Abstract
Objective: This investigation has focused to characterize the elastic liposome containing 5-fluorouracil (5-FU) and to enhance drug permeation across stratum corneum (SC) of the skin (rat) using various surfactants and in vivo dermal toxicity evaluation.
Methodology: 5-FU-loaded elastic liposomes were developed, prepared and characterized for their entrapment efficiency, vesicle size, number of vesicles, morphological characteristics, surface charge and turbidity. In vitro drug release profile, in vitro skin permeation potential and in vitro hemolytic ability of the formulation have been evaluated to compare with drug solution for 24 h. In vitro skin permeation potential was also compared with marketed cream. Furthermore, in vivo skin irritation potential, drug penetration into the skin using confocal laser scanning microscopy (CLSM) and in vivo toxicity studies were performed.
Results and conclusions: The optimized elastic liposomes demonstrated maximum drug entrapment efficiency, optimum vesicular size and considerable elasticity. In vitro skin permeation studies showed the highest drug permeation flux like 77.07 ± 6.34, 89.74 ± 8.5 and 70.90 ± 9.6 µg/cm2/h for EL3-S60, EL3-S80 and EL3-T80, respectively, as compared to drug solution (8.958 ± 6.9 µg/cm2/h) and liposome (36.80 ± 6.4 µg/cm2/h). Drug deposition of optimized elastic liposome EL3-S80 was about three fold higher than drug solution. Skin irritation and CLSM studies suggested that optimized gel was free from skin irritation and capable to deliver 5-FU into the epidermal area for enhanced topical delivery than drug solution. The in vitro study showed minimum hemolysis in the optimized formulation. Finally, in vivo toxicity studies followed with hisptopathological assessment showed that elastic liposome was able to extract SC to improve drug permeation without changing general anatomy of the skin.
Introduction
5-Fluorouracil (5-FU) is a hydrophilic anti-neoplastic agent with plasma half life of 10–20 min (short half life) and high dose that has to be given a dose to attain therapeutic drug level (Peters et al., Citation1993; Holland et al., Citation1997). However, the clinical utility of this drug is challengeable due to serious side effects (Fraile et al., Citation1980). Several serious side-effects are commonly associated with conventional therapy including myelosuppression, hand-foot syndrome and gastrointestinal toxicities. 5-FU has always been administered through parenteral and oral route leading to rapid drug metabolism and erratic drug absorption from the gastro-intestinal tract. Chemically, 5-FU is an antimetabolite with promising antineoplastic activity against several premalignant and malignant conditions of the skin including Bowen’s disease and superficial basal cell carcinomas (Epstein, Citation1985; Bargman & Hochman, Citation2003).
Skin cancer is a deadly disorder with high incidence of cutaneous malignant melanoma and non-melanoma skin cancer that has increased more than 600% worldwide since the 1940s (Osterlind et al., Citation1991). It is a serious health issue of the world that continues to get worse as the incidence increases 5–10% every year. This drug is used in the treatment of several types of solid tumors such as cancer of the gastro intestinal tract, breast, head, neck and pancreas. Some authors reported for the treatment of various skin problems like actinic and solar keratoses (Tutrone et al., Citation2003). Furthermore, several attempts have been made to deliver both hydrophilic drugs (like 5-FU) as well as lipophilic drug in encapsulated liposome carrier. In early report, the preparation of conventional liposome to deliver such hydrophilic drug has been found to have limited entrapment efficiency owing to non-interacting property of 5-FU with lipid bilayer (Tsukada et al., Citation1984). This limited entrapment effect was applicable to smaller liposomes due to the small ratio of trapped to external volume. Furthermore, it was reported that trapping efficiency of 5-FU was found to have only 2–6% depending on lipid composition (Özer, Citation1992; Fresta et al., Citation1993). On the other hand, on prolonged standing of the liposome formulation, variation in vesicular size, phase separation of the lipid bilayer and leakage of trapped drug after removal of un-entrapped drugs are another major physical instability-related issue in developing liposome.
In this investigation, elastic liposome was developed to deliver hydrophilic 5-FU as an alternative vehicle for topical drug delivery to oral conventional dosage form. Several attempts have been made in permeation of the challengeable drug candidate across the stratum corneum (SC). 5-FU drug was the most suitable candidate to deliver across the skin for the management of skin conditions. Ultra-deformability property of the vesicle makes this system a versatile carrier for systemic and topical delivery of the drug. Different non-ionic surfactants in different concentration ratio with lipid had been tried to get enhanced drug permeation and drug deposition into the skin. In vivo toxicity on repeated dose dermal application was evaluated in rat model.
Materials and methods
Materials
5-FU was purchased from Spectrochem Pvt. Ltd. (Mumbai, India). Soya phosphatidylcholine (SPC) was purchased from Sigma (Sigma-Aldrich St. Louis, MO, United States). Chloroform, di-potassium hydrogen phosphate, potassium dihydrogen orthophosphate and sodium hydroxide pellets (AR grade) were procured from S.D. Fine Chemicals Ltd. (Mumbai, India). Rhodamine Red-X (RR) was obtained from Molecular Probe (Eugene, OR). Flonida cream 5% w/w as marketed formulation (marketed product) was purchased from local medical shop. Span-60, Span-80 and Tween-80 were purchased from Himedia (Mumbai, India). HPLC grade methanol and water were procured from Sigma-Aldrich (Mumbai, India). Ethanol and acetone were obtained from Avarice Laboratories Pvt., Ltd. Dialysis membrane with molecular weight cut off value of 12 000–14 000 Dalton was procured from Himedia. In this study, all other reagents were of analytical grade. Double distilled water and Milli-Q water (Millipore, Bedford, MA) were used throughout the experiments.
Methods
Preparation of elastic liposomes
Conventional rotary evaporation sonication method was followed in the preparation the elastic liposome as reported earlier (Cevc et al., Citation1997; El Maghraby et al., Citation2001). A number of batches of elastic liposomes were prepared using different proportions of surfactants (Span-60, Span-80 and Tween-80), phospholipid and potent drug 5-FU. Precisely, a weighed quantity of SPC and non-ionic surfactants were taken in a clean, dry, round-bottom flask (RBF) to dissolve completely in small quantity of methanol:chloroform mixture (1:2; v/v). The solvents were removed using rotary evaporator (60 rpm) under reduced pressure and temperature (40 ± 1 °C) (Perfit Pvt. Ltd., Ambala Cantt., India) and formed a thin film on the inner wall of RBF. Furthermore, traces of solvents were completely removed under vacuum condition. Next, deposited lipid film was hydrated with phosphate buffer solution (PBS, pH 7.4) containing 5-FU and ethanol (7% v/v). Now obtained vesicles were subjected to swell (2 h) at room temperature to get large multi-lamellar vesicles (LMLVs). The elastic liposome dispersions were prepared in triplicate. In order to reduce the size of LMLVs, LMLVs were sonicated at 4 °C temperature and 40 watt for 15 min using ultra probe sonicator [Probe Ultrasonicator, Imeco Ultrasonics, Sonics and Materials. Inc. Newtown, CT]. The optimized formulation was lyophilized (instrument) in presence of cryoprotectant sucrose (4 g/g of lipid) (Nounuo et al., Citation2005). The final formulation contains 5% w/v drug in vesicular formulation.
Preparation of liposomal formulation
Conventional liposome (control) was prepared by the cast film method (Mishra et al., Citation2007). In this procedure, lipophilic surfactants (especially Span-60, Span-80 and Tween-80) have been replaced with cholesterol (CH) keeping constant molar ratio of PC:CH (7:3) and dissolved with least quantity of chloroform: methanol mixture (2:1; v/v) in a RBF. A very thin lipidic film was developed by evaporating solvents under reduced pressure and temperature described earlier using rotary evaporator. Now, still remained trace quantity of solvents were removed using vacuum method. Thus obtained film was hydrated with PBS (pH 7.4) containing 2.5 mg/ml 5-FU at high temperature (50–55 °C) followed with continued vortex of the flask for an hour in addition to get multi-lamellar vesicles. MLVs were further transformed into unilamellar vesicles using probe sonicator for 5 min.
Incorporation of optimized elastic liposome in carbopol-980 gel (1% w/v)
Finally, elastic liposome EL3-S80 was screened as an optimized formulation based on vesicular size and size distribution, elasticity, percentage entrapment efficiency (% EE) of 5-FU and selected to incorporate in carbopol-980 gel (1% w/v). EL3-S80 was incorporated into previous prepared blank carbopol-980 (5 g) to obtain homogeneous gel. In this method, a calculated amount of carbopol-980 polymer was completely dispersed in distilled water and homogenized. Then after, it was left overnight after mixing with 2–3 drops of basic triethanolamine (Sigma-Aldrich) as cross linking agent on constant slow stirring, and the final pH was adjusted to pH 7.4. The entrapped air while homogenization was removed by keeping the gel overnight at room temperature (Hussain et al., Citation2014). Moreover, it enables complete cross linking of the acidic carbopol polymer with the basic triethanolamine. The final strength of the drug in 0.5% w/v gel was found to be 5% w/w.
Analysis
In order to estimate drug concentration in different formulation, percentage entrapment and in vitro drug permeation study, 5-FU content was determined using high-performance liquid chromatography (HPLC) method. The content of drug was determined with liquid chromatograph model-1120 Compact LC (Agilent Technologies, Santa Clara, CA) and an ultraviolet detector. Separation was obtained using reverse phase column Shiseido C-18 (250 × 4.6 mm, 5 µm internal diameter). 5-FU was detected at 266 nm with mobile phase of water and methanol (95:5, v/v) at a flow rate of 1.3 ml/min. Mobile phase was prepared freshly, filtered through membrane filter and degassed by bath sonication. The experiment was performed at room temperature (25 °C).
Physico-chemical characterization of elastic liposome and its gel
Morphological analysis of vesicles
Initially, developed elastic liposome formulations were diluted with 0.9% saline solution, and they were counted per mm3 using optical microscope with hemocytometer. Morphology of liposomes was studied under optical microscope (Leica, DMIRE2, Wetzlar, Germany) and electron microscope. Scanning electron microscopy (SEM) of optimized lyophilized elastic liposome and transmission electron microscopy (TEM) of optimized elastic liposome were carried out to examine the morphological characteristics. For morphology study under SEM, samples were coated with gold under vacuum using gold coater and visualized (JSM – 5310LV Scanning Microscope, Tokyo, Japan). Moreover, elastic liposomal vesicles were visualized using TEM (Hitachi H-500 TEM) at voltage of 100 kV. For this analysis, a single drop of the sample was kept on carbon-coated copper grid to get a thin film. It was negatively stained with a drop staining reagent (1% w/v phosphotungstic acid) before the film to be dried on the grid. Excess of the solution was drained off with a filter paper, and the grid was allowed to air dry thoroughly to view on a transmission electron microscope (Guo et al., Citation2000). The zeta potential (mV) of elastic liposome formulations was determined using Zetasizer (Malvern Instrument Ltd., Worcestershire, United Kingdom) at 25 ± 1 °C temperature. The samples were analyzed using an aqueous dip cell in an automatic mode by diluting with Milli-Q water.
Drug entrapment efficiency (% EE)
Elastic liposome is an ultra-deformable bilayer vesicular system that allows the hydrophilic drug to be entrapped inside the aqueous compartment and lipophilic drug into the outer lipid bilayer. Therefore, it was utmost need to calculate the entrapped drug into the vesicle. The %EE was determined using method reported earlier (Bendas & Tadros, Citation2007). Briefly, the elastic liposome formulations were kept overnight at 4 °C temperature and then subjected to ultracentrifugation for 2 h at 15 000 rpm. The supernatant was obtained, and 5-FU content was determined using HPLC method at λmax 266. Thus, the loaded or trapped drug in the vesicles was calculated. The percentage drug entrapped in the vesicles was then calculated using the following equation.
(1)
where Qt was total theoretical amount of the drug added, whereas Qs was the amount of 5-FU found in the supernatant.
Assessment of turbidity and elasticity
Turbidity measurement was performed as per method reported by Jain et al. (Citation2008). To measure turbidity of the several developed elastic liposome, PBS (pH 7.4) was used as blank reference standard. Next, turbidity was determined against PBS using Nephlometer (Superfit, Mumbai, India).
Elastic nature of the vesicles of elastic liposomes was measured by extruding through the polycarbonate filter membrane (Millipore) with pore diameter of 60–200 nm holder (stainless steel) of 25 mm diameter filters of 200 ml capacity barrel at 2.5 bars. The amount/volume of vesicle suspension, which was extruded during 10 min, were measured for vesicle shape and size before and after the filtration. The elasticity of vesicle membrane was calculated by using the following formula:
(2)
where E = elasticity of vesicle membrane; J = amount of suspension, which was extrude during 10 min; rv = vesicles size after extrusion; and rp = pore size of the barrier.
Viscosity measurement
The viscosity and rheology of the optimized elastic liposome gel were determined using R/S CPS Plus Rheometer (Brookfield Engineering Laboratories, Middleboro, MA) using spindle # C 50-1 at 25 ± 1 °C. Sample of gel formulations were analyzed at different rotational speed (0.5, 1, 2, 3, 4, 5, 10, 20, 30, 50, 60, 70, 80 and 100 rpm) of the spindle. Gel (1 g) was used for the assessment of viscosity for 50 min of the operation time. Applied shear rate was 410 min−1, and the diameter of the spindle was 50 mm.
Spreadability measurement
Rheological and flow properties measurement are needed to characterize topical formulation for determination of its rheological behavior. Spreading properties was measured by the method reported earlier (Hussain et al., Citation2014). For the assessment of spreading properties of the elastic liposome formulation formulation, a known weighed amount of cellulose acetate filter paper (W1) was kept at the middle of the aluminum foil sheet. The prepared and developed formulations were taken into the 5-ml glass syringe. Now, a constant number of the elastic liposome suspensions to be tested (around 20 drops) were injected out of the syringe on the specific surface area at the center of the filter paper. The filter paper portion saturated with the formulation after 10 min was removed away and weighed again. Now, the unsaturated portion was accurately weighed (W2), and percentage spread by weight was reported using the following equation:
(3)
In vitro drug release assessment
In vitro drug release study of elastic liposomal formulations, drug solution and marketed cream was carried out using dialysis membrane (molecular cut-off 14 000 Da, Himedia Labs) under sink condition maintained throughout the studies by adding 2% v/v DMSO in receptor chamber that facilitates the diffusion rate. A weighed amount of drug (5% w/v) from each vesicular formulation was loaded on the dialysis membrane and suspended in a beaker containing phosphate buffer media (100 ml, pH 7.4) at 32 ± 1 °C with constant stirring (100 rpm) for 12 h. Control drug solution was free 5-FU aqueous solution (5 mg/ml). Gel formulation (5% w/w of gel) was loaded in dialysis tube. Samples (1 ml) were withdrawn at 0.5-, 1-, 2-, 4-, 6-, 8-, 10- and 12-h time intervals, replaced with an equal quantity of fresh same buffer solution and analyzed for the drug released using UV-Vis Spectrophotometer (Shimadzu, U-1800, Tokyo, Japan).
In vitro skin permeation and deposition studies
In vitro drug permeation of elastic liposome loaded with 5-FU was determined using Franz diffusion cell as per method reported (Jain et al., Citation2005). The rat was ethically sacrificed, and excised skin was washed with isotonic saline solution and made free from hair using shave blade. The fatty debris present to the dermis portion was removed using isopropyl alcohol. The abdominal rat skin was mounted between the donor and receptor compartment of diffusion cell (3.14 cm2) in such a way that epidermal portion faces the donor side. The receptor chamber was filled (22.5 ml) with buffer solution (PBS; pH 7.4). Formulations loaded with 5-FU were placed onto the donor chamber on the skin. The permeation study was performed at temperature of the cell assembly maintained at 37 ± 1 °C throughout the study, and the medium of the receptor compartment was stirred using a magnetic stirrer. One mlliliter sample was withdrawn from receptor compartment at 0.5-, 1-, 2-, 4-, 6-, 8-, 10-, 12-, 16-, 20- and 24-h time intervals and immediately replaced with equal volumes of fresh media. The drug permeated across the skin was evaluated by HPLC method at 266 nm. Flux and extraction ratio were calculated for different formulations and compared with drug solution and marketed cream.
The in vitro skin deposition of 5-FU from different elastic liposomal, drug solution and marketed cream were studied using Franz diffusion cell. Similar procedure used for skin permeation studies was followed for measurement of drug deposited into the skin. The mounted skin after 24 h of the permeation studies was removed carefully. The remaining formulation adhering to the skin was removed by washing five times with 50% v/v ethanol. The washed skin was cut into small pieces and homogenized with hydro-alcoholic solution (50% v/v ethanol; 10 ml) and left for 24 h. Amount of 5-FU drug was determined from the supernatant using HPLC after centrifugation for 5 min at 3000 rpm.
Hemolysis study
Preparation of samples
To carry out the hemolysis study, elastic liposome formulations in different dilution and drug solution in PBS (pH 7.4) were prepared before commencement of the experiment in varied concentrations. 5-FU drug was completely dissolved in PBS in different concentration ranging from 0.125 to 2 mg/ml, and fresh formulations were diluted in same concentration ranges. All formulations were stored in cool temperature before study.
Preparation of erythrocyte suspension
Blood samples were taken from human healthy volunteer. Blood was collected in sample vials containing EDTA, which prevents the blood from clotting. Blood sample of 5 ml was collected and centrifuged to separate red blood cells (RBCs) from serum at 2400 rpm for 5 min at room temperature. This fresh erythrocyte cells was washed with isotonic PBS and again centrifuged at 2400 rpm for 5 min at same temperature. The same washing and centrifugation were repeated three times to get intact RBCs cells. Supernatant was taken away, and the RBCs cells were diluted to a hematocrit of 4% v/v in PBS. All the samples were stored at 0 °C and 4 °C to prevent from auto-hemolysis.
Hemolysis measurement
Hemolysis was assessed by previously described method (Hussain et al., Citation2014). Each sample (0.5 ml) was diluted with PBS (0.5 ml). For control, 0.5 ml of RBC suspension was mixed with 0.5 ml of PBS (as negative hemolysis control). Distilled water was used as positive hemolysis control. 5-FU elastic liposomal formulation at different concentrations was interacted with RBC suspension. All formulations and controls were incubated at 37 ± 1 °C with constant agitation for 24 h. Each sample was then centrifuged at speed of 4000 rpm for 10 min to separate out of RBCs and debris. The obtained supernatant (200 µl) was removed and diluted with 2 ml of distilled water. The content of hemoglobin released was determined by taking absorbance at 540 nm (λmax) using a UV-Vis spectrophotometer. One-hundred percent hemolysis was defined as the maximum amount of hemolysis obtained from distilled water. All experiments were performed in triplicate, and the mean values were reported.
In vivo animal studies
All the animal studies were performed by using mice weighing 25–30 g of either sex, rats weighing around 180–250 g and rabbits (1.8–2.0 kg) for Draize study. Rabbits were used for irritation study and had not been sacrificed. They were properly marked and kept in plastic cages in an air-conditioned room. Food pellets and water were provided ad libitum. Experiments were carried out after approval of the Institutional Animal Ethics Committee of the Faculty of Pharmaceutical Sciences, PCTE Group of Institutes and in accordance with the disciplinary principles and guidelines of CPCSEA, Punjab, India.
Skin irritation potential
The irritancy of different formulations was determined in male Albino rabbits (1.8–2.0 kg) based on the method described by Draize et al. (Citation1944). The hairs on the back with marked area were trimmed just 24 h before the commencement of experiment. Three squares were developed on backside of each animal. The animals were randomly grouped into nine groups. Each rabbit had three different marked areas for application. The first, second, third and fourth group received sham control, PBS, drug solution and SLS (20% w/v) (as positive control), respectively, for topical application. Next, group fifth, sixth and seventh received EL3-S60, EL3-S80 and EL3-T80 elastic liposome formulations, respectively. Finally, eighth and ninth group received marketed and liposome formulation, respectively. The treated area was protected from wash out of treatment applied using nylon mesh kept above the treated area. At different time intervals 0, 4 and 72 h after application, and exposed area was scored for the erythema and edema on grade of 0–4 as described by US-FDA guidelines. The mean erythema and edema scores were calculated after inspection based on their degree of severity caused by application of formulations: no erythema/edema = 0, slight erythema/edema = 1, moderate erythema/edema = 2 and severe erythema/edema = 3.
Confocal laser scanning microscopy study
For further investigation of permeation potential of elastic liposome through the different layer of skin, penetration study was performed using confocal laser scanning microscopy (CLSM) (Khurana et al., Citation2013). To visualize the formulations, formulation EL3-S80 was prepared loaded with fluorescent probe RR instead of 5-FU and served as control EL3-S80-RR. Second was considered as treated with drug loaded EL3-S80-RR. Previously prepared rat skin sample free from hairs and any damage was mounted on the donor and receptor compartment of Franz diffusion cell filled with mixture of PBS (pH 7.4) and methanol (30% v/v) solution to achieve sink condition in receptor compartment. Excess skin portion was trimmed off. Sample (each gram equivalent to 0.5% w/v probe RR of elastic liposome) of formulations was kept at the dorsal site of marked area (1 cm2) on the skin in donor compartment and protected from any contamination. Control skin was treated with probe (0.5% w/v) only. Next the ex vivo skin permeation study was conducted as per method described above in permeation study. Experiment was carried out under the same experimental condition of temperature (32 ± 1 °C). Rat skin was removed after 24 h and sectioned to evaluate for the depth of penetration of probe into the skin (Dubey et al., Citation2007). The formulations and dye solution adhered on the surface were washed with distilled water three times to drain off completely. To image the full thickness of the skin, it was scanned at different increment of through the z-axis of a CLSM (LSM 510 with an attached universal Zeiss epifluoroscence microscope). In this study, optical excitation was conducted with a 488 nm Argon Laser Beam and fluorescence emission at 560 nm.
In vivo toxicities evaluation
Optimized formulation EL3-S80 gel loaded with drug 5-FU was evaluated for any in vivo toxicities in mice after topical application. In total, 18 mice were randomly divided into three groups, each containing six mice. Mice were healthy and weighed about 25–30 g before commencement of experiment. The dorsal skin of the mice was shaved properly to remove hair and made an application site for gel formulation. Great care was kept while trimming and shaving the skin for any surgical lesion. First group was untreated and served as control group. Group second was treated with blank formulation. Finally, third group was treated with EL3-S80 gel loaded with 5-FU. Now, blank gel and EL3-S80 drug-loaded gel was applied with the dose of 100 mg gel (equivalent to 0.2 mg drug in formulation) for group second and third, respectively. The remaining portion of the gel after drug release (24 h) was washed away with adsorbent cotton dipped in physiological solution (0.9% w/v saline) from the treated skin surface of mice. The applied dorsal site of the skin was regularly inspected for any types of changes occurred during 24 h of experiment. In this study, mice were observed frequently and recorded for variations in eyes, mucous membranes, somatomotor activity, circulatory, respiratory, autonomic and their behavioral changes. The erythema or irritation at the site of application was mainly observed. After 24 h of experiment, animals were ethically sacrificed by cervical dislocation. Thereafter, major visceral organs like skin, liver, kidney and heart were taken out. Then, isolated organs and skin were preserved in 10% formalin solution for histopathological examination. By the histopathological study, the gross anatomical changes in the major organs were observed and compared with normal anatomy. The major organs were isolated and preserved in 10% formalin solution for histopathological study as per procedure reported (Chen-Yu et al., Citation2012). Skin, liver, kidney and heart were studied after dissection. Each individual organ’s specimen was stained with hematoxylin and eosin.
Statistical analysis
Data were presented in terms of the mean and standard deviation. The mean and statistical analysis was carried out employing analysis of variance. In calculation of differences, statistically significant values were considered at p < 0.05. All the parameters were calculated by applying some software like GrapPad software (GraphPad prism, version 5.01, Inc, La Jolla, CA) and Origin 6.1, v6, 1052 (B232) (Origin Lab Corporation, Northampton, MA). All the experiments were performed in the triplicate manner.
Results and discussion
Preparation of elastic liposomes, conventional liposome and gel formulation
Several elastic liposome formulations of 5-FU were prepared by varying the concentration and type of surfactants. Both hydrophilic and lipophilic surfactants were used to develop formulations as listed in . The effect of type and concentration of surfactants were evaluated on vesicle size, elasticity of the vesicles, % EE and permeation flux and drug deposition. The surfactants like Tween 80, Span 60 and Span 80 used in concentration range of 5–30% w/w of lipid (SPC) have shown significant effect on entrapment efficiency of 5-FU. In spite of being better than liposome as drug delivery system, physical stability issue must be taken into consideration in term of vesicle size, drug leakage and phase separation of bilayer on long-term storage. Hence this problem was rectified through lyophilization process that improved shelf life of the finished optimized product (data not given). Some authors also reported that the stability property of liposome-based dispersion for topical application could be highly superior through their incorporation in appropriate in hydrophilic polymer (Gabrijelcic & Sentjurc, Citation1995). Therefore, the final product was lyophilized and incorporated into the hydrophilic carbopol gel described later in this study.
Table 1. Composition of vesicular formulation and their characterizations.
From the , it was observed that Span-80 containing formulation had more affect on % EE, average vesicle size and elasticity with some exception (like EL1-S60, EL2-S60 and EL3-S80). This might probably be due to hydrophilic Tween 80 was not able to interact with lipophilic lipid to form stable bilayer. Formulations were prepared varying lipid:surfactant ratio as listed in the . The optimum lipid to surfactant was 7:3 (v/v) to get high % EE of 5-FU, elasticity and optimum vesicle size in both type of surfactants used. On the other hand, liposome composed of SPC and CH (instead of Span-60 and Span-80) showed least drug entrapment (43.1 ± 1.76%) and elasticity (12.7). Lower value of elasticity in liposome could be obtained with the use of CH. CH forms a stable bilayer and is a cause of least flexibility or deformability in liposome. It was reported that 5-FU was not shown to be adsorbed to dipalmitoylphosphatidylcholine/PC bilayer (Fresta et al., Citation1993). In contrast, it was observed between lipid and CH.
Carbopol 980 gel (1%w/v) was prepared and used as carrier for topical application of the 5-FU loaded with optimized elastic liposome (EL3-S80). Carbopol-980 aqueous dispersion is acidic in nature and neutralized by adding basic triethanolamine to get consistent and viscous transparent gel. Presence of surfactant in carbopol-980 gel changes the intrinsic viscosity and flow behavior of resulting gel after incorporation. Some authors reported that an increase in non-ionic surfactant concentration gradually decreases the viscosity and elasticity of the gel, they are obtained due to enough surfactant molecules surrounding each carbopol-980 molecule to form intrapolymeric micelle. However, non-ionic surfactant like Tween-80 and Span-80 were less interactive with carbopol-934 NF than ionic surfactants like sodium dodecyl sulfate as well as high molecular weight pluronic F-127 (Barreiro-Iglesias et al., Citation2001). Thus, Span-80 present in the elastic liposome was not in such quantity to decrease the viscosity of gel too much. Final strength of gel obtained after incorporation of EL3-S80 into 1% w/v gel was 5% w/w of the gel (0.5% w/w). The obtained gel was completely free from grittiness, translucent and with optimum thixotropic flow performance. The gel enriched with surfactant (Span-80) that helps to penetrate into the skin and delivers elastic liposome loaded with drug into the epidermal area across SC. The presence of ethanol (7% v/v) in elastic liposome additionally promotes squeezing of the carrier through the barrier SC.
Characterization of elastic liposome and gel loaded with optimized formulation
Number of vesicles of the formulation per cubic mm and turbidity has been investigated as shown in the . There was no significant difference in turbidity between formulations EL4-S80 and EL5-S80. Significant difference was observed in the optimized formulation EL3-S80. It was observed that on increasing the concentration of surfactant relatively to lipid, turbidity increases in all three surfactants. Vesicular size and size distribution, zeta potential, % EE, elasticity measurement, viscosity, % spreading value and vesicle shape were depicted in the . In the , it was revealed that the entrapment efficiency of the 5-FU increases with increase in Span-60 and Span-80 concentration with respect to lipid amount. But, there was no any such trend in Tween-80. This might be due to coexistence of mixed micelles and vesicles at higher concentration of surfactant, with the consequence of lower drug entrapment in mixed micelles. The formation of micelle structure at higher concentration of surfactant is an established fact. This fact was proved by Lasch et al. (Citation1991) and Lopez et al. (Citation1998). Elastic liposomes composed of different types of surfactants showed varied entrapment efficiency (% EE). In the , selected formulations were displayed with their vesicular size, elasticity and % EE for comparison among them. EL3-S60, EL3-S80 and EL3-T80 were found to have with maximum % EE 82.2 ± 1.8, 88.7 ± 2.9 and 78.4 ± 1.2, respectively, among other formulations. Maximum figure was observed in EL3-S80 followed with EL3-S60 and EL3-T80. This maximum loading could be explained of hydrophilic drug in the aqueous compartment of the vesicular elastic liposome. This might be probably facilitated by the partitioning of hydrophilic drug toward the aqueous phase. Theoretically, hydrophilic–hydrophobic balance (HLB) value of Span-60, Span-80 and Tween-80 is 4.7, 4.3 and 16.7, respectively. Thus interaction of these surfactants with lipid decreases with increase in HLB value leading to stable formation of lipid bilayer with SPC and Span-80 in the ratio of 7:3. All these three formulations were found to have close % EE value owing to same lipid to surfactant ratio (7:3). Furthermore, revealed a comparative characterization of best three elastic liposomes (EL3-S60, EL3-S80 and EL3-T80) and optimized gel formulation (EL3-S80 gel) as compared to blank carbopol-980 gel. Water solubility of 5-FU (pKa value 8 and 13) can greatly be increased on increasing pH value corresponding to maximum drug solubility in buffer for improved entrapment in elastic liposome (Barreiro-Iglesias et al., Citation2001).
Table 2. Characterization of selected formulations and gel (EL3-S80 gel).
Optical microscopy of the optimized elastic liposome was used to visualize vesicular distribution as shown in the (without sonication). The images of the vesicles were somewhat appearing vesicular. However, the SEM () and TEM analysis () were performed to evaluate morphology (shape) and lamellarity of the vesicle of optimized EL3-S80, respectively. The elastic liposomes were found to have unilamellar and spherical in shape () after sonication. In the , vesicle size and size distribution (204 ± 14.8 nm) and zeta potential (−11.2 ± 0.2 mV) of optimized EL3-S80 was shown. After incorporation into the gel, the mean vesicle size was decreased to 156.5 ± 11.5 nm possibly due to diffusion of water from elastic liposome out of the inner compartment. The size was calculated using differential light scattering method, which was in accordance with the results obtained from TEM. Furthermore, there was no significant difference (p > 0.05) in % entrapment efficiency after incorporation () into the carbopol gel. The pH value was maintained at 7.4 in both elastic liposome suspension and gel formulation.
Figure 1. Leica optical microscopy of formulation (400×). (a) Blank elastic liposome and (b) optimized 5-FU loaded elastic liposome (without sonication).
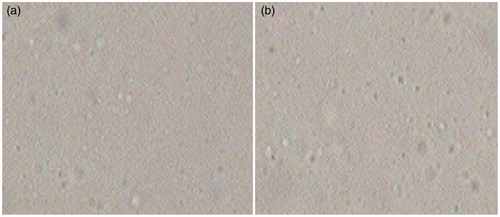
Figure 4. SEM photograph showing globular vesicle of lyophilized elastic liposome EL3-S80 (a) and transmission electron micrograph (TEM) of optimized elastic liposome formulation. Magnification: ×15 000.
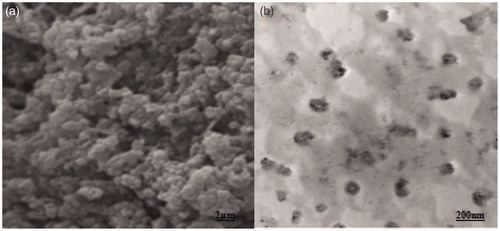
Deformability is the unique property of the elastic liposome. Sensitivity of this carrier due to uniqueness to water gradient across the skin makes elastic liposome a novel and potent system for topical drug delivery. The highly crystalline SC barrier of the skin is allied with sparingly distributed pores. Formulations are permeated through these pores significantly and act as permeability shunt. Moreover, these pores locally lower the skin permeability barrier. It is noteworthy that these pores act as major sites for ultradeformable bodies to be squeezed across the skin under the effect of transepidermal water gradient as driving force. The flexibility of the vesicle membrane and stress-dependent adaptability are the two potential feature of the elastic liposome as compared to conventional liposome and other types of the drug-loaded lipid suspensions. In was studied that very low drug leakage was observed when the formulations were stored at 4 °C as compared with formulations stored at 25 ± 1 °C after 120 d storage. This finding suggested that the refrigerated conditions are well-suited for the developed formulation. Therefore, in this study, optimized elastic liposome was lyophilized to prevent drug leakage and enhance shelf life of the formulation. Elastic liposome has an ultra-deformable behavior to get squeezed out (due to fluidity property) through smaller pore of the skin. Therefore, elastic property was determined, and maximum value was obtained in EL3-S80 (47.7) as listed in . The elasticity was somewhat improved by addition of ethanol (7% v/v) into the formulation. The effect of surfactants on fluidity of the vesicular elastic liposome can be explained, which would be considered as a major factor for squeezing through pores of the skin. Chemically, Span-60, Span-80 and Tween-80 are sorbitan monostearate, sorbitan monooleate and polyoxyethylene sorbitan monooleate, respectively. The physical property of phospholipid membrane could be studies using electron spin resonance (Knowles & Marsh, Citation1991). Span-80 with unsaturated sorbitan ester containing elastic liposome possessed a much higher elasticity (fluidity) than Span-60-based elastic liposome. It was reported that the region near the head group of Span-60 niosome seems to be slightly more ordered than Span-80 niosome. However, the bent structure due to unsaturation in the oleate chain of Span-80, compared to Span-60, causes drastic changes in the chain packing leading to higher fluidity in the vesicular lipid membrane. CH addition in the liposome formulation causes restricted fluidity and subsequently found to have minimum flux across the SC of the skin. Furthermore, this oleate-induced fluidity can also be observed in Tween-80-based elastic liposome. Both fatty ester (Span-80 and Tween-80) have common unsaturated hydrocarbon chain, but Tween-80 has a much bigger headgroup than Span-80, which is responsible to interfere with tight packing of the surfactant molecules. Therefore, it is noteworthy that the bigger headgroup of Tween-80 and the presence of double bond in oleate fatty acid had increased the fluidity of elastic liposome than Span-60 (Yeom, Citation2014).
Spreadability study
Spreading property of the topical gel is an important parameter for proper flow and smooth spreading ability at the site of application. After assessment of spreading ability of elastic liposomes, the optimized formulations EL3-S60, EL3-S80 and EL3-T80 exhibited maximum percentage of spread by weight 112.45 ± 10.2, 101 ± 8.9 and 138 ± 13.5, respectively. This indicated excellent spreadability behavior on the skin as listed in the . Observed differences were significant (p < 0.05) between the spreadability of elastic liposome formulations and EL3-S80 gel. There were almost two reductions in spreading value in gel formulations than optimized elastic liposome.
In vitro drug release study
In vitro drug release from the elastic liposome and elastic liposome gel formulations was absolutely significant (p < 0.05) as compared to free drug solution (0.01% w/w). The elastic liposome gel (EL3-S80 gel) provided extended drug release rate over period of 24 h than drug solution (). The drug release showed that 7.85 ± 1.1% w/w drug of the formulation from the EL3-S80 gel, whereas 12.3 ± 0.12% w/w and 89.58 ± 2.5% w/w drug were released from the elastic liposome (EL3-S80) and 5-FU solution, respectively, within initial first hour. This revealed that rapid release of the drug from drug solution indicated absence any interaction of the drug with dialysis membrane in given set of experimental condition. Increased release rate of 5-FU from the elastic liposome could be attributed due to larger surface area and permitting drug release rate. Although the drug release rate from the EL3-S80 gel than elastic liposome (pH 7.4) was slow as statistically significant (p < 0.05). Possible reason for this significantly different release rate of EL3-S80 gel is its higher value of viscosity as well as its smaller vesicular size. Thus, elastic liposome (32.4% w/w) and EL3-S80 gel (18.81% w/w) formulation had shown 2.7- and 4.67-fold slower drug release, respectively, as compared to drug solution (87.97%) in first 4 h suggesting controlled and extended release profile. Drug release profile as treated in zero order, first order, Higuchi and Korsmeyer–Peppas mathematical models to evaluate the release pattern from carrier systems and the drug release mechanism. The correlation coefficient value of Korsmeyer–Peppas model was greater than 0.94 in EL3-S80 gel and elastic liposome except drug solution. The drug release pattern from EL3-S80 gel and elastic liposome showed zero-order release kinetics with a best fit r2 value 0.9985 and 0.9898, respectively, than drug solution following first-order release kinetics (r2 = 0.9997) in initial 4 h.
In vitro skin permeation and drug deposition study
The permeation studies of all developed formulations of 5-FU across excised rat abdominal skin were conducted using Franz-diffusion cell, and results were summarized in the . The maximum drug permeation for different elastic liposomal formulations were 55.75%, 64.92%, 51.29%, 26.62%, 6.48% and 36.64% for EL-S60, EL-S80, EL-T80, liposomes, plain drug and 5-FU marketed cream, respectively. The maximum drug permeation was revealed by EL3-S80 formulation (64.92%) than the others. Thus, maximum percentage cumulative amount of drug permeated across the skin using EL3-S80 was about 10.01-fold higher than that obtained from drug solution as shown in the . The elastic liposomes (EL3-S80) showed maximum permeation, whereas liposomes and free drug solution showed relatively low skin permeation. This might be because of rigid liposome containing CH in its composition and hydrophilic nature of 5-FU does not allow its permeation across the highly crystalline barrier (SC) of the skin. Furthermore, EL3-S60, EL3-S80, EL3-T80 and EL3-S80 gel have shown steady state flux 77.07 ± 6.34, 89.74 ± 8.5, 70.9 ± 9.6 and 78.89 ± 2.8 µg/cm2/h, respectively. For comparison, the enhancement ratio (ER1) and ER2 of EL3-S80 was 10.01 and 1.77, respectively, which was found to be higher value than EL3-T80 (ER1 = 7.91 and ER2 = 1.4). The maximum flux and enhancement ratio of the EL3-S80 was in accordance with the highest drug entrapment efficiency, responsible for maximum therapeutic effectiveness of the drug in disease management. Moreover, in vitro permeation profile of the optimized formulations (EL3-S80) was found to be slow release (nearly 65% in 24 h) and acts as local drug depot as shown in the . Slow and extended permeation rate of 5-FU may be attributed owing to limited diffusion of hydrophilic 5-FU across lipophilic bilayer of vesicle as well as SC. The rationale of enhanced flux and enhancement ratio in Span-80-based elastic liposome was its chemical structure and HLB value controlling the size and fluidity of vesicular phospholipid membrane. In general, the size of vesicle increases with decrease in HLB value of surfactant. In addition, incorporation of the CH in the vesicle decreases the fluidity and elasticity of the vesicles. As discussed earlier, the unsaturation or the presence of double bond in long chain hydrocarbon of oleate ester led to disturbance in packing of chain of surfactant (Yeom, Citation2014). Increased fluidity in membrane of vesicle increases the elasticity and squeezing capability of the drug-loaded formulation into the dermal area across the SC. Span-80 showed profound fluidity near the bilayer center of phospholipid membrane than the region of headgroups.
Figure 6. Percentage cumulative amount of 5-FU permeated through albino rat skin over a period of 24 h.
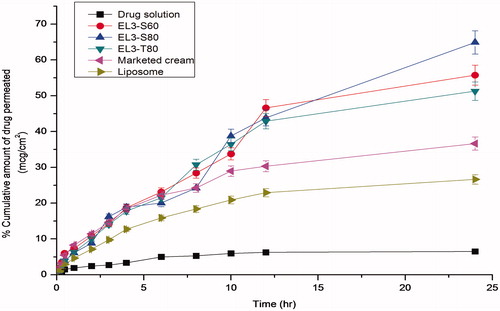
Table 3. In vitro permeation parameters of different 5-FU formulations across the albino rat skin after 24 h.
The elastic characteristics of vesicle membrane and permeation enhancement effects of elastic liposomes support their better skin permeation potential due to their elastic nature (Cevc et al., Citation1997; Jain et al., Citation2006). Effective drug deposition studies were performed with the objective of determining the drug depot owing to elastic liposomes in the deeper layers of skin. In this study, we hypothesized that 5-FU-loaded elastic liposome could lead to significant deposition into the skin. Dosage form should have to be accumulated in the deeper layer of the skin to cure the skin-related actinitic, skin cancer and solar keratoses. depicted comparative percentage drug deposition into the skin after topical application of elastic liposome formulation, liposome and free drug solution. Drug deposited in the skin was found to be 265 ± 11.4, 201 ± 15.9, 176 ± 9.0 and 89 ± 3.7 µg for EL3-S80, EL3-S60, EL3-T80 and drug solution, respectively, after 24 h of topical administration of these formulations. Thus, drug deposited in EL3-S80 was about three fold higher than drug solution and two fold greater than liposome formulation (132 ± 8.2 µg), respectively. The observed better skin accumulation of elastic liposomes likely to be attributed due to the difference in the mechanism of drug transport across the skin from elastic liposomes and drug solution (Jain et al., Citation2006; Garg et al., Citation2008). However, after reaching to the upper site of dermal layer and lower site of epidermis that is its site of action in various skin diseases like cutaneous (skin) cancer, actinitic and solar keratoses, it is available in sufficient concentration for effective therapeutic response. Thus, topical application could be suggested as an effective therapeutic efficacy and reduced systemic side effects.
Hemolysis measurement
Results of hemolytic toxicity assay of elastic liposomes and 5-FU drug solution formulation after 12 h of incubation at concentration of 2, 0.5 and 0.125 mg/ml are reported in . The results showed that all three tested formulations were found to be in concentration-dependent hemolysis over period of 24 h. The elastic liposome formulations showed 35% hemolysis at higher concentration (2.0 mg/ml). Higher hemolysis was observed with higher concentration of formulation, which might be due to presence of surfactant and ethanol. The lesser hemolytic activity of elastic liposome formulation is due to their biocompatible lipid composition applied topically. These vesicles are made from biocompatible phospholipids as major constituent with little quantity of surfactant. After incubation with human erythrocytes, distilled water (as positive control) led to 100% hemolysis over the period of 24 h. In case of negative control, normal saline caused no significant (p > 0.05) hemolysis. Moreover, hemoglobin released after 24 h incubation with the erythrocytes was found to be insignificant as compared to normal saline solution group suggesting elastic liposome are unable to interact with human RBCs at physiological level.
Animal studies
Skin irritation potential studies
The skin irritation potential was determined based on the Draize method. SLS was used as positive control as listed by European Union as irritant chemicals, and PBS-treated group (pH 7.4) served as negative control. The test is based on visual scoring of changes, such as erythema and edema, observed following topical application. The irritation potential of topical formulations was evaluated, and results have been shown (). Its utility and acceptability by the patients have been limited when any irritation or erythema is observed on topical application. Hence, any topical delivery system of elastic liposomal should be free of these erythematic reactions. In this study, the results showed that no severe irritation symptoms such as erythema (redness) and edema (swelling) during 72 h except reference positive control group (SLS). After application, all the scores were zero for placebo/plain gel (sham control), drug solution (group III) and elastic liposomal formulations (group V, VI and VII; ). The reference SLS aqueous solution triggered itching and redness at the applied area, resulting in visible skin irritation and inflammation with group III, calculated to be 1 score. Hence, skin irritation study revealed that neither polymeric plain gel alone nor elastic liposomal formulation (5-FU elastic liposome) exhibited any noticeable irritation or inflammation on or around the application site. Thus, the developed formulation could be considered as a non-irritant and safe on topical application.
Table 4. Mean erythemal and edema scores observed at the end of 1, 24, 48 and 72 h.
Confocal laser scanning microscopy
CLSM study was used to justify the permeation potential and drug deposition of the 5-FU-loaded formulation into the skin after topical application over period of 24 h as shown in . It was clearly revealed that the distribution of dye in both control and treated drug-loaded EL3-S80-RR are same in term of florescence intensity indicating equal extent of penetration of dye probe-loaded elastic liposome and drug cum dye probe-loaded elastic liposome. After 24 h of incubation on Franz diffusion cell, the penetrated drug-loaded elastic liposome could be observed to the upper dermal layer of the skin in the (arrow indicating elastic liposome deposited). Moreover, it was found that there was time-dependent penetration in the different strata of the skin. In initial first few hrs, dye distribution was more confined to the SC and upper epidermis. In contrast to this finding, dye probe distribution was dramatically increased to upper layer of dermis in all formulations (data not shown) after 24 h. This might be due to elastic property of the elastic liposome, lipophilic dye probe and possibly subsequent fusion with the membrane lipids of the skin. Similar results were observed in podophyllotoxin-loaded solid lipid nanoparticle with enhanced penetration as compared to podophyllotoxin tincture (Chen et al., Citation2006). Furthermore, the results of in vitro skin permeation study were in accordance with the observation in CLSM penetration study with increased penetration and deposition of 5-FU-loaded elastic liposome. This is extremely important for clinical efficacy of the drug for the treatment of cutaneous diseases as described earlier. As a result of this carrier-based delivery, highest dye intensity and distribution with drug was obtained in the epidermis when applied topically for 24 h.
Figure 9. CLSM photomicrographs of cross-sections of hairless viable rat skin incubated on Franz diffusion cell with elastic liposome containing probe RR for 24 h, sectioned 0.5 μm below the cutting surface: (a) skin treated with EL3-S80-RR (0.5% w/v) and (b) skin treated with drug loaded EL3-S80-RR. Lens with magnification of 40× (immersion objective); excitation: 488 nm; emission: 560 nm.
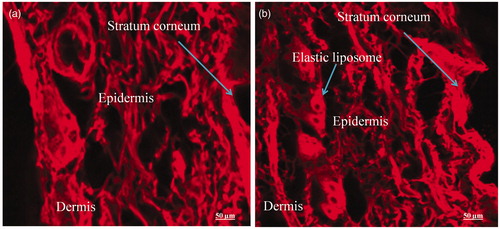
In vivo toxicity assessment
This potent drug is prescribed in the management of carcinomas of the breast, colon, head, neck, pancreas, rectum and stomach. 5-FU is also used topically for the treatment of various skin problems, particularly actinic or solar keratoses (Tutrone et al., Citation2003). Moreover, limited investigation has been carried out for the treatment of genital precancerous and cancerous lesion (Gonzalez Sanchez et al., Citation2002; Niwa et al., Citation2003). This investigation has mainly been focused to evaluate in vivo toxicity after treatment for 24 h. The utmost need had required due to serious scarcity of in vivo available in animal model. We used mice as animal model for assessment of toxicity of the developed vesicular carrier-based gel formulation and compared with control mice skin (). Animals were visualized for any behavioral changes after treatment. revealed hematoxylin and eosin-stained sections of mice skin with general anatomical architecture. Control skin showed complete intact margin of SC, epidermis and dermis layer. The epidermal layer was composed of cornified squamous layers, germinative and granular layers. Dermis layer consist collagen fibers, hair follicles, small capillaries and sebaceous glands in sub-epidermal region. It is noteworthy that the hydrophobic crystalline barrier of SC was extracted out at some places indicating that formulation was capable of enhancing drug permeation across the barrier significantly. This might be due to ethanolic content present in the formulation in trace quantity (7% v/v). Moreover, there were absence of any inflammatory infiltrates, visible malignancy and granulomatous pathology in the treated skin () as compared to control group. On the other hand, the isolated major visceral organs () were sectioned to identify any abnormalities observed after topical treatment with drug-loaded gel formulation. After histopathological examination of liver, kidney and heart, no serious changes have been observed in formulation-treated groups in their anatomy as compared to the control group. These findings suggested that the developed formulation for topical application of 5-FU was safe and effective for topical drug delivery using vesicular carrier system.
Figure 10. Representative histopathological sections of various organs of mice showing effect of in vivo topical application of 5-FU gel formulation in 28-d dermal toxicity study. (a) Control skin (40×), (b) treated skin, (c) control kidney, (d) treated kidney, (e) control liver, (f) treated liver, (g) control heart and (h) treated heart (10× for all other organs). Tissue sections were stained with hematoxylin and eosin.
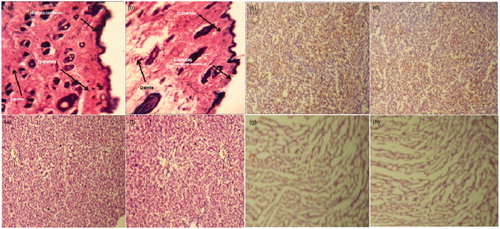
Conclusion
5-FU is a potent antineoplastic agent, widely used to cure solid tumor and skin problems. Presently, conventional therapeutic application is associated with a number of serious side effects either dose-dependent or drug-related unwanted effects. Topical drug delivery of 5-FU as compared to conventional oral drug delivery has found a place in the management of several dermatological disease including skin cancer. In vitro drug-related profile from optimized elastic liposome revealed that drug release pattern was slow and sustained as compared to free drug solution. Moreover in vitro skin permeation and deposition results showed enhanced flux rate effectively. From safety and tolerability point of view of formulations, we evaluated hemobiocompatibility assessment that was found to be in safe range and insignificant interaction with RBCs. Finally, histopathological study of the skin, after repeated dose toxicity application, demonstrated that optimized formulation did not cause any abnormality in general architecture of the skin. Thus the finding in this study was very encouraging for possible topical application of 5-FU in elastic liposome gel in the treatment of various skin-related cancer, solar-caused problem and skin actinic.
Declaration of interest
Authors report not any conflicts of interest.
References
- Bargman H, Hochman J. (2003). Topical treatment of Bowen’s disease with 5-FU. J Cutan Med Surg 7:101–5
- Barreiro-Iglesias R, Alvarez-Lorenzo C, Concheiro A. (2001). Incorporation of small quantities of surfactants as a way to improve the rheological and diffusional behavior of carbopol gels. J Control Release 77:59–75
- Bendas ER, Tadros MI. (2007). Enhanced transdermal delivery of salbutamol sulfate via ethosomes. AAPS PharmSciTech 8:E1–8
- Cevc G, Blume G, Schatzlein A. (1997). Transfersomes mediated transepidermal delivery improves the regio-specificity and biological activity of corticosteroids in vivo. J Control Release 45:211–26
- Chen-Yu G, Chun-Fen Y, Qui-Lu L, et al. (2012). Development of quercetin-loaded nanostructured lipid carrier formulation for topical delivery. Int J Pharm 430:292–8
- Chen H, Chang X, Du D, et al. (2006). Podophyllotoxin-loaded solid lipid nanoparticles for epidermal targeting. J Control Release 110:296–306
- Draize J, Woodard G, Calvery H. (1944). Methods for the study of irritation and toxicity of substance applied to the skin and mucous membranes. J Pharmacol Exp Ther 82:377–90
- Dubey V, Mishra D, Dutta T, et al. (2007). Dermal and transdermal delivery of an anti-psoriatic agent via ethanolic liposomes. J Control Release 123:148–54
- El Maghraby GM, Williams AC, Barry BW. (2001). Skin delivery of 5-Fluorouracil from ultra deformable and traditional liposomes in vitro. J Pharm Pharmacol 53:1069–76
- Epstein E. (1985). Fluorouracil paste treatment of thin basal cell carcinomas. Arch Dermatol 121:207–13
- Fraile RJ, Baker LH, Buroker TR, et al. (1980). Pharmacokinetics of 5-fluorouracil administered orally, by rapid intravenous and by slow infusion. Cancer Res 40:2223–8
- Fresta M, Villari A, Puglisi G, Cavallaro G. (1993). 5-Fluorouracil: various kinds of loaded liposomes: encapsulation efficiency, storage stability and fusogenic properties. Int J Pharm 99:145–56
- Gabrijelcic V, Sentjurc M. (1995). Influence of hydrogels on liposome stability and on the transport of liposome entrapped substances into the skin. Int J Pharm 118:207–12
- Garg T, Jain S, Singh HP, et al. (2008). Elastic liposomal formulation for sustained delivery of antimigraine drug: in vitro characterization and biological evaluation. Drug Deve Ind Pharm 34:1100–10
- Gonzalez Sanchez JL, Flores Murrieta G, Chavez Brambila J, et al. (2002). Topical 5-fluorouracil for treatment of vaginal intraepithelial neoplasms. Ginecol Obstet Mex 70:244–7
- Guo J, Ping Q, Sun G, Jiao C. (2000). Lecithin vesicular carriers for transdermal delivery of cyclosporine-A. Int J Pharm 194:201–7
- Holland JF, Frei III E, Bast RC Jr et al. (eds.). (1997). Cancer medicine. Philadelphia, PA: Lea & Febiger, 924–48
- Hussain A, Samad A, Singh SK et al. (2014). Nanoemulsion gel-based topical delivery of an antifungal drug: in vitro activity and in vivo evaluation. Drug Deliv, Early Online 1–16. [Epub ahead of print]
- Jain S, Jain N, Bhadra D, et al. (2005). Transdermal delivery of an analgesic agent using elastic liposomes: preparation, characterization and performance evaluation. Curr Drug Deliv 2:223–33
- Jain S, Tiwary AK, Jain NK. (2006). Sustained and targeted delivery of an anti-HIV agent using elastic liposomal formulation: mechanism of action. Curr Drug Deliv 3:157–66
- Jain SK, Gupta Y, Jain A, Rai K. (2008). Enhanced transdermal delivery of acyclovir sodium via elastic liposomes. Drug Deliv 15:141–7
- Lasch J, Laub P, Woblrab W. (1991). How deep do intact liposomes penetrate into human skin? J Control Release 18:55–8
- Lopez C, Maza A, Coderch L, et al. (1998). Direct formation of mixed micelle in the solublization of phospholipid liposomes by Triton X-100. FEBS Lett 426:314–18
- Mishra N, Gupta PN, Mahor S, et al. (2007). Liposomes as adjuvant for combination vaccines. Indian J Exp Biol 45:237–41
- Niwa K, Tagami K, Lian Z, et al. (2003). Topical vidarabine or 5-fluorouracil treatment against persistent HPV in genital (pre)cancerous. Oncol Rep 10:1437–41
- Nounuo MM, El-Khordagui LK, Khalafallah N. (2005). Release stability of 5-fluororacil concentrates, gels and lyophilized powder. Acta Pol Pharm 62:381–91
- Knowles PF, Marsh D. (1991). Magnetic resonance of membranes. Biochem J 274:625–41
- Khurana S, Jain NK, Bedi PMS. (2013). Nanoemulsion based gel for transdermal delivery of meloxicam: physico-chemical, mechanistic investigation. Life Sci 92:383–92
- Osterlind A, Berthelsen JG, Abildgaard N. (1991). Risk of bilateral testicular germ cell tumor in Denmark: 1960–1984. J Natl Cancer Inst 83:1391–5
- Özer AY. (1992). Stability studies on 5-FU liposomes. Drug Target Deliv 1:151–60
- Peters GJ, Lankelma J, Kok RM, et al. (1993). Prolonged retention of high concentrations of 5-fluorouracil in human and murine tumors as compared with plasma. Cancer Chemother Pharmacol 31:269–76
- Tsukada S, Ueda S, Ukada R. (1984). Preparation of liposome encapsulated antitumor drugs relationship between lipophilicity of and in vitro drug release. Chem Pharm Bull 32:1929–35
- Tutrone WD, Saini R, Caglar S, et al. (2003). Topical therapy for actinic keratoses I: 5-fluorouracil and imiquimod. Cutis 71:365–70
- Yeom S, Shin BS, Han S. (2014). An electron spin resonance study of non-ionic surfactant vesicles (niosomes). Chem Phys Lipids 181:83–9