formulation, we used a gamma scintigraphy camera to derive comprehensive biodistribution information. The gamma scintigraphy images of rabbit 0.50 h post IV injection and IN administrations are shown in . Gamma scintigraphy images showed accumulation of significantly higher radioactivity in the rabbit brain after IN administration of PALI compared with IV administration. Among IN formulations, PALI-MME shows higher radioactivity compared with PALI-ME and PALI-SOL. The scintigraphy images were consistent with the results shown in and high uptake of PALI-MME into the brain was observed.
Figure 2. Gamma scintigraphy of anteroposterior (AP) views of rabbit following intravenous administration of 99mTc-PALI-ME (A), intranasal administration of 99mTc-PALI-SOL (B), 99mTc-PALI-ME (C) and 99mTc-PALI-MME (D). Rabbits were administered 100 µCi radioactivity by intravenous and intranasal administration.
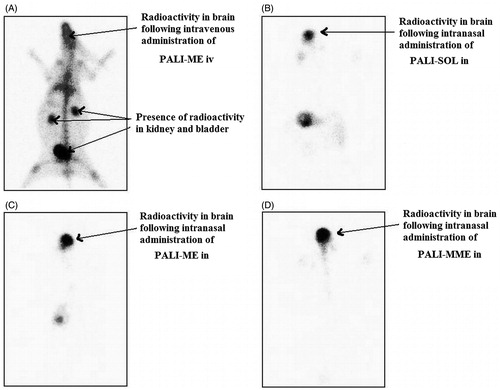
Conclusion
In the present investigation, the utility of ME as carrier for nasal delivery of PALI was studied. All formulations were successfully formulated, characterized and found suitable for intranasal administration. The pharmacodynamic studies (apomorphine-induced compulsive behavior and spontaneous motor activity) revealed that PALI-MME showed lower score for stereotype behavior when compared with PALI-ME, both administered intranasally, indicating the superiority of intranasal PALI-MME over PALI-ME. The reduction in spontaneous motor activity by intranasal administration of PALI-ME and PALI-MME was better when compared with PALI-ME administered intravenously, suggesting superiority of nose-to-brain delivery of PALI over intravenous route of administration. The results demonstrate superior delivery of PALI to the brain via intranasal route from the formulation containing muco-adhesive polymer polycarbophil. The pharmacokinetic studies reveal that the intranasal administration of PALI-MME provide higher brain PALI concentrations compared to the intravenous administration and demonstrated the existence of nose-to-brain transport of PALI. As a consequence of this, decrease in the dose and frequency of administration for drugs is possible to achieve the desired therapeutic activity. Also, intranasal administration of the drugs will avoid unwanted peripheral tissue distribution of the drugs and hence the associated peripheral side effects. These together will improve the therapeutic efficacy of the drugs.
Given the results of this investigation, the in vitro and in vivo studies demonstrated the potential of developed MME for intranasal delivery of PALI and confirm the existence of a transport pathway for a PALI to the brain directly from the nasal cavity. However, clinical benefits to the risk ratio of the formulation developed in this investigation will decide its appropriateness in the clinical practice for the treatment of schizophrenia.
Acknowledgements
Authors are thankful to Torrent Pharmaceutical Ltd. (India) for providing the gift sample of pure powder of drugs, Gattefosse (Saint-Priest, France), Colorcon (Asia) Pvt. Ltd. (Mumbai, India), BASF (Mumbai, India), Noveon (Cleveland, USA) for readily providing gratis samples of excipients, to Veterinary Nuclear Medicine Centre, Bombay Veterinary College, Mumbai, to Institute of Nuclear Medicine and Allied Science (INMAS), New Delhi, for permitting us to carry out experimental work at INMAS, to Sophisticated Instrumentation Center for Applied Research and Testing (SICART), Vallabh Vidyanagar, for providing facilities and research assistant.
Declaration of interest
All India Council for Technical Education (AICTE), New Delhi is gratefully acknowledged for financial support (F.No.8023/BOR/RID/RPS-147). The author(s) confirm that this article content has no conflicts of interest.
References
- Babbar AK, Singh AK, Goel HC, et al. (2000). Evaluation of 99mTc-labeled Photosan-3, a hematoporphyrin derivative, as a potential radiopharmaceutical for tumor scintigraphy. Nucl Med Biol 27:419–26
- Braso A, Princep M, Schmid M, et al. (2003). Developmental pharmacology: behavior assay in antipsychotics. Methods Find Exp Clin Pharmacol 25:1–2
- Canuso CM, Youssef EA, Bossie CA, et al. (2008). Paliperidone extended-release tablets in schizophrenia patients previously treated with risperidone. Int Clin Psychopharm 23:209–15
- Chow HS, Chen Z, Matsuura GT. (1999). Direct transport of cocaine from the nasal cavity to brain following intranasal cocaine administration in rats. J Pharm Sci 88:754–8
- Eckelman WC. (1995). Radiolabeling with technetium-99m to study high capacity and low-capacity biochemical systems. Eur J Nucl Med 22:249–63
- Elshafeey AH, Bendas ER, Mohamed OH. (2009). Intranasal microemulsion of sildenafil citrate: in vitro evaluation and in vivo pharmacokinetic study in rabbits. AAPS PharmSciTech 10:361–4
- Fazil M, Md S, Haque S, et al. (2012). Development and evaluation of rivastigmine loaded chitosan nanoparticles for brain targeting. Eur J Pharm Sci 47:6–15
- Jogani VV, Shah PJ, Mishra P, et al. (2008). Intranasal mucoadhesive microemulsion of tacrine to improve brain targeting. Alzheimer Dis Assoc Disord 22:116–24
- Kaur P, Kim K. (2008). Pharmacokinetics and brain uptake of diazepam after intravenous and intranasal administration in rats and rabbits. Int J Pharm 364:27–35
- Kulkarni SK, Jisheph P. (1998). Psychopharmacological profile of sitone granules, a herbal drug preparation. Indian drug 35:536–44
- Le M, Simon H. (1991). Mesocorticolimbic dopaminergic network functional and regulatory roles. Physiol Rev 71:155–234
- Li L, Nandi I, Kim KH. (2002). Development of an ethyl laurate-based microemulsion for rapid-onset intranasal delivery of diazepam. Int J Pharm 237:77–85
- Mahajan HS, Mahajan MS, Nerkar PP, Agrawal A. (2014). Nanoemulsion-based intranasal drug delivery system of saquinavir mesylate for brain targeting. Drug Deliv 21:148–54
- Md S, Khan RA, Mustafa G, et al. (2013). Bromocriptine loaded chitosan nanoparticles intended for direct nose to brain delivery: pharmacodynamic, pharmacokinetic and scintigraphy study in mice model. Eur J Pharm Sci 48:393–405
- Nasrallah HA, Targum SD, Tandon R, et al. (2005). Defining and measuring clinical effectiveness in the treatment of schizophrenia. Psychiatr Serv 56:273–82
- Patel RB, Patel MR, Bhatt KK, Patel BG. (2010). HPTLC Method development and validation: quantification of paliperidone in formulations and in vitro release study. Anal Methods 2:525–31
- Patel RB, Patel MR, Bhatt KK, Patel BG. (2013a). Paliperidone loaded mucoadhesive microemulsion in treatment of schizophrenia: formulation consideration. J Pharm Innov 8: 195–204
- Patel RB, Patel MR, Bhatt KK, et al. (2013b). Risperidone microemulsion for transnasal delivery: pharmacodynamic and pharmacokinetic evaluation. Pharm Nanotechnol 1:44–53
- Porecha S, Shah T, Jogani V, et al. (2009). Microemulsion based intranasal delivery system for treatment of insomnia. Drug Deliv 16:128–34
- Salamone JD, Correa M, Mingote SM, Weber SM. (2005). Beyond the reward hypothesis: alternative functions of nucleus accumbens dopamine. Curr Opin Pharmacol 5:34–41
- Shibuya TT, Nishimori MH. (1982). Behavioural pharmacological studies in the monkey with DD-3480. Int J Clin Pharmacol Ther Toxicol 20:251–4
- Shinde RL, Jindal AB, Devarajan PV. (2011). Micromeulsions and nanoemulsions for targeted drug delivery to the brain. Curr Nanosci 7:119–33
- Vyas TK, Babbar AK, Sharma RK, et al. (2006). Intranasal mucoadhesive microemulsions of clonazepam: preliminary studies on brain targeting. J Pharm Sci 95:570–80
- Wolfgang L, Heidrun P. (2005). Blood–brain barrier active efflux transporters: ATP-binding cassette gene family. NeuroRx 2:86–98
- Zacher JL, Grady SE. (2007). Paliperidone extended-release tablets (Invega). Psychopharm Rev 42:51–8
- Zhang Q, Jiang X, Jiang W, et al. (2004). Preparation of nimodipine-loaded microemulsion for intranasal delivery and evaluation on the targeting efficiency to the brain. Int J Pharm 275:85–96