Abstract
The purpose of this study was to develop an efficient dual-ligand based liposomal drug delivery system with targeting specificity as well as properties that would kill melanoma cells. Liposomes modified with transferrin (Tf) and cell-penetrating peptide TAT was prepared, which encapsulated two kinds of chemotherapy drugs, paclitaxel and doxorubicin (Tf/TAT-PTX/DOX-LP). The Tf ligands specifically bind to the overexpressed Tf receptors on the surface of melanoma cells, while the TAT ligands functioned as a classical cell penetrating peptide, helping dual-ligand liposomes be internalized by melanoma cells. The effect of dual-targeting system and “double-drug” combination therapy were evaluated both in vitro and in vivo. In vitro, cellular uptake, intracellular distribution and tumor spheroids penetration studies demonstrated that the system could not only be selectively and efficiently penetrate melanoma cells. Besides, apoptosis staining assay and cytotoxicity showed effective anti-tumor capability and obvious synergistic effect of combination therapy of PTX and DOX. In vivo imaging and fluorescent images of tumor section further demonstrated that Tf/TAT-PTX/DOX-LP had the highest tumor distribution. The results of these experiments demonstrated that double-drug liposomal drug delivery systems (DDS) had both enhanced targeting efficiency and increased therapeutic efficacy.
Introduction
Melanoma had been one of the most aggressive sorts of human cancer for a long time (Nestle et al., Citation1998). Usually melanoma could not be cured totally by surgical removal of the tumor or traditional chemotherapy (Young et al., Citation2006). As the surgical treatment is too difficult to guarantee clearing the tumor completely, and non-selective anti-cancer drugs would cause huge cytotoxicity to the normal cells. Therefore, different drug delivery systems (DDS) targeting to tumor sites had been designed to overcome these problems (Zhang et al., Citation2008; Malam et al., Citation2009; Chen et al., Citation2010; Li et al., Citation2011).
Among the targeting strategies to melanoma, anticancer drugs encapsulated within ligand-modified PEGylated liposomes (PEG-LPs) demonstrated increased affinity (Saul et al., Citation2006; Takara et al., Citation2010, Citation2012), improved cytotoxicity and, in many cases, enhanced treatment efficacy, compared with PEG-LPs (Stephenson et al., Citation2004; Kibria et al., Citation2011a,Citationb; Sharma et al., Citation2013). In this study, we chose transferrin (Tf) as a specific ligand, which could selectively target transferrin receptors (TfR) overexpressed on a large set of cancer cell (including melanoma, glioblastoma, breast carcinoma, etc.) (Eaveri et al., Citation2004). Then TfR-mediated endocytosis, a process by which cells externalized molecules (Salter-Cid et al., Citation1999), could internalize the Tf-modified liposomes. Compared to PEGylated liposomes without ligands, TfR targeting increased the interactions of liposomes with target cells, resulting in enhanced cellular uptake as well as better endosomal escape (Kibria et al., Citation2011b).
Although Tf was able to deliver a therapeutic moiety to target cells, its transferrin receptor saturation occurred on the surface of cancer cells made them inefficient to construct an effective targeted delivery system of high cellular uptake rate (Warren et al., Citation1997). To overcome this drawback, the trans-activating transduction (TAT) ligand, a frequently-used CPP (Watson & Edwards, Citation1999), was attached to the surface of the liposome as a cationic ligand. The former mechanism study showed that TAT ligand promoted the cell-penetrating efficiency through a non-specific endocytosis way (Torchilin, Citation2008). And it was reported that TAT could enter cells both in vitro and in vivo when being in conjugation with small molecules or bulky cargoes, such as peptides and phage encapsulated DNA (El-Sayed et al., Citation2009).
Doxorubicin (DOX) and paclitaxel (PTX) were among the most commonly used chemotherapeutic drugs with distinct solubility characteristics and different anticancer mechanisms (Jaracz et al., Citation2005), due to their excellent anti-tumor efficiency against various solid tumors. In detail, PTX was a mitotic inhibitor, which interfered with the normal breakdown of microtubules during the period of cell division (Lee et al., Citation2002), and DOX interacted with DNA by intercalation and inhibition of macromolecular biosynthesis (Tishler et al., Citation1992). However, the traditional use of single chemotherapeutic drug had also shown some limitations in anti-cancer treatment (Pérez-Tomás, Citation2006), such as no lasting efficacy, drug resistance, undesirable toxicity and other side effects. Fortunately, co-delivery of these two drugs simultaneously to the tumor cells could serve as a strategy to overcome these limitations. This method had been proved to minimize the amount of drug and to achieve a synergistic therapeutic effect when treating cancers in vitro and in vivo in previous study (Tishler et al., Citation1992). Therefore, we took the strategy of co-delivery of Paclitaxel and Doxorubicin to improve the treatment effect of the liposomal carrier.
Therefore, a kind of Tf and TAT co-modified liposomes encapsulated with both PTX and DOX (Tf/TAT-PTX/DOX-LP) was designed to target transferrin expressing cells. Corresponding experiment was operated to verify the targeting efficiency and therapeutic effect of dual-targeting liposomal drug delivery system. In vitro, the stability of liposomes in serum and drug release experiment was carried on to compare the parameters with conventional liposomes. The tumor spheroid uptake, intracellular distribution, cytotoxicity assay and inhibition effect of B16 experiments was carried on to examine whether this drug delivery system had advantages over control groups. In vivo, in-vivo imaging experiment was operated to achieve the same goal.
Methods
Materials
Soybean phospholipids (SPC) were purchased from Shanghai Taiwei Chemical Company (Shanghai, China). Cholesterol (CHO) was purchased from Chengdu Kelong Chemical Company (Chengdu, China). DSPE-PEG3500-Mal and DSPE-PEG1000-Mal were purchased from JENKEM Technology (Beijing, China). TAT peptide with terminal cysteine (Cys-AYGRKKRRQRRR) was synthesized by Chengdu KaiJie Biopharmaceutical Co., Ltd. (Chengdu, China). Transferrin peptide was purchased from Sigma Chemical Company (St. Louis, MO). DSPE-PEG2000 and 1,10-Dioctadecyl-3,3,30,30-tetramethylindotricarbocyanine iodide (DiR) was obtained from Biotium Chemical Company (Invitrogen, Waltham, MA). The Annexin V-FITC apoptosis detection kit and 4′,6-Diamidino-2-phenylindole (DAPI) were purchased from Beyotime Biotechnology Company, Limited. (Nantong, China). Paclitaxel (PTX) was purchased from AP Pharmaceutical Company, Limited. (Chongqing, China).
Plastic cell culture plates were purchased from Corning Incorporation (Corning, NY). Dulbecco’s modified Eagle’s medium (high glucose), foetal bovine serum (FBS), trypsin-EDTA (0.25%) and penicillin-streptomycin were purchased from Gibco (Invitrogen, Waltham, MA). All other reagents and chemicals of analytical grade were purchased from Hengxin Chemical Reagent (Chengdu, Sichuan, China). Sephadex G-50 column was purchased from Pharmacia Biotech (Piscataway, NJ).
B16 cell lines were obtained from the Chinese Academy of Sciences Cell Bank (Shanghai, China). Male C57BL/6 mice (20 ± 2 g) were purchased from Experiment Animal Center of Sichuan University. All the animal experiments adhered to the principles of care and use of laboratory animals and were approved by the Experiment Animal Administrative Committee of Sichuan University.
Synthesis of DSPE-PEG1000-TAT
DSPE-PEG1000-TAT was synthesized in the aqueous phase as described previously (Kuai et al., Citation2011). Briefly, chloroform was removed from DSPE-PEG1000-Mal (1 equiv) solution by rotary evaporation, and the obtained thin film was kept in vacuum over 4 h to remove the residual solvent. Then the thin film was hydrated in phosphate-buffered saline (PBS; pH 7.4) in a bath-type sonicator for about 1 min, and TAT peptide (3 equiv) in PBS (pH 7.4) was added into the micelle solution. The mixture was allowed to react at 4 °C under nitrogen for about 48 h. The excess TAT was removed by passing through a Sephadex-G50 column. The collected micelle solution was freeze-dried and then extracted by chloroform twice to remove the inorganic salts.
Synthesis of DSPE-PEG3500-Tf
DSPE-PEG3500-Tf was synthesized in the mixed solvent as described previously (Zong et al., Citation2014a). DSPE-PEG3500-Mal (1 equiv) were reacted with Tf-SH (1.5 equiv) in the mixed solvent of CHCl3/MeOH (V:V = 2:1) containing 3-fold molar excess of triethylamine in darkness at room temperature with stirring for about 48 h, and the reaction was traced by TLC until DSPE-PEG3500-Mal was completely consumed. The organic solvent was filtered, the filtrates were evaporated by rotary evaporation and the obtained thin film was kept in vacuum over night to remove the residual solvent. The thin film was redissolved by chloroform and filtered again to purify the production. The supernatants were evaporated by rotary evaporation and stored at −20 °C until used.
Liposomes preparation
Liposomes were prepared by the lipid hydration method (Schiffelers et al., Citation2003). All the abbreviations of our liposomal groups and their full names were listed in . The concentration of liposomes in in vivo experiment was 6 µmol/ml; and the concentration of liposomes in in vitro experiment was 3 µmol/ml. Briefly, various amounts of lipid materials () were dissolved in the mixed solvent of chloroform and methanol (V:V = 2:1). Then the organic solvent was removed by rotary evaporation. The obtained film was kept in vacuum for over 6 h to completely remove the residual organic solvent. The thin film was hydrated in 2 ml phosphate buffer solution (PBS, pH = 7.4) by incubating for 30 min at 37 °C, followed by a 1-min bath-type sonication. Then it was further intermittently sonicated by a probe sonicator in ice-bath at 80 W for 75 s. The free ammonium sulfate was removed by passing through a Sephadex G-50 column in PBS (pH 7.4) solution.
Table 1. The abbreviations of liposomes used in the article.
Table 2. The compositions of liposomes without drugs.
For the preparation of DOX-loaded liposomes, DOX (doxorubicin hydrochloride:phospholipids = 1:20, w/w) was added, mixed, and then incubated at 45 °C for 20 min. The PTX-loaded liposomes were made by the same way. Free DOX/PTX was removed by passing through a Sephadex G-50 column. The liposomes were stored at 4 °C for later use.
For the preparation of PTX and DOX-loaded liposomes (TAT-PTX/DOX-LP), the PBS was replaced by 1 ml 300 Mm (pH = 4) (NH4)2SO4 solution. The PTX-loaded liposomes were passed through a Sephadex G-50 gel-filtration column pre-equilibrated in PBS to exchange the external phase. Doxorubicin was then remote-loaded via an ammonium sulfate gradient method (Bolotin et al., Citation1994). In brief, PTX-loaded liposomes were pre-heated in 50 °C, and proper amount of 5 mg/ml Doxorubicin solution was added to incubate with the liposomes at 50 °C for 20 min, stirring gently to load Doxorubicin into the liposome inner phase. Free doxorubicin and paclitaxel was then removed by Sephadex G-50 gel-filtration. Liposomes were stored at 4 °C and used within 24 h of preparation.
Characterization of drug loaded liposomes
The hydrodynamic diameters, polydispersity index (PDI) and Zeta potential of liposomes were measured by using dynamic light scattering (DLS). The analysis was performed with 10 mW He–Ne laser (633 nm) at a scattering angle of 90° at 25 °C. The liposome was diluted with PBS (pH = 7.4) before measurement. The results were determined three times for each sample.
For encapsulation efficiency measurement (Shew & Deamer, Citation1985), a 0.1 ml liposome suspension was passed through a Sephadex G-50 gel-filtration column, and the contents of PTX and DOX were measured by high-performance liquid chromatography (Agilent Technologies Inc., Santa Clara, CA) through HPLC method at 227 nm and a fluorospectrophotometry (Ex = 470 nm, Em = 590 nm), respectively. The encapsulation efficiency was calculated as follows:
In vitro stability of liposomes in serum and drug release
In order to demonstrate the serum stability of liposomes, particle sizes and turbidity variations were monitored in the presence of 50% fetal bovine serum (FBS). Briefly, liposomes were mixed with equal volume of FBS under 37 °C with gentle shaking at 60 rpm. At predetermined time points (1 h, 2 h, 4 h, 8 h and 24 h), 200 μl of the sample was pipetted out and onto a 96-well plate to measure the transmittance at 750 nm by a microplate reader (Thermo Scientific Varioskan Flash, Waltham, MA) and another 200 μl was diluted to 1 ml with PBS for the particle size measurements by Malvern Zetasizer Nano ZS90 instrument (Malvern Instruments Limited, Malvern, UK).
To determine the release amount of PTX and DOX from liposomes, liposomes (1 ml) were placed in a dialysis tubing (molecular weight cut-off size 14 000) and dialyzed against 40 ml PBS at 37 °C for 48 h by gentle shaking. At designated time points, 200 μl aliquots were withdrawn from the incubation medium and replaced with equal volume of the fresh medium. At the end of 48 h, the dialysis bags were cut open and 2.0 ml 10% Triton X-100 was mixed thoroughly with the release medium. The concentrations of paclitaxel and doxorubicin were then measured by HPLC and a fluorospectrophotometry to calculate the maximum drug release amount.
Cell culture
B16 cells (a murine melanoma tumor cell line) were grown in Dulbecco’s modified Eagle’s medium (DMEM) containing 10% FBS, 100 μg/ml streptomycin and 100 U/ml penicillin. The cells were maintained at 37 °C in a humidified incubator under 5% CO2.
In vitro cellular uptake study
Intracellular distribution of liposomes by confocal laser scanning microscopy
B16 cells at a density of 1 × 105 cells/well were seeded in a six-well tissue culture plate. The cells were incubated for 24 h to 50% confluence and then incubated with 200 µl of 3 µmol/ml PEG-DOX-LP, Tf-DOX-LP, TAT-DOX-LP and Tf/TAT-DOX-LP for 4 h as described above. The cells were then washed three times with cold PBS, fixed in 4% paraformaldehyde at room temperature for 30 min, followed by cell nuclei staining with DAPI for 5 min before washed three times with PBS for confocal microscopy analysis. Leica SP2 CLSM (Wetzlar, Germany) was used to detect the intracellular uptake of doxorubicin (excitation/emission wavelength: 480 nm/540 nm) and investigate their cellular distribution. Fluorescent images of cells were analyzed using laser scanning confocal microscope (LSCM) Fluorescence Intensity Quantitative Software.
Qualitative analysis of cellular uptake by flow cytometry
An aliquot of 1.0 ml of B16 cells (1 × 105 cells/well) were seeded in a six-well tissue culture plate and incubated for 24 h at 37 °C under 5% CO2 until cells were grown nearly confluent (Gao et al., Citation2014a). Then, the medium was respectively replaced with 1.0 ml PEG-DOX-LP, Tf-DOX-LP, TAT-DOX-LP and Tf/TAT-DOX-LP, diluted with FBS free DMEM medium, with final DOX concentration of 20 μg/ml, and the plates were incubated at 37 °C under 5% CO2. After 4 h, the medium was removed and cell monolayer was suspended by brief treatment with trypsin and then washed three times with cold PBS. Then the cell samples were examined by flow cytometry using a FACScan (Becton Dickinson, San Jose, Canada). The intracellular doxorubicin was excited with an argon laser (488 nm), and fluorescence was detected at 560 nm. Files were collected of 10 000 gated events and analyzed with the FCS Express Software.
Evaluation of the cellular uptake mechanism
To investigate the mechanism of internalization of Tf/TAT-DOX-LP, 10 000 cells/well B16 were seeded in a six-well plate and the plate was incubated overnight at 37 °C. Before transfection, the cells were pre-incubated with different inhibitors for 30 min at 37 °C. Poly-lysine (800 μg/ml), amiloride (1.48 mg/ml), chlorpromazine (20 μg/ml), filipin (5 μg/ml) and free transferrin peptide (2 mg/ml) were added, respectively (Gao et al., Citation2014a). Tf/TAT-DOX-LP were added and the cells were incubated for 2 h at 37 °C and 4 °C in the presence or absence of inhibitors. The cells were washed twice by adding 1 ml of PBS supplemented to completely remove the surface-bound Tf/TAT-DOX-LP and the fluorescence intensity of doxorubicin was then determined, as described above. The fluorescence intensity was determined by flow cytometer (Cytomics FC500, Beckman Coulter, Miami, FL).
Co-localization of liposomes and lysosome
The intracellular localization of liposomes into the lysosomes and endosomes was determined using Lyso Tracker Blue, Invitrogen, Waltham, MA (Liu et al., Citation2014). Briefly, B16 cells (1 × 105 cells/well) were plated in a six-well cell culture plate in 1 ml of media and incubated overnight for attachment. Next day, the cells were treated with liposomes at a concentration of 100 nM for 4 h. The control cells were left untreated. After the predetermined incubation period, the cells were washed with PBS (pH = 7.4) and incubated with the media containing Lyso Tracker Blue (4 µl of 1 mM DMSO solution in 40 ml of media) for 1 h in the dark. Thereafter, the cells were washed twice with PBS and fixed for 20 min using 10% buffered formaldehyde. The images were observed by confocal microscope (FV1000, Olympus, Center Valley, PA).
Tumor spheroid uptake
B16 three-dimensional spheroids were established as per the previously described method (Gao et al., Citation2014b). Briefly, agarose was added into serum-free 1640 culture medium and heated to 80 °C for 30 min to form 2% (w/v) solution. 500 ml of agarose solution was added to each well of a 96-well culture plate. After cooling to room temperature, 100 μl of cells contained medium at a density of 1000 cells/well was seeded into each well. The culture plates were allowed to agitate gently for 5 min and cultured at 37 °C for 24 h. Seven days later, spheroids were ready to use with temperate size and tight cell interaction. The spheroids were treated with 20 μg/ml DOX-loaded liposomes for 4 h. Then, the spheroids were rinsed with PBS and fixed with 4% paraformaldehyde for 0.5 h. After being transferred to a glass layer, the fluorescent intensity was captured by CLSM (Leica, Wetzlar, Germany). Then dissociating the spheroids into cell suspensions, flow cytometry was performed to quantitatively determine the fluorescence intensity within tumor cells in spheroid models.
In vitro apoptosis staining assay
The quantitative analysis of apoptosis induced by different PTX/DOX-loaded liposomes was performed by Annexin V-FITC/PI double staining (Schutte et al., Citation1998). B16 cells were treated with PTX/DOX-loaded preparations (DOX/PTX = 20 μg/ml) for 6 h at 37 °C. At the end of the treatment, cells were harvested, washed with cold PBS, suspended in 500 μl binding buffer and stained by 5 μl Annexin V-FITC and 5 μl PI. The cells were incubated in the darkness for 15 min and measured by flow cytometer (Cytomics FC500, Beckman Coulter, Miami, FL).
In vitro cytotoxicity assay
Cytotoxicity of various liposomes was tested on B16 cells and bEnd.3 cells. Briefly, each well of a 96-well plate was seeded with 5000 cells and incubated for 48 h. The cells were then exposed to serial concentrations of liposomes diluted in culture medium. For the cytotoxicity of no drug-loaded liposomes, 100 µl of 6 µmol/ml was added to each well of 96-well plate to incubate with bEnd.3 cells (Youn et al., Citation2014). After cultured for 24 h at 37 °C, the cell monolayer was washed with PBS. Then, 20 μl of MTT solution (5 mg/ml) was added to each well, followed by incubating for another 4 h at 37 °C. Finally, the medium was replaced by 150 μl of DMSO, and the absorbance was read on Sunrise Absorbance Microplate Reader (TECAN, Grödig, Austria) at a wavelength of 490 nm. The experiment was carried out in triplicate. The data reported represent the means of triplicate measurement.
Growth inhibition of tumor spheroid
In vitro avascular B16 spheroids model was established using a lipid overlay system as reported previously (Carlsson & Yuhas, Citation1984). Then 100 μl of serum-PEG-PTX/DOX-LP, Tf-PTX/DOX-LP, TAT-PTX/DOX-LP, Tf/TAT-PTX-LP, Tf/TAT-DOX-LP and Tf/TAT-PTX/DOX-LP were added, respectively with the final concentration of DOX and PTX, all reached 20 μg/ml. The growth inhibition of the B16 spheroids was counted by measuring the size of B16 spheroid under an invert microscope (Chongqing Optical & Electrical Instrument, Co., Ltd., Chongqing, China) and the B16 tumor spheroids volume was calculated as reported previously.
In vivo imaging of liposomes in B16-bearing mice
Male C57BL/6 mice (6–8 weeks old, 18–22 g) inoculated 1/5 well of melanoma B16 cells (5 × 107 cells/well) subcutaneously in the right flank of the mice (Gao et al., Citation2014b). After the tumor volume increased to 50 mm3 a week later, 500 μl of DIR (100 µg/ml) was used to label liposomes at the concentration of 6 µmol/ml, and 0.3 ml liposomes were injected into the tail vein of mice. The blank group was injected in 0.3 ml PBS buffer. The in vivo imaging was performed at different time points (0.5 h, 1 h, 2 h and 4 h) post-injection using the in vivo IVIS spectrum imaging system (Perkin Elmer, Hopkinton, MA). At each time points, one mouse from each group was intraperitoneally injected with 5% chloral hydrate followed by heart perfusion with saline and 4% paraformaldehyde. Subsequently, the major organs were harvested and the ex vivo imaging of the organs was also captured.
Then, the organs were fixed in 4% paraformaldehyde for 24 h, and dehydrated with 15% and 30% sucrose solution until subsidence, respectively. Thereafter, the brains were frozen in OCT (Sakura, Torrance, CA) at −20 °C and cut into 10 μm sections. After counterstaining with 1 μg/ml DAPI for 5 min at room temperature, slides were observed using a fluorescence microscope (Leica, Wetzlar, Germany).
Statistical analysis
All of the quantitative measurements were performed in triplicate. The data were expressed as mean ± SD. Survival data were analyzed using the Kaplan–Meier method (IBM SPSS 19.0, Armonk, NY). For statistical analysis between two groups, Student’s t-test for independent means was used, and a value of p < 0.001 was considered as statistically significant.
Results
Characterization of the liposomes
It was reported that the size of liposomes, especially aggregation sizes in the presence of serum, is one of the most important factors governing the fate of liposomes in vivo (Maeda et al., Citation2004). The particle size of liposomes () was controlled around 120 nm and did not exhibit significant variations. Zeta potentials were mainly in the range of 4–10 mV. Either loaded with drugs or not, the particle size and the zeta potentials showed little variation. The aggregation characteristics in PBS or FBS were investigated subsequently. No increase in turbidity (indicated by the decrease of transmittance, T%) was observed after 24 h in PBS for all formulations (data not shown). The obtained liposomes could maintain the stability in PBS or 50% FBS at 37 °C for 24 h.
Table 3. The size, PDI, zeta-potential and encapsulating efficiency of different liposomes.
As shown as , the entrapment efficiencies of Paclitaxel and Doxorubicin were above 80 and 90%, respectively, and the concentration of Paclitaxel and Doxorubicin in the liposome suspensions was 130 and 260 μg/ml. Furthermore, compared with PEG-PTX/DOX-LP, Tf-PTX/DOX-LP and TAT-PTX/DOX-LP, the entrapment efficiency of Tf/TAT-PTX/DOX-LP group did not change much, i.e. it also shows a fine efficiency for carrying both paclitaxel and doxorubicin.
Transmission Electron Microscope (TEM) photograph showed that the Tf/TAT-PTX/DOX-LP was a regularly shaped spherical and had a smooth surface with a uniform distribution ().
In vitro serum stability in 50% FBS and drug release study
Particle sizes and transmittance variations as important parameters were monitored to explore the serum stability of liposomes (Senior & Gregoriadis, Citation1982). Liposomal particle stability against physiological condition is prerequisite for the further application in vivo, so 50% FBS was employed to mimic the in vivo situation. As shown in and (), the particle sizes and transmittance of all kinds of liposomes did not show any drastic increase, suggesting that all the liposomes did not generate large aggregation in the presence of serum within 24 h. As a result, all liposomes showed fine stability in the presence of 50% FBS (no aggregation occurred).
Figure 2. The variations in particle sizes (A) and turbidity (represented by transmittance) (B) of liposomes in 50% FBS. The PTX (C) and DOX (D) release profiles of liposomes in PBS over 48 h at 37 °C. The results are represented as means ± SD (n = 3).
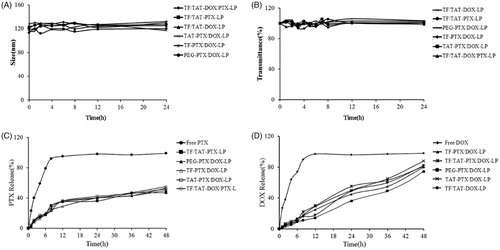
In vitro drug release study was also performed to examine the drug release property of liposomes in PBS (pH = 7.4). As shown in , compared with the rapid release of free Paclitaxel, all modified liposomes exhibited similar and sustained release manners, and no initial burst release was observed. Besides, as shown in , compared with the release profile of free Doxorubicin, all modified groups also had no initial burst release.
In vitro cellular uptake study
The efficient cellular uptake played an important role for the targeted delivery of liposomes. As shown in , the cellular uptake of different liposomes in B16 cells were investigated by CLSM. Compared to PEG-DOX-LP, single-ligand liposomes (TAT-DOX-LP, Tf-DOX-LP) had stronger red fluorescence, which could be clearly found in the cell nucleus and cytoplasm. Furthermore, the dual-ligand liposomes (Tf/TAT-DOX-LP) showed stronger fluorescence signals inside the B16 cells than the single-ligand liposomes indicating that Tf/TAT-DOX-LP was efficiently internalized by the B16 cells under the existence of both ligands.
Figure 3. Cellular uptake of DOX-loaded liposomes on B16 cells. (A) Four kinds of liposomes loaded DOX showed red autofluorescence and their uptake by the B16 cells recorded by confocal microscopy. Cell nuclei were stained blue with DAPI. (B) The cellular uptake was further investigated quantitatively by flow cytometry. The results are represented as means ± SD (n = 3). **p < 0.01; ***p < 0.001 versus Tf/TAT-DOX-LP.
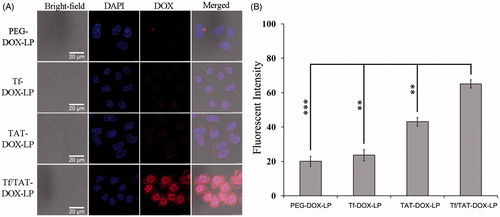
Then the cellular uptake of different liposomes in B16 cells was quantitatively evaluated using flow cytometer. As shown in , compared to the cellular uptake of Tf/TAT-DOX-LP, the PEG-DOX-LP group had only 1/14 fluorescent intensity, which showed statistically significant difference, i.e. Tf/TAT-DOX-LP had much higher cellular uptake rate than traditional long-circulating liposomes, PEG-DOX-LP. In addition, the dual-ligands group (Tf/TAT-DOX-LIP) was increased 8.7- and 2.8-fold than single-ligand liposomes (Tf-DOX-LP, TAT-DOX-LP) in B16 cells, respectively. The results were consistent with the result of CLSM. According to the above result, the dual-ligands liposomes, Tf/TAT-DOX-LP, showed the highest cellular uptake rate in B16 cells among all the other liposomes, which might be the effect of the synergistic effect of two ligands.
Cellular uptake mechanism of Tf/TAT-DOX-LP
In order to explore the endocytosis pathways for the dual-ligand liposomes, endocytosis inhibition assay was conducted. Amiloride, chlorpromazine and filipin were employed to block macropinocytosis-mediated, clathrin-mediated and caveolin-mediated endocytosis, respectively; and poly-lysine was chosen as positive charge inhibitor. 4 °C was selected to investigate the impact of energy on the cellular uptake (Gao et al., Citation2013). Many researchers have reported that clathrin-mediated endocytosis was the main endocytosis pathway for conventional liposomes (Brodin et al., Citation2000). However, the endocytosis pathway could be changed by the peptide or the ligand exposed on the nanoparticles.
According to the results, all the inhibitors exerted inhibition efficacy to different extent on B16 cells after 4 h of incubation with liposomes. After incubating under 4 °C, it is indicated that the endocytosis progress was energy-dependent. Poly-lysine positive charge inhibitor could drastically inhibit the cellular uptake of Tf/TAT-DOX-LP (down to 20%); and Genistein was able to inhibit the cellular uptake (down to 63%) as well, showing energy-dependent and caveolae-mediated endocytosis (). Free Tf peptide reduced the cellular uptake of Tf/TAT-DOX-LP in this case, suggesting that there was competitive inhibition and Tf/TAT-DOX-LP were internalized into the cells via specific receptors of Tf peptide.
Figure 4. The endocytosis inhibition on Tf/TAT-DOX-LP assay on B16 cells. The results are represented as means ± SD (n = 3). *p < 0.5; **p < 0.01; ***p < 0.001 versus Tf/TAT-DOX-LP.
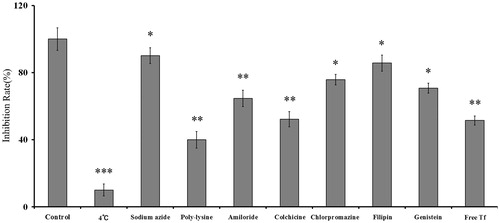
Generally, the uptake of Tf/TAT-DOX-LP was significantly suppressed by poly-lysine, amiloride, Genistein and free Tf. These results indicated that Tf/TAT-DOX-LP uptake by B16 cells was energy-dependent, macropinocytosis, charge-mediated endocytosis and Tf-mediated endocytosis pathway.
Evaluation of intracellular trafficking
Lysosome was an important organelle and might have an influence on drug bioavailability and activity. To further confirm the cellular uptake mechanism of liposomes, the study was followed by the co-localization of liposomes and lysosomes under CLSM. An obvious purple signal which signified the co-localization of the liposomes and lysosomes was observed ().
Figure 5. The co-localization of liposomes and lysosomes on B16 cells under CLSM. The Tf/TAT-DOX-LP group showed that the liposomes escaped from the lysosomes to great extent, while the other two group showed that the liposomes were highly trapped inside.
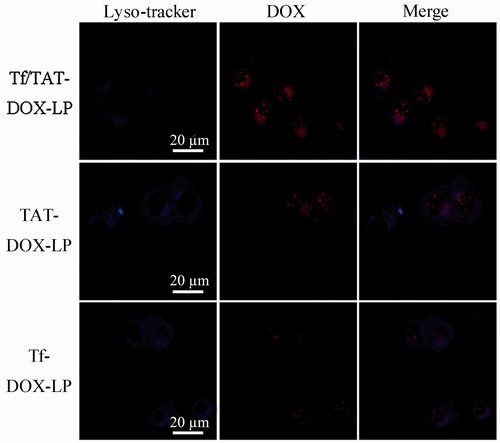
Lysosomes were dyed blue in the presence of Lyso-Tracker, while red fluorescence was from the autofluorescence of Doxorubicin, which represented the DOX-loaded liposomes. The red fluorescence intensity of TAT-DOX-LP and Tf/TAT-DOX-LP were much higher than that of Tf-DOX-LP, which was consistent with the result of cellular uptake. Almost all Tf-DOX-LP distributed in the lysosome of the cells, as revealed by the appearance of number of purple dots in the merged image. On the other hand, a large fraction of TAT-DOX-LP appeared in the cytoplasmic region outside the lysosome. This result coincided with previous reports (Qin et al., Citation2011). Meanwhile, the majority of Tf/TAT-DOX-LP could also escape from lysosome, indicating that TAT peptide more remarkably affected the uptake mechanism of Tf/TAT-DOX-LP. The uptake mechanism demonstrated that Tf/TAT-DOX-LP could successfully avoid the degradation of lysosomes, providing a possibility for the delivery of drugs into cells.
Tumor spheroid uptake
In this experiment, the tumor spheroids were cultured with different DOX-loaded liposomes for 4 h. As shown in , Tf/TAT-DOX-LP showed the highest tumor spheroid penetrating capability in confocal slicing. Besides, as shown in , Tf/TAT-DOX-LP exhibited stronger fluorescence than PEG-DOX-LP, Tf-DOX-LP and TAT-DOX-LP. These results were consistent with the results of cellular uptake study. Compared to the dual-ligand group liposomes, the tumor spheroid uptake of PEG-DOX-LP, Tf-DOX-LP, TAT-DOX-LP were obviously lower, their fluorescence were about 1/5, 1/4, 2/3 of that of Tf/TAT-DOX-LP, respectively. PEG-DOX-LP and Tf-DOX-LP showed significant differences when compared with the dual-ligand group. This result was consistent with the B16 cells uptake study, indicating that Tf/TAT-DOX-LP was efficiently internalized by the B16 tumor spheroid under the existence of both ligands.
Figure 6. (A) The confocal slicing of DOX-loaded liposomes on B16 tumor spheroid for 4 h using CLSM. The Tf/TAT-DOX-LP group showed the strongest fluorescence among all the groups, demonstrating that this group had the strongest penetrating capability. (B) The quantitative uptake of tumor spheroids 4 h after treatment with DOX-loaded liposomes. Data represent the mean ± SD (n = 3). *p < 0.5; **p < 0.01; ***p < 0.001 versus Tf/TAT-DOX-LP.
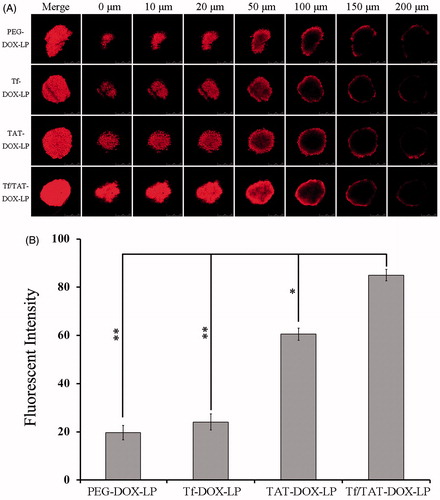
Growth inhibition of B16 tumor spheroids
The influence of various treatments on the growth of B16 spheroids was also investigated in this study. represents the B16 spheroid volume ratios after treatment with PEG-PTX/DOX-LP, Tf-PTX/DOX-LP, TAT-PTX/DOX-LP, Tf/TAT-PTX-LP, Tf/TAT-DOX-LP and Tf/TAT-PTX/DOX-LP, respectively. It was observed that B16 spheroids without any drug continued to grow in 1.38-fold volume of the control group after 7 days. However, the obvious reduction in volume of B16 spheroids was observed for all liposomal groups after 7 days treatment, indicating that B16 spheroids were both sensitive to DOX and PTX. Further, a remarkable treatment efficacy was observed in the experiment when using co-delivery liposomes of DOX and PTX. In detail, B16 spheroid volume ratio was 82.1, 70.4, 63.7, 81.9, 65.2 and 53.6% (compared to the control group) for PEG-PTX/DOX-LP, Tf-PTX/DOX-LP, TAT-PTX/DOX-LP, Tf/TAT-PTX-LP, Tf/TAT-DOX-LP and Tf/TAT-PTX/DOX-LP, respectively. Results showed that Tf/TAT-PTX/DOX-LP significantly improves the inhibitory effects on the 3D tumor spheroids. The highest inhibitory effect of dual-ligand group suggested that Tf/TAT-PTX/DOX-LP might not only have a synergistic effect of double ligands, but also have promoting efficacy to both PTX and DOX, which led to the improvement therapeutic effect on tumor spheroids inhibition.
In vitro cytotoxicity
The cytotoxicity of DOX-loaded liposomes was evaluated with MTT assay (). The DOX-IC50 and PTX-IC50 values on B16 cells were evaluated, respectively. For DOX-IC50 and PEG-DOX-LP showed the highest concentration to kill 50% of B16 cells, while the co-delivery and dual-modified liposomes only reached quarter of the concentration the former used. Besides, the single-drug group and single-modified group also showed higher concentration than the Tf/TAT-DOX/PTX-LP; and for PTX-IC50, the same result was observed, such as the concentration of PEG-PTX-LP was 9-fold of Tf/TAT-DOX/PTX-LP. This indicated that the cytotoxicity effect of the dual-ligand and co-delivery liposomes had remarkable treatment efficacy on killing B16 cells.
Table 4. IC50 values of representative drugs against B16 cells.
shows the apoptosis and necrosis dot diagrams of B16 cells treated with different liposomes. The left bar graph showed the percentage of living cells, PEG-DOX-LP group showed the highest survival rate, and Tf/TAT-PTX/DOX-LP the lowest. And the right bar graph showed the percentage of apoptotic and necrotic cells, Tf/TAT-PTX/DOX-LP induced the most prominent cell apoptosis and necrosis among various formulations, with 88.1 ± 2.01% of the cells observed in the apoptosis and necrotic quadrants, which was higher than that of PEG-PTX/DOX-LP (4.1%), Tf-PTX/DOX-LP (14.2%), TAT-PTX/DOX-LP (57.2%), TAT/TF-PTX-LP (43.5%) and TAT/TF-DOX-LP (78.2%), respectively. Based on apoptosis assays, it could be concluded that the apoptosis and necrotic effect of the PTX/DOX-loaded liposomes was markedly elevated by the co-modified by TAT and Tf ligands.
Figure 8. The apoptosis study on B16 cells after incubation with different liposomes. Data represent the mean ± SD (n = 3). The left bar graph showed the percentage of living (survival) cells, while the right bar graph showed the apoptotic and necrotic rate of B16 cells.
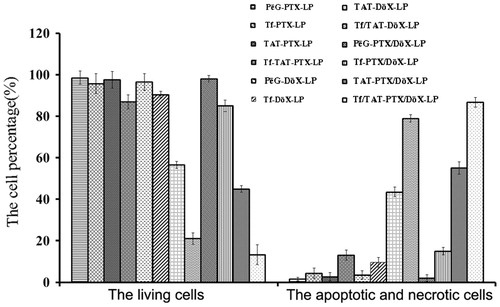
The cytotoxicity of DOX-loaded liposomes was also evaluated in bEnd.3 cells with MTT assay (). The DOX-IC50 and PTX-IC50 values on bEnd.3 cells were evaluated respectively. The group of Tf/TAT-PTX/DOX-LP also showed lower IC50 value than other groups, which meant higher cytotoxicity on bEnd.3 cells. However, compared with the IC50 value on B16 cells (), IC50 on bEnd.3 cells were a little higher, which meant a clearly different cytotoxicity on these two cells.
Table 5. IC50 values of representative drugs against bEnd.3 cells.
The cytotoxicity of no drug-loaded liposomes was also evaluated on bEnd.3 cells with MTT assay (). The cell viability of blank liposomes showed little cytotoxicity to endothelial cells, and the percentages were between 80 and 120%.
In vivo imaging of DIR-labeled liposomes in B16-bearing mice
To further confirm the dual-targeting effects in vivo, intracranial B16 tumor-bearing mice were utilized to evaluate the distribution of DIR-labeled liposomes. The ex vivo imaging of B16-bearing mice showed dual-targeting liposome (Tf/TAT-DIR-LP), which had high tumor targeting capability and resulted in stronger fluorescence intensity in the tumor than other groups during 24 h (). After systemic administration of DIR-loaded liposomes, different liposomal groups had various imaging effect under CLSM. The Tf/TAT-DIR-LP had a more obvious red region in the ex vivo tumor section, while other groups just showed slight red fluorescence, demonstrating that the cellular uptake rate of Tf/TAT-DIR-LP was the highest (). The image of isolated organ is as shown in . All groups had a strong accumulation in liver; and TAT/TF-PTX-DOX-LP group showed a little less accumulation than other groups, but still not showed significant difference.
Figure 10. In vivo and ex vivo images of the tumors of B16 bearing mice given different DIR loaded liposomes. (A = Blank, B = PEG-DIR-LP, C = TAT-DIR-LP, D = Tf-DIR-LP, E = Tf/TAT-DIR-LP).
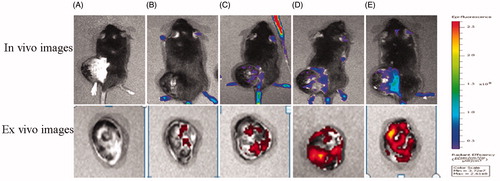
Discussion
Chemotherapy was crucial in the treatment of melanoma, however, the efficacy of free drug was usually limited due to the poor physiochemical properties, low stability, short circulating half-life and the toxicity to normal tissues (Allen & Cullis, Citation2004). Therefore, targeted delivery strategies were becoming more essential to overcome these problems. Non-targeted PEGylated liposomes have been widely used for delivering cytotoxic drugs to tumors via the EPR effect (Forssen et al., Citation1992; Zhang et al., Citation2008). However, these common liposomes had relatively small chance to get close to the targeting cells resulting in unsatisfying cellular uptake and poor endosomal escape. This obstacle would finally lead to a loss of the transport of drugs. Therefore, an active targeting liposomal drug delivery system was designed to achieve selective and excellent cellular uptake.
Many studies have indicated that the expression level of transferrin receptor (TfR) on tumor cells was much higher than that on normal cells (Daniels et al., Citation2006). Therefore, TfR was chosen as the targeted receptor in this study. As shown in , free transferrin (Tf) could inhibit the transport of liposomes modified with Tf ligand which indicated that Tf-modified liposomes was transported into cells through a TfR-mediated endocytosis (Qian et al., Citation2002; Suzuki et al., Citation2008). However, the TfR saturation was a limitation for transporting Tf-modified liposomes in large quantities (Warren et al., Citation1997).
Cell-penetrating peptides (CPPs), short peptide sequences that had no saturation phenomenon, have been used for drug or gene delivery for some time (Joliot & Prochiantz, Citation2004). Among all the CPPs, the TAT which had good cell membrane penetration ability was already exploited for the delivery of nucleotides, proteins, drugs and genes into cells (Fittipaldi & Giacca, Citation2005), so we chose TAT to break the limitation of TfR saturation. Hence, it is worth mentioning that why the length of DSPE-PEGs using to combine TAT and Tf were different. When the chains of DSPE-PEG3500-Tf were longer than DSPE-PEG1000-TAT, Tf ligands could reach Tf receptors and specifically identify them immediately. After the recognition, short chain of DSPE-PEG1000-TAT were exposed because of some DSPE-PEG3500-Tf chains breakage during recognition progress. As a result, TAT played an important role of cell penetrating peptides, which extremely enhanced the cellular penetrating capability. The increased uptake efficiency of the dual-ligand liposome was verified by flow cytometry (), and the semi-quantitative result demonstrated that the dual-ligand liposome (Tf/TAT-DOX-LP) demonstrated statistically significant difference compared with non-targeting PEGylated liposome group (). After incubation with four different groups of liposomes, the intracellular distribution of liposomes was observed by the confocal microscopy. A strong red fluorescent (from the autofluorescence of doxorubicin) signal and a purple signal could be clearly observed from the cellular membrane and the nuclei of melanoma (B16) cells in the dual-ligand group (Tf/TAT-DOX-LP), respectively. The red fluorescence of doxorubicin on the cellular membrane demonstrated the trans-membrane process of dual-ligand liposomes into the B16 cells. The purple signal was derived from the fluorescent signal overlay of doxorubicin and nuclear dye DAPI, demonstrating that doxorubicin could be transported into the nuclei. Lysosomal escape capability was also tested; and also an effective result has been observed (). The in vivo targeting effect of liposomes was evidenced by in vivo imaging and ex vivo tumor section ().
Recently, many multifunctional delivery systems have been designed for co-delivery of different guests, including micelles (Lee et al., Citation2009), liposomes (Zong et al., Citation2014b) and inorganic nanoparticles (Chen et al., Citation2009). Synergistic combination of two or more drugs was a promising strategy to overcome undesirable toxicity and other side effects by reducing dosage of each agent or accessing context-specific multiple targets (Chen et al., Citation2009). Paclitaxel and doxorubicin have been chosen to achieve an excellent synergistic therapeutic effect in melanoma treatment.
For an intact drug delivery system (DDS), particle size was a precondition and a significant factor which would affect the delivery process. The size between 100 and 200 nm was acknowledged as the best particle size for EPR (Maeda et al., Citation2000), which was the main mechanism for passive targeting of unmodified particle to tumor sites. In this study, the particle size of Tf/TAT-PTX/DOX-LP was controlled around 124 nm (). The dual-ligand liposome was negatively charged, which benefited the stability of particle size and transmittance under biologically relevant conditions ( and ). The release rate of the DDS was also examined ( and ).
After ensuring the stability and release rate of the dual-ligand liposome, experiments of therapeutic effect were carried out. To simulate a solid tumor environment, multicellular tumor spheroids were constructed for evaluating anticancer effect of the targeting liposomes. To test the penetrating ability and destructing effect, the slices of B16 tumor spheroids treated with DOX-loaded liposomes were observed by confocal microscopy. The Tf/TAT-DOX-LP group showed an obviously increased fluorescent intensity in each layer of the spheroids compared with the non-targeting PEGylated liposomes and the single-ligand liposomes, showing strong penetrating ability (). Besides, the destructing effect of different liposomes was also evaluated in a tumor spheroid growth inhibition experiment (). As acknowledged before, DOX bound to DNA by intercalation and motivated a series of biochemical events inducing apoptosis of tumor cells, and PTX could inhibit microtubules disassembly and promote the formation of unusually stable microtubules, thereby disrupting normal dynamic reorganization of the microtubule network required for mitosis and cell proliferation, and finally causing cell apoptosis (Wang et al., Citation2014). In the apoptosis study experiment (), the co-delivery groups showed higher cytotoxicity than these single-drug groups with the same drug concentration.
Therefore, this newly-developed dual-ligand liposome multidrug delivery system had the possibility to provide better cellular targeting ability and therapeutic effect. Consequently, this system would be an effective design for the treatment of melanoma in the future.
Conclusion
In this study, we successfully developed a highly efficient liposomal drug delivery system. These liposomes were modified with two ligands, Tf and TAT, and loaded with two kinds of drugs, namely, Paclitaxel and Doxorubicin. In vitro experiments showed high cellular uptake rate and tumor spheroid inhibition ability of this drug delivery system, while in vivo experiments exhibited high targeting efficiency. As a dual-targeting liposomal drug delivery system, it had better cell-targeting capability and higher cell-penetrating ability than single-ligand liposomes for melanoma cells. Besides, double-drug strategies also showed high anti-melanoma capability. Therefore, our drug delivery system might be quite potential to achieve excellent treatment effect for melanoma treatment.
Declaration of interest
The authors report no declarations of interest.
This work was funded by the National Natural Science Foundation of China (81373337).
References
- Allen TM, Cullis PR. (2004). Drug delivery systems: entering the mainstream. Science 303:1818–22
- Bolotin EM, Cohen R, Bar LK, et al. (1994). Ammonium sulfate gradients for efficient and stable remote loading of amphipathic weak bases into liposomes and ligandoliposomes. J Liposome Res 4:455–79
- Brodin L, Low P, Shupliakov O. (2000). Sequential steps in clathrin-mediated synaptic vesicle endocytosis. Curr Opin Neurobiol 10:312–20
- Carlsson J, Yuhas J. (1984). Liquid-overlay culture of cellular spheroids. Spheroids Cancer Res 1--23. DOI: http://dx.doi.org/10.1007/978-3-642-82340-4_1
- Chen AM, Zhang M, Wei D, et al. (2009). Co-delivery of Doxorubicin and Bcl-2 siRNA by mesoporous silica nanoparticles enhances the efficacy of chemotherapy in multidrug-resistant cancer cells. Small 5:2673–7
- Chen W, Meng F, Cheng R, Zhong Z. (2010). pH-Sensitive degradable polymersomes for triggered release of anticancer drugs: a comparative study with micelles. J Control Release 142:40–6
- Daniels TR, Delgado T, Rodriguez JA, et al. (2006). The transferrin receptor, part I: biology and targeting with cytotoxic antibodies for the treatment of cancer. Clin Immunol 121:144–58
- Eaveri R, Ben-Yehudah A, Lorberboum-Galski H. (2004). Surface antigens/receptors for targeted cancer treatment: the GnRH receptor/binding site for targeted adenocarcinoma therapy. Curr Cancer Drug Targets 4:673–87
- El-Sayed A, Futaki S, Harashima H. (2009). Delivery of macromolecules using arginine-rich cell-penetrating peptides: ways to overcome endosomal entrapment. AAPS J 11:13–22
- Fittipaldi A, Giacca M. (2005). Transcellular protein transduction using the Tat protein of HIV-1. Adv Drug Deliv Rev 57:597–608
- Forssen EA, Coulter DM, Proffitt RT. (1992). Selective in vivo localization of daunorubicin small unilamellar vesicles in solid tumors. Cancer Res 52:3255–61
- Gao H, Yang Z, Cao S, et al. (2014a). Tumor cells and neovasculature dual targeting delivery for glioblastoma treatment. Biomaterials 35:2374–82
- Gao H, Yang Z, Zhang S, et al. (2013). Glioma-homing peptide with a cell-penetrating effect for targeting delivery with enhanced glioma localization, penetration and suppression of glioma growth. J Control Release 172:921–8
- Gao HL, Yang Z, Zhang S, et al. (2014b). Study and evaluation of mechanisms of dual targeting drug delivery system with tumor microenvironment assays compared with normal assays. Acta Biomater 10:858–67
- Jaracz S, Chen J, Kuznetsova LV, Ojima I. (2005). Recent advances in tumor-targeting anticancer drug conjugates. Bioorg Med Chem 13:5043–54
- Joliot A, Prochiantz A. (2004). Transduction peptides: from technology to physiology. Nature Cell Biol 6:189–96
- Kibria G, Hatakeyama H, Harashima H. (2011a). A new peptide motif present in the protective antigen of anthrax toxin exerts its efficiency on the cellular uptake of liposomes and applications for a dual-ligand system. Int J Pharm 412:106–14
- Kibria G, Hatakeyama H, Ohga N, et al. (2011b). Dual-ligand modification of PEGylated liposomes shows better cell selectivity and efficient gene delivery. J Control Release 153:141–8
- Kuai R, Yuan W, Li W, et al. (2011). Targeted delivery of cargoes into a murine solid tumor by a cell-penetrating peptide and cleavable poly(ethylene glycol) comodified liposomal delivery system via systemic administration. Mol Pharm 8:2151–61
- Lee AL, Wang Y, Cheng HY, et al. (2009). The co-delivery of paclitaxel and Herceptin using cationic micellar nanoparticles. Biomaterials 30:919–27
- Lee S, Baek M, Kim H-Y, et al. (2002). Mechanism of doxorubicin-induced cell death and expression profile analysis. Biotechnol Lett 24:1147–51
- Li X, Zhou H, Yang L, et al. (2011). Enhancement of cell recognition in vitro by dual-ligand cancer targeting gold nanoparticles. Biomaterials 32:2540–5
- Liu YR, Fang JX, Kim YJ, et al. (2014). Codelivery of doxorubicin and paclitaxel by cross-linked multilamellar liposome enables synergistic antitumor activity. Mol Pharm 11:1651–61
- Maeda H, Wu J, Sawa T, et al. (2000). Tumor vascular permeability and the EPR effect in macromolecular therapeutics: a review. J Control Release 65:271–84
- Maeda N, Takeuchi Y, Takada M, et al. (2004). Anti-neovascular therapy by use of tumor neovasculature-targeted long-circulating liposome. J Control Release 100:41–52
- Malam Y, Loizidou M, Seifalian AM. (2009). Liposomes and nanoparticles: nanosized vehicles for drug delivery in cancer. Trends Pharm Sci 30:592–9
- Nestle FO, Alijagic S, Gilliet M, et al. (1998). Vaccination of melanoma patients with peptide- or tumorly sate-pulsed dendritic cells. Nat Med 4:328–32
- Pérez-Tomás R. (2006). Multidrug resistance: retrospect and prospects in anti-cancer drug treatment. Curr Med Chem 13:1859–76
- Qian ZM, Li H, Sun H, Ho K. (2002). Targeted drug delivery via the transferrin receptor-mediated endocytosis pathway. Pharmacol Rev 54:561–87
- Qin Y, Chen H, Zhang Q, et al. (2011). Liposome formulated with TAT-modified cholesterol for improving brain delivery and therapeutic efficacy on brain glioma in animals. Int J Pharm 420:304–12
- Salter-Cid L, Brunmark A, Li Y, et al. (1999). Transferrin receptor is negatively modulated by the hemochromatosis protein HFE: implications for cellular iron homeostasis. Proc Natl Acad Sci 96:5434–9
- Saul JM, Annapragada AV, Bellamkonda RV. (2006). A dual-ligand approach for enhancing targeting selectivity of therapeutic nanocarriers. J Control Release 114:277–87
- Schiffelers RM, Koning GA, Ten Hagen TLM, et al. (2003). Anti-tumor efficacy of tumor vasculature-targeted liposomal doxorubicin. J Control Release 91:115–22
- Schutte B, Nuydens R, Geerts H, Ramaekers F. (1998). Annexin V binding assay as a tool to measure apoptosis in differentiated neuronal cells. J Neurosci Methods 86:63–9
- Senior J, Gregoriadis G. (1982). Stability of small unilamellar liposomes in serum and clearance from the circulation: the effect of the phospholipid and cholesterol components. Life Sci 30:2123–36
- Sharma G, Modgil A, Layek B, et al. (2013). Cell penetrating peptide tethered bi-ligand liposomes for delivery to brain in vivo: biodistribution and transfection. J Control Release 167:1–10
- Shew R, Deamer D. (1985). A novel method for encapsulation of macromolecules in liposomes. Biochim Biophys Acta 816:1–8
- Stephenson SM, Low PS, Lee RJ. (2004). Folate receptor-mediated targeting of liposomal drugs to cancer cells. Methods Enzymol 387:33–50
- Suzuki R, Takizawa T, Kuwata Y, et al. (2008). Effective anti-tumor activity of oxaliplatin encapsulated in transferrin-PEG-liposome. Int J Pharm 346:143–50
- Takara K, Hatakeyama H, Kibria G, et al. (2012). Size-controlled, dual-ligand modified liposomes that target the tumor vasculature show promise for use in drug-resistant cancer therapy. J Control Release 162:225–32
- Takara K, Hatakeyama H, Ohga N, et al. (2010). Design of a dual-ligand system using a specific ligand and cell penetrating peptide, resulting in a synergistic effect on selectivity and cellular uptake. Int J Pharm 396:143–8
- Tishler RB, Geard CR, Hall EJ, Schiff PB. (1992). Taxol sensitizes human astrocytoma cells to radiation. Cancer Res 52:3495–7
- Torchilin VP. (2008). TAT peptide-mediated intracellular delivery of pharmaceutical nanocarriers. Adv Drug Deliv Rev 60:548–58
- Wang Y, Ma S, Xie Z, Zhang H. (2014). A synergistic combination therapy with paclitaxel and doxorubicin loaded micellar nanoparticles. Colloids Surf B: Biointerfaces 116:41–8
- Warren RA, Green FA, Enns CA. (1997). Saturation of the endocytic pathway for the transferrin receptor does not affect the endocytosis of the epidermal growth factor receptor. J Biol Chem 272:2116–21
- Watson K, Edwards RJ. (1999). HIV-1 trans-activating (Tat) protein: both a target and a tool in therapeutic approaches. Biochem Pharmacol 58:1521–8
- Youn P, Chen Y, Furgeson DY. (2014). A myristoylated cell-penetrating peptide bearing a transferrin receptor-targeting sequence for neuro-targeted siRNA delivery. Mol Pharmaceut 11:486–95
- Young SE, Martinez SR, Essner R. (2006). The role of surgery in treatment of stage IV melanoma. J Surg Oncol 94:344–51
- Zhang L, Gu FX, Chan JM, et al. (2008). Nanoparticles in medicine: therapeutic applications and developments. Clin Pharmacol Ther 83:761–9
- Zong T, Mei L, Gao H, et al. (2014a). Synergistic dual-ligand doxorubicin liposomes improve targeting and therapeutic efficacy of brain glioma in animals. Mol Pharm 11:2346–57
- Zong T, Mei L, Gao H, et al. (2014b). Enhanced glioma targeting and penetration by dual-targeting liposome co-modified with T7 and TAT. J Pharm Sci 103:3891–901