Abstract
Objective: The purpose of this study was to formulate stable Ganoderma lucidum (GLT) nanogels suitable for topical delivery with a view to improve the therapeutic effect for frostbite.
Methods: GLT nanosuspensions were formulated using the high-pressure homogenization technique and then suitably gelled for characterized. In order to confirm the advantages of GLT nanogel for dermal application, skin permeation studies in vitro and pharmacodynamic evaluation in vivo were studied and compared with GLT–carbopol gel.
Results: The particle size analysis and SEM studies revealed that GLT nanosuspensions were still stably kept their particle size after suitably gelled by carbopol preparation. The drug content, pH, and spreadability of the GLT nanogel was found to be 99.23 ± 1.8%, 6.07 ± 0.1, and 26.42 (g·cm)/s, which were within acceptable limits. In vitro permeation studies through rat skin indicated that the amount of GLT permeated through skin of GLT nanogel after 24 h was higher than GLT–carbopol gel, and GLT nanogel increased the accumulative amount of GLT in epidermis five times than GLT–carbopol gel. No oedema and erythema were observed after administration of GLT nanogel on the rabbits' skin. Pharmacodynamic study showed that GLT nanogel was more effective than GLT–carbopol gel in treatment of frostbite.
Conclusion: The GLT nanogel possess superior therapeutic effect for frostbite compared with the GLT–carbopol gel, which indicates that nanogels are eligible for the use as a suitable nanomedicine for dermal delivery of poorly soluble drugs such as GLT.
Introduction
Frostbite is a local freezing injury involving damage to skin and tissues due to extreme cold. It occurs when exposure to cold external temperature results in frozen tissue, sometimes leading to extensive tissue loss and disability (Auerbach et al., Citation2013). Historically, frostbite has been a disease of wars, but now it is a hazard for high altitude mountaineers, skiers, and others who venture outdoors in cold weather for recreation or for work. It is also a danger for homeless or displaced persons, especially during natural disasters or forced migrations. Frostbite is most commonly observed in the fingers/hands, toes/feet, nose, cheeks, and ears because they are exposed, peripheral, and difficult to protect (Zafren, Citation2011). Frostbite often causes tissue damage and necrosis that requires eventual amputation, with the distal extremities often being at highest risk (Johnson et al., Citation2011). At present, there are no commercially available and special drugs in the treatment of frostbite. Hence, an effective drug is highly desirable for frostbite treatment.
In the previous studies, triterpenoids isolated from Ganoderma lucidum (GLT) were found to have therapeutic effect for frostbite (Han et al., Citation2012). Ganoderma lucidum is a popular medicinal mushroom, which has been used in the Traditional Chinese medicine for the prevention or treatment of a variety of diseases such as cancer, hepatopathy, arthritis, hypertension, and neurasthenia (Leung, Citation2002; Luo & Lin, Citation2002; Wu et al., 2013a, b). GLT, including ganoderic acids, ganoderenic acids, sanedermic acids, lucidenic acids, ganederals, ganolucidic acids, ganederiols, ganederols, ganodosterone, and ganodermatriol (Boh et al., Citation2007; Wei, Citation2010), is responsible for the major medicinal properties. Our study showed that GLT have therapeutic effect for frostbite, which may be related to their bioactivities of anti-inflammatory (Ko et al., Citation2008; Dudhgaonkar et al., Citation2009), antinociception (Koyama et al., Citation1997), antimicrobial (Karwa & Rai, Citation2012; Li et al., Citation2012), antioxidation (Smina et al., Citation2011), resisting histamine release (Kohda et al., Citation1985; Zhang et al., Citation2010), immunoregulation (Boh, Citation2013), etc. However, GLT exhibit an unsatisfactory therapeutic effect for frostbite due to its poor solubility and the limitation in permeation of effective concentrations of drugs into the skin for desired therapeutic action. It needs to be incorporated into a proper vehicle to increase the solubility of GLT and maintain therapeutically relevant concentrations of GLT in the epidermis/dermis.
Topical gels for dermal application have been reported in many literatures (Cho et al., Citation2008; Song & Shin, Citation2009; Patel et al., Citation2012; Aerry et al., Citation2013; Rehman & Zulfakar, Citation2014). It has been proved to be a beneficial vehicle for topical drug delivery or for the localized drug action on skin (Rehman & Zulfakar, Citation2014). However, the permeation challenge is pronounced in case of poorly soluble drug molecules. Penetration enhancers were used to alert permeability of the drug through the skin (Zafar et al., Citation2010; Mohammed et al., Citation2013), but is restricted by the skin irritation that may be caused by some of these compounds. Supersaturation was also employed to achieve drug enhancement (Inoue & Sugibayashi, Citation2012), but it always emerged crystallization of drug molecules immediately after formulation or even during storage.
Recently, nanosuspensions, as a particle size reduction's technology, have been successfully used to tackle the formulation problem of poorly soluble drugs for site-specific dermal drug delivery (Mitri et al., Citation2011; Lai et al., Citation2013). Nanosuspensions are sub-micron colloidal dispersions of pure drug particles stabilized by surfactants, polymers, or a mixture of both (Shen et al., Citation2013). Production techniques of nanosuspensions are classified as bottom-up methods, top-down methods, or a combination technology of the two methods mentioned (Shen et al., Citation2013). Remarkable nanosuspensions properties including increased drug solubility, high drug loading, low incidence of side effects by the excipients, simple production process, easy scale-up, and universal adaptivity enable its applications in the formulation of large amounts of poorly soluble compounds (Gao et al., Citation2011; Liu et al., Citation2011).
In this paper, a novel GLT nanogel was developed with the aim of enhancing GLT poor water solubility and improving its therapeutic effect for frostbite. GLT nanosuspension was first prepared by high-pressure homogenization technique and then incorporated in carbopol gel to formulate GLT nanogel. Then GLT nanogel was evaluated by particle size distribution, zeta potential analysis, SEM, in vitro percutaneous penetration, skin irritation test, and in vivo pharmacodynamic evaluation.
Materials and methods
Materials
The fruiting body of G. lucidum was kindly obtained from Beijing Union Pharmaceutical Factory and authenticated by Dr. Yuan (302 Military Hospital of China, Beijing, China). GLT (22.7% ganoderic acid A, 11.3% ganoderic acid B, 9.2% ganoderic acid C2, 7.9% ganoderic acid E, 6.5% ganoderic acid G, 9.8% ganoderenic acid B, 9.1% ganoderenic acid E, 6.3% lucidenic acid A, 7.3% lucidenic acid H, and 5.6% ganederiol F) were prepared in the laboratory of Dr. Yuan. Sodium dodecyl sulfate (SDS), Kollidon® 30 (PVP K-30), and poloxamer F68 were obtained from the BASF Corp. (Ludwigshafen, Germany). Tween 80 was purchased from Sigma Aldrich Chemicals Pvt. Ltd. (St Louis, MO). Lecithin (PC > 95%) was obtained from Shanghai TaiWei Pharmaceutical Industry Co. Ltd. (Shanghai, China). Carbopol 940P was purchased from Guangzhou Bo Feng Chemical Co., Ltd. (Guangzhou, China). All other reagents were of analytical grade.
Preparation of GLT nanosuspension
GLT nanosuspension was prepared by high-pressure homogenization technique. GLT powder (1%, w/v) was grinded in a mortar and then dispersed in a stabilizer solution. Then the dispersion was first processed using a high shear homogenizer (Ultra-Turrax® T25, IKA, Germany) at 13 300 rpm for 5 min and then homogenized at high pressure using a piston-gap high pressure homogenizer (EmulsiFlex-C3, Avestin Inc., Ottawa, Canada) to obtain the GLT nanosuspension. Different formulations and process parameters optimized during formulation development were stabilizer or combination of stabilizers, stabilizer concentration, homogenization pressure, and homogenization cycles (). For the development of GLT nanosuspension, PVP K30, SDS, Tween 80, Poloxamer F68, Lecithin, and their combinations were tried as stabilizers to get the desired particle size distribution. A pressure of 1000, 1200, or 1500 bar and cycle numbers of 10, 15, 20, or 25 cycles were tried during the high homogenization process.
Table 1. Batches of GLT nanosuspension.
For solid-state characterization, the optimized GLT nanosuspension was pre-frozen at −80 °C for 24 h (DW-86L388, Haier Co., Ltd., Shandong, China) followed by freeze-dried using a lyophilizer (FD5-series, Gold SIM, Beijing, China) at −50 °C and at 0.10 mbar of pressure for 72 h till solid powder was obtained. Mannitol as a cryoprotectant with the concentrations at 5% (w/v) in water was added to nanosuspension prior to freeze-drying.
Preparation of GLT nanogel
A topical gel containing GLT nanosuspension was formulated according the literature methods (Venkataraman & Nagarsenker, Citation2013) with a modification. Briefly, carbopol 940P (0.5%, w/w) was dispersed in distilled water and kept aside for 1 h for swelling. The obtained freeze dried GLT nanosuspension powders (5%, w/w) were added into the swelled carbopol 940P dispersions under continuous stirring at 800 rpm using a magnetic stirrer (85-2, Changzhou Guohua Electric Appliance Co., Ltd., Jiangsu, China) and mixed evenly. Triethanolamine was then added under gentle stirring and the pH was adjusted to 5.5–6.5. The gel was allowed to stand 24 h at room temperature to equilibrate. Meanwhile, GLT coarse powders were used to prepare the GLT–carbopol gel.
Characterization of GLT nanogel
Particle size and zeta potential analyses
The volume average particle size and polydispersity index (PI) of GLT nanosuspension during process optimization were determined by photon correlation spectroscopy (PCS) using a Zeta-sizer (3000SH, Malvern Instruments Ltd., Malvern, UK). Zeta potential of optimized formulation was estimated by determining the particle electrophoretic velocity using the same instrument.
The same method was used to measure the particle size distribution of the GLT nanogel. The gel network was destroyed by dilution with distilled water and then analyzed at room temperature.
SEM
SEM photographs were taken by scanning electron microscopy (SEM, S-4800, Hitachi Technologies Corporation, Ibaraki-ken, Japan). The SEM samples of coarse GLT, freeze-dried GLT nanosuspension, and GLT nanogel were fixed on the metal stub using a double-sided adhesive tape and then coated with gold in vacuum by a sputter coater. The scanning electron microscope was operated at a voltage of 5 kV.
Determination of drug content, spreadability, and pH
In order to quantify the total drug content of nanogel after production, an amount of GLT nanogel was diluted with methanol and stirred for 12 h in order to completely extract GLT. A filtered sample was then diluted appropriately and analyzed by the HPLC method. GLT content was calculated as follows:
Spreadability of the gel formulation was determined as per procedure described by Chaudhary et al. (Citation2013).
The pH of the nanogel was recorded using the digital pH meter (pHS-25; Nanjing Everich Medicare Import and Export Co., Ltd., Nanjing, China) at room temperature. About 0.5 g of the nanogel formulation was weighed in a 200 mL glass beaker, and then volume was made up with distilled water to 100 mL.
In vitro skin permeation and retention study
Franz diffusion cells with an effective diffusion area of 1.77 cm2 and a receptor volume of 17.5 mL were used to evaluate the percutaneous permeation from the developed formulations. Abdominal rat skin was excised. Its hair, adhering fat, and other visceral tissue were removed carefully and washed with isotonic NaCl. The excised skin was then mounted on the Franz diffusion cells with the stratum corneum (SC) side to the donor compartment. The receptor compartment was filled with phosphate buffer saline (PBS, pH 7.4), which was continuously stirred at 500 rpm with magnetic stirrer (SH-2, Beijing Jinbeide Industrial And Trading Co., Ltd., Beijing, China) and kept at 32 ± 0.5 °C to simulate the skin temperature. GLT nanogel (0.5 g, 25 mg of GLT) and GLT–carbopol gel (0.5 g, 25 mg of GLT) were placed onto the skin in the donor compartment. At predetermined time intervals (1, 2, 3, 5, 7, 9, 12, and 24 h), 1 mL samples were withdrawn from the receptor compartment using a syringe needle and immediately replaced with the same volume of pre-thermostated (32 °C) receptor solution to ensure sink conditions. All the collected samples were filtered through 0.45 µm millipore filter and analyzed for GLT content by HPLC. After 24 h, the skin surface of specimens was gently washed several times with PBS (pH 7.4) to remove any formulations remaining. To extract the drug, the skin samples were cut into small pieces and homogenized in absolute ethanol to extract all the retained GLT. All the samples were then centrifuged at 8000 rpm for 20 min to remove any skin debris and the supernatants was then filtered and assayed for GLT content by HPLC.
The content of ganoderic acid A (GA, an indexical component found in GLT, 22.7%, w/w) was measured using a Prominence LC system (Shimadzu Corporation, Kyoto, Japan) equipped with a diode array detector (SPD-M20A), a quaternary pump (LC-20AT), an auto sampler (SIL-20A), a degassing unit (DGU-20A5R), and Shimadzu CBM-20A station for data analysis. GA was separated at 15 °C on an Alltima C18 column (250 mm × 4.6 mm, 5 μm, Alltech, Beijing, China). The mobile phase was composed of (A) acetonitrile and (B) 0.03% formic acid solution using a gradient elution of 25–35% A at 0–35 min, 35–45% A at 35–55 min, 45–55% A at 55–65 min, 55–25% A at 65–66 min, and 25% A at 66–80 min with a flow rate of 1.0 mL/min. The samples were put into HPLC vials and 10 μL automatically injected into the HPLC system and the detector wavelength was set at 254 nm.
Skin irritation study
The evaluation of skin irritation potential of GLT nanogel was carried out using the Draize patch test on rabbits (Joshi & Patravale, Citation2006). For this study, three white New Zealand rabbits weighing 2.5–3 kg were used. Hairs were depleted from the back and sides of the rabbits with the help of depilatories 24 h prior to the application of formulation, and the GLT nanogel (1g) was applied on the hair free skin of rabbits by uniform spreading within the area of 2 cm × 2 cm. Gel was applied once a day for 7 days and the skin was observed for any visible change such as redness, edema, and skin rash according to the Draize scale.
Pharmacodynamic efficacy of GLT nanogel
Sprague–Dawley rats (adult male, 200–220 g) used in the experiments were provided by the Experimental Animal Center of Military Medical Sciences (Beijing, China). The rats were housed at a room temperature of 23 ± 1 °C with a 12 h-light and 12 h-dark cycle (lights on from 6:00 am to 6:00 pm). Food and water were available ad libitum. The rats were treated in compliance with the Principles of Laboratory Animal Care and the Guide for the Care and Use of Laboratory Animals. Experiments followed protocol approved by 302 Military Hospital of China Institutional Animal Care and Use Committee.
The rat's model for frostbite was made according the literature method (Wang & Li, Citation2010; Jiao et al., Citation2013). Briefly, the dorsal skin surface with a diameter of 2.5 cm of each rat was shaved and cleaned under light ether anesthesia 24 h prior to experiment. The rat was anaesthetized by receiving intraperitoneal injection of 10% (w/v) chloral hydrate (3 mL/kg). A round sheet of steel with a diameter of 2.5 cm and a thickness of 2 mm was completely immersed in liquid nitrogen tanks for at least 15 min to make it fully cooled to −196 °C. The steel sheet was then took out and immediately and tightly attached to the hair free skin of anesthetic rat for 5 s to make topical frostbite.
The rats were randomly divided into four groups with eight animals in each group: (A) Normal control group; (B) Model group; (C) GLT–carbopol gel group; (D) GLT nanogel group. The rats of groups B–D were made frostbite injury on skin as described above. After 12 h frostbite, the drugs were applied on the frostbitten skin by uniform spreading within the area of frostbite once daily for seven consecutive days. The rats of group D were treated with GLT nanogel (1 g, 50 mg of GLT), group C with GLT–carbopol gel (1 g, 50 mg of GLT), and groups A and B with no topical treatment, respectively. The apparent image of rats' skin 6 h after last application of drugs was recorded by a digital camera (Coolpix S600, Nikon, Tokyo, Japan) and then was processed by RdfSnap software and MiE image micro-processing software. The percentage of survival area (Psa) of rats' skin before frostbite and 6 h after last application of drugs used for evaluation of therapeutic effect of frostbite was calculated as follows:
where S0 is the survival area of rats’ skin before frostbite, R is 1.25 cm, St is the survival area of rats'; skin 6 h after last application of drugs.
For the histopathological studies, 12 h after last application of drugs, the rats were sacrificed by anesthesia. The rats' skin were removed and fixed in 10% formalin. The fixed tissues were then dehydrated by different concentrations of ethanol gradient, vitrification by dimethylbenzene, and then embedded in paraffin. After the pathological section was prepared, the morphological change in the frostbite tissues' sections stained with hematoxylin and eosin (H&E) was observed by a Slide Scanner (Leica SCN400, Leica Microsystems GmbH, Wetzlar, Germany).
Statistical analysis
Statistical analysis was performed using one-way analysis of variance (ANOVA) followed by the least significant difference post-hoc test. Differences between experimental groups were considered significant at p < 0.05. All data are expressed as the mean ± standard deviation (SD).
Results and discussion
Preparation of GLT nanogel
Screening of stabilizer for GLT nanosuspension
Stabilizers with functions of wetting the drug particles thoroughly and preventing Ostwald's ripening play an important role in the formulation of nanosuspension (Patravale et al., Citation2004). In order to obtain the GLT nanosuspension having a diameter in nanometer range with narrow size distribution, five stabilizers including three kinds of surfactants and two kinds of polymers were studied in this paper (, S-1–S-5). Among them, the GLT nanosuspension stabilized by Tween 80 (S-1) and Poloxamer F68 (S-4), respectively, showed a significant smaller particle size and lower PI value as compared with the GLT nanosuspension stabilized by other stabilizers (, S-1, S-2, and S-4; p < 0.05). For further improvement in particle size distribution, various ratios of Tween 80 to Poloxamer F68 were tried (, S-6–S-8). As shown in , the GLT nanosuspension containing 0.2% (w/v) Tween 80 and 0.1% (w/v) Poloxamer F68 showed significant reduction and narrow distribution of particle size (S-8) compared with that of other GLT nanosuspension containing different ratios of Tween 80 to Poloxamer F68 (S-6 and S-7; p < 0.05).
Figure 1. The particle size and PI value of different formulations of GLT nanosuspension with different stabilizers (S-1–S-8, mean ± SD, n = 3).
▾ p < 0.05 the particle size of S-1 compared with that of S-2, S-3, S-5, S-6, S-7, S-8; ♦ p < 0.05 the particle size of S-4 compared with that of S-2, S-3, S-5, S-6, S-7, S-8; ★ p < 0.05 the particle size of S-8 compared with that of S-1, S-2, S-3, S-4, S-5, S-6, S-7; ▽ p < 0.05 the PI value of S-1 compared with that of S-2, S-3, S-5, S-6, S-7, S-8; ⋄ p < 0.05 the PI value of S-4 compared with that of S-2, S-3, S-5, S-6, S-8; ☆ p < 0.05 the PI value of S-8 compared with that of S-1, S-2, S-3, S-4, S-5, S-7.
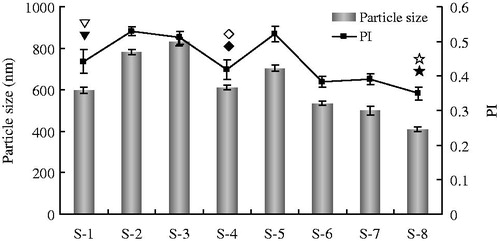
Screening of process parameters for GLT nanosuspension
During the high-pressure homogenization process, homogenization pressure and homogenization cycles were identified as critical factors to determine the particle size characteristics of nanosuspension (Yue et al., Citation2013). In this study, GLT nanosuspension was prepared by the high-pressure homogenization method. Different homogenization pressure and homogenization cycles were investigated with particle size and PI value as evaluation indexes (, S-8–S-13). The results are shown in . With the increase in homogenization pressure from 1000 bar to 1200 bar, the particle size and PI had reduced to 299 nm and 0.27 (S-9) from 408 nm and 0.35 (S-8), respectively. However, a further increase in homogenization pressure from 1200 bar to 1500 bar did not show any significant difference in particle size and PI (S-9 and S-10). Then the GLT nanosuspension prepared was subjected to 15, 20, or 25 cycles of 1200 bar pressure. As shown in , the particle size did not change significantly after the 10th cycle (S-9), whereas the PI decreased with the increase in homogenization cycles until to the 20th cycle (S-12). The results obtained are in agreement to the previous which reported that the majority of the particles reach smaller size relatively fast and further homogenization cycles have little effect on the mean diameter of the particle but reduce the PI by eliminating the remaining few large crystals (Venkataraman & Nagarsenker, Citation2013). It was concluded that 20 cycles of 1200 bar pressure is the optimum pressure cycle required to produce 1% GLT nanosuspension with 0.2% Tween 80 and 0.1% Poloxamer F68.
Figure 2. The particle size and PI value of different formulations of GLT nanosuspension with different process parameters of homogenization (S-8–S-13, mean ± S.D., n = 3). ▾ p < 0.05 the particle size of S-8 compared with that of other formulations; ▽ p < 0.05 the PI value of S-8 compared with that of other formulations; ☆ p < 0.05 the PI value of S12 compared with that of S-8, S-9, S-10, S-11.
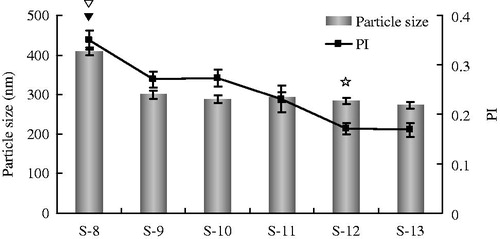
Characterization of GLT nanogel
The prepared GLT nanogel was opaque, light gray, smooth, and homogenous of semisolid consistency without sticky skin feel. The particle size and PI of the optimized GLT nanosuspension were 283 ± 7 nm and 0.171 ± 0.011, respectively. The zeta potential of optimized batch (S-12) was found to be −32.1 ± 2.6 mV indicating electrostatic and steric stabilization by Tween 80 and Poloxamer F68. There was a marginal increase in the particle size after gelling of up to 297 ± 8 nm for the GLT nanoparticles, which indicated that the GLT nanoparticles were still stably kept their particle size after it was entrapped in the gel.
The morphologies of GLT raw material, freeze-dried GLT nanosuspension, and GLT nanogel are shown in . SEM images revealed apparent differences in the morphologies of the samples. The GLT raw material was appeared to be irregular with a particle size of 5–50 µm, while the GLT nanosuspensions presented a granular shape with a diameter of 100–500 nm (). This might be ascribed to the high pressure homogenization process of the coarse drug. During high-pressure homogenization process, cavitation effect, shear force, and collisions between the drug particles easily disintegrated the drug microparticles into nanometer range (Lai et al., Citation2009). Well-dispersed GLT nanoparticles with a size of about 300 nm were observed in GLT nanogel ().
Figure 3. SEM images of (A) raw GLT, (B) freeze-fried GLT nanosuspension, and (C) GLT nanogel. Arrows show GLT nanoparticles.
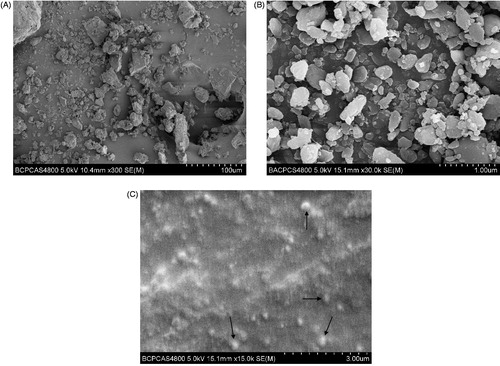
The drug content of the GLT nanogel was found to be 99.23 ± 1.8% and the pH of GLT nanogel was found to be 6.07 ± 0.1, which was within acceptable limits. The value of spreadability was 26.42 (g· cm)/s, which indicated that the gel was easily spreadable by small amount of shear and possessed acceptable bioadhesion.
In vitro permeation study and skin deposition studies
The amount of GA penetrate through the skin versus time and deposit in the skin after applying GLT nanogel and GLT–carbopol gel are shown in . As expected, the amount of GA permeated from GLT nanogel was obviously higher compared with GLT–carbopol gel (). The permeated amounts of GA from GLT nanogel (67.69 μg) was about three times enhancement over GLT–carbopol gel (23.52 μg) after 24 h. GLT–carbopol gel is a carbopol-based gel of GLT representing a simple, aqueous system with GLT dispersed in the matrix. Permeation of GLT from gel is thus determined by permeation properties of the drug. The superior permeation results obtained from the gel system produced from nanosuspension can be explained by the larger surface area and potentially higher dissolution velocity of the small nanocrystals, hence promoting penetration of GLT as a lipophilic compound through the skin. Another reason was the presence of surfactants in the nanogel, which can decrease the crystallinity of the intracellular lipid bilayers of the skin that act as a permeation enhancer (Kumbhar et al., Citation2013).
Figure 4. Amount of GA penetrate through skin (A) and in the skin (B) after applying GLT nanogel and GLT–carbopol gel for 24 h (mean ± SD, n = 3).
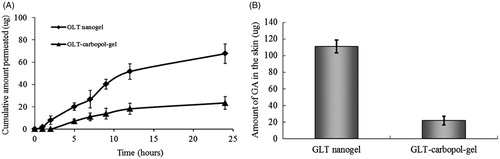
The skin deposition study showed that GLT nanogel has higher deposition potential for the GA as compared with the carbopol gel containing coarse drug only. There was a five-fold increase in deposition of GA from nanogel (111.08 μg) compared with GLT–carbopol gel (21.67 μg) (). It was maybe that the smaller size of nanoparticles could ensure close contact with the stratum corneum and increase the drug amount penetrating into the skin (Jana et al., Citation2014). According to the previous literatures, if the size of the particles is higher than 5 μm, they do not penetrate into the lipid layers of the stratum corneum (Keck & Müller, Citation2006), however, small particles at a diameter of approximately 750 nm penetrated most efficiently into the follicles, a process which could even be enhanced by mechanical massage of the particles into the skin (Lademann et al., Citation2007). In GLT nanogel, after 24 h, only 67.69 μg GA permeated through the skin, but 111.08 μg GA located in the skin. The improved skin uptake of GLT might result from the general adhesiveness to skin surfaces of nanoparticles (Müller & Jacobs, Citation2002), which leads to a prolonged residence and contact time in the skin, thereby increasing its skin deposition. For the development and optimization of pharmaceutical dermal products and cosmetics, a certain penetration into the skin is desired, especially if the active compound should be localized in the skin but not systemically absorbed (Mitri et al., Citation2011; Chen et al., Citation2013).
Skin irritation study
The results of the skin irritation study indicated that GLT nanogel exhibited no irritation, even after 7 days of application. Therefore, the developed GLT nanogel can be used for topical application.
Pharmacodynamic efficacy of GLT nanogel
To confirm the effect of GLT nanogel in the treatment of frostbite, pharmacodynamics trial was carried out in rats and compared with that of GLT–carbopol gel.
As shown in , the Psa of rats' skin of the normal control group was 100%. Compared with the normal control group, the Psa of rats' skin in the model group was 53.24 ± 11.49%, which was significantly different from the normal control group (p < 0.05), indicating that the rats' skin in the model group was made significant injury by attached with steel sheet that previously immersed in liquid nitrogen. Compared with the model group, the GLT nanogel group and the GLT–carbopol gel group could significantly increase Psa of rats' skin (p < 0.05). Moreover, the Psa of rats' skin in the GLT nanogel group was higher than that of GLT–carbopol gel group (p < 0.05). Experimental evidence obtained in the present study indicated that the GLT nanogel was more effective than GLT–carbopol gel in treatment of frostbite.
Table 2. Therapeutic effect of GLT nanogel and GLT–carbopol gel on frostbite of rats' skin.
Pathological change of skin tissue in each group is displayed in . The epidermis of rats' skin in the normal control group had clear layer and stratum corneum was clearly visible; hair follicles and arrectores pilorum were observed in dermis and the dermis had no edema, hemorrhage, and inflammatory cell infiltration, the subcutaneous tissue had no necrosis and other changes (). Compared with the normal control group, an obvious injury in rats' skin in the model group includes significant epidermal cells diabrosis and defect, subcutaneously visible congestion, edema, acute, and chronic inflammation and increase in inflammatory cell infiltration (). The application of GLT nanogel and GLT–carbopol gel resulted in significant recovery of frostbite in different sections of the rats' skin. In the GLT–carbopol gel group, vascular congestion, edema, and inflammatory cell infiltration were visible in dermis (). While there were only subcutaneous edema and inflammatory cell infiltration in the GLT nanogel group, skin appendages showed no obvious morphological changes (). The results showed that GLT nanogel was more effective than GLT–carbopol gel in treatment of frostbite, which is in good agreement with the results of Psa.
Figure 5. Histological photomicrographs of rats' skin (H&E stain × 40, (A) Normal control group; (B) Model group; (C) GLT–carbopol gel; (D) GLT nanogel).
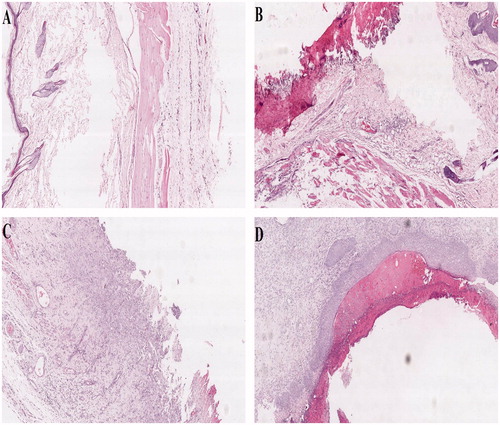
The GLT could significantly increase survival area and improve the degree of pathological change of skin tissue of the rats' skin with frostbite. The reasons that GLT nanogel was more effective than GLT–carbopol gel in treatment of frostbite can be explained as follows: the small particle size of GLT nanogel provides a large specific surface area, which resulted in enhancement of drug bioadhesion to the skin surface and prolonged contact time with the skin, thus improving its skin deposition and absorption, ultimately enhancing its therapeutic effect for frostbite.
Conclusion
In this study, GLT nanogel was successfully developed and showed suitable characteristics for topical delivery. The results of particle size analyses and SEM showed that GLT nanosuspensions was still stably kept their particle size after suitably gelled by carbopol preparation. In vitro permeation studies through rat skin indicated that the amount of GA permeated through skin of GLT nanogel after 24 h was higher than GLT–carbopol gel, and GLT nanogel increased the accumulative amount of GA in epidermis five times than GLT–carbopol gel. A significant improvement in frostbite treatment effect was found after the application of GLT nanogel compared with GLT–carbopol gel. Overall results show that nanogel is eligible for use as a suitable nanomedicine for dermal delivery of poorly soluble drugs such as GLT.
Declaration of interest
The authors report no conflict of interest. The authors alone are responsible for the content and writing of the paper. This study was supported by the Beijing Natural Science Foundation of China (No. 7122176), the National Natural Science Foundation of China (No. 81102821), and the National Key New Drugs Innovation Foundation (Nos. 2014ZX09J14106-01A and CWS11J165).
References
- Aerry S, De A, Kumar A, et al. (2013). Synthesis and characterization of thermoresponsive copolymers for drug delivery. J Biomed Mater Res A 101:2015–26
- Auerbach LJ, Galvez MG, De Clerck BK, et al. (2013). A novel mouse model for frostbite injury. Wilderness Environ Med 24:94–104
- Boh B. (2013). Ganoderma lucidum: a potential for biotechnological production of anti-cancer and immunomodulatory drugs. Recent Pat Anticancer Drug Discov 8:255–87
- Boh B, Berovic M, Zhang J, Zhi-Bin L. (2007). Ganoderma lucidum and its pharmaceutically active compounds. Biotechnol Annu Rev 13:265–301
- Cho CW, Choi JS, Shin SC. (2008). Development of the ambroxol gels for enhanced transdermal delivery. Drug Dev Ind Pharm 34:330–5
- Chaudhary H, Rohilla A, Rathee P, Kumar V. (2013). Optimization and formulation design of carbopol loaded Piroxicam gel using novel penetration enhancers. Int J Biol Macromol 55:246–53
- Chen S, Liu W, Wan J, et al. (2013). Preparation of coenzyme Q10 nanostructured lipid carriers for epidermal targeting with high-pressure microfluidics technique. Drug Dev Ind Pharm 39:20–8
- Dudhgaonkar S, Thyagarajan A, Sliva D. (2009). Suppression of the inflammatory response by triterpenes isolated from the mushroom Ganoderma lucidum. Int Immunopharmacol 9:1272–80
- Gao L, Liu G, Wang X, et al. (2011). Preparation of a chemically stable quercetin formulation using nanosuspension technology. Int J Pharm 404:231–7
- Han J, Zhou X, Yuan HL, et al. (2012). Research on the therapeutic effect of compound Lingzhi cream for mild frostbite on rabbit ears. Chin Pharm J 47:689–92
- Inoue K, Sugibayashi K. (2012). In vivo enhancement of transdermal absorption of ketotifen by supersaturation generated by amorphous form of the drug. Eur J Pharm Sci 47:228–34
- Joshi M, Patravale V. (2006). Formulation and evaluation of nanostructured lipid carrier (NLC)-based gel of Valdecoxib. Drug Dev Ind Pharm 32:911–18
- Johnson AR, Jensen HL, Peltier G, DelaCruz E. (2011). Efficacy of intravenous tissue plasminogen activator in frostbite patients and presentation of a treatment protocol for frostbite patients. Foot Ankle Spec 4:344–8
- Jiao MK, Hu J, Jiao L, et al. (2013). The effect of low frequency pulsed electromagnetic fields on blood circulation in frostbite tissue. Chinese J Med Phys 30:3946–9
- Jana S, Manna S, Nayak AK, et al. (2014). Carbopol gel containing chitosan-egg albumin nanoparticles for transdermal aceclofenac delivery. Colloids Surf B Biointerfaces 114:36–44
- Karwa AS, Rai MK. (2012). Naturally occurring medicinal mushroom-derived antimicrobials: a case-study using Lingzhi or Reishi Ganoderma lucidum (W. Curt.:Fr.) P. Karst. (higher Basidiomycetes). Int J Med Mushrooms 14:481–90
- Kohda H, Tokumoto W, Sakamoto K, et al. (1985). The biologically active constituents of Ganoderma lucidum (Fr.) Karst. Histamine release-inhibitory triterpenes. Chem Pharm Bull 33:1367–74
- Ko HH, Hung CF, Wang JP, Lin CN. (2008). Antiinflammatory triterpenoids and steroids from Ganoderma lucidum and G. tsugae. Phytochemistry 69:234–9
- Koyama K, Imaizumi T, Akiba M, et al. (1997). Antinociceptive components of Ganoderma lucidum. Planta Med 63:224–7
- Keck CM, Müller RH. (2006). Drug nanocrystals of poorly soluble drugs produced by high pressure homogenisation. Eur J Pharm Biopharm 62:3–16
- Kumbhar D, Wavikar P, Vavia P. (2013). Niosomal gel of lornoxicam for topical delivery: in vitro assessment and pharmacodynamic activity. AAPS Pharm Sci Tech 14:1072–82
- Lai F, Pireddu R, Corrias F, et al. (2013). Nanosuspension improves tretinoin photostability and delivery to the skin. Int J Pharm 458:104–9
- Leung SWS. (2002). Lingzhi (Ganoderma) research – the past, present and future perspectives. Ganoderma: genetics, chemistry, pharmacology and therapeutics. In: Proceedings of the International Symposium on Ganoderma Research; 2002; : Medical University Press, 1–9
- Lai F, Sinico C, Ennas G, et al. (2009). Diclofenac nanosuspensions: influence of preparation procedure and crystal form on drug dissolution behaviour. Int J Pharm 373:124–32
- Lademann J, Richter H, Teichmann A, et al. (2007). Nanoparticles – an efficient carrier for drug delivery into the hair follicles. Eur J Pharm Biopharm 66:159–64
- Li WJ, Nie SP, Liu XZ, et al. (2012). Antimicrobial properties, antioxidant activity and cytotoxicity of ethanol-soluble acidic components from Ganoderma atrum. Food Chem Toxicol 50:689–94
- Luo J, Lin ZB. (2002). Advances of pharmacological effects of triterpenes from Ganoderma lucidum. Yao Xue Xue Bao 37:574–8
- Liu P, Rong X, Laru J, et al. (2011). Nanosuspensions of poorly soluble drugs: preparation and development by wet milling. Int J Pharm 411:215–22
- Mohammed D, Hirata K, Hadgraft J, Lane ME. (2013). Influence of skin penetration enhancers on skin barrier function and skin protease activity. Eur J Pharm Sci 51:118–22
- Müller RH, Jacobs C. (2002). Buparvaquone mucoadhesive nanosuspension: preparation, optimisation and long-term stability. Int J Pharm 237:151–61
- Mitri K, Shegokar R, Gohla S, et al. (2011). Lutein nanocrystals as antioxidant formulation for oral and dermal delivery. Int J Pharm 420:141–6
- Patel KK, Kumar P, Thakkar HP. (2012). Formulation of niosomal gel for enhanced transdermal lopinavir delivery and its comparative evaluation with ethosomal gel. AAPS Pharm Sci Tech 13:1502–10
- Patravale VB, Date AA, Kulkarni RM. (2004). Nanosuspensions: a promising drug delivery strategy. J Pharm Pharmacol 56:827–40
- Rehman K, Zulfakar MH. (2014). Recent advances in gel technologies for topical and transdermal drug delivery. Drug Dev Ind Pharm 40:433–40
- Shen BD, Shen CY, Yuan XD, et al. (2013). Development and characterization of an orodispersible film containing drug nanoparticles. Eur J Pharm Biopharm 85:1348–56
- Song JH, Shin SC. (2009). Development of the loratadine gel for enhanced transdermal delivery. Drug Dev Ind Pharm 35:897–903
- Smina TP, Mathew J, Janardhanan KK, Devasagayam TP. (2011). Antioxidant activity and toxicity profile of total triterpenes isolated from Ganoderma lucidum (Fr.) P. Karst occurring in South India. Environ Toxicol Pharmacol 32:438–46
- Venkataraman M, Nagarsenker M. (2013). Silver sulfadiazine nanosystems for burn therapy. AAPS Pharm Sci Tech 14:254–64
- Wang P, Li J. (2010). Construction of rat models with frostbite to varying degrees. J Clin Rehabil Tissue Eng Res 14:8608–11
- Wei Y. (2010). Authentication of Chinese medicines. : Shanghai Scientific and Technical Press, 397–9
- Wu GS, Guo JJ, Bao JL, et al. (2013a). Anti-cancer properties of triterpenoids isolated from Ganoderma lucidum - a review. Expert Opin Investig Drugs 22:981–92
- Wu X, Zeng J, Hu J, et al. (2013b). Hepatoprotective effects of aqueous extract from Lingzhi or Reishi medicinal mushroom Ganoderma lucidum (higher basidiomycetes) on α-amanitin-induced liver injury in mice. Int J Med Mushrooms 15:383–91
- Yue PF, Li Y, Wan J, et al. (2013). Process optimization and evaluation of novel baicalin solid nanocrystals. Int J Nanomedicine 8:2961–73
- Zafren K. (2011). Frostbite: prevention and initial management. High Alt Med Biol 14:9–12
- Zafar S, Ali A, Aqil M, Ahad A. (2010). Transdermal drug delivery of labetalol hydrochloride: feasibility and effect of penetration enhancers. J Pharm Bioall Sci 2:321–4
- Zhang Q, Andoh T, Konno M, et al. (2010). Inhibitory effect of methanol extract of Ganoderma lucidum on acute itch-associated responses in mice. Biol Pharm Bull 33:909–11