Abstract
In this study, a novel amperometric glucose biosensor was developed by immobilizing glucose oxidase (GOX) by cross-linking via glutaraldehyde on electrochemically polymerized polypyrrole-poly(vinyl sulphonate) (PPy–PVS) films on the surface of a platinum (Pt) electrode. Electropolymerization of pyrrole and poly(vinyl sulphonate) on the Pt surface was carried out with an electrochemical cell containing pyrrole and poly(vinyl sulphonate) by cyclic voltammetry between −1.0 and + 2.0 V (vs.Ag/AgCl) at a scan rate of 50 mV/s upon the Pt electrode. The amperometric determination was based on the electrochemical detection of H2O2 generated in enzymatic reaction of glucose. Determination of glucose was carried out by the oxidation of enzymatically produced H2O2 at 0.4 V vs. Ag/AgCl. The effects of pH and temperature were investigated and optimum parameters were found to be 7.5 and 65°C, respectively. The effect of working potential was investigated and optimum potential was determined to be 0.4 V. The operational stability of the enzyme electrode was also studied. The response of the PPy/PVS-GOX glucose biosensor exhibited good reproducibility with a relative standard deviation (RSD) of 2.48%. The glucose biosensor retained 63% of initial activity after 93 days when stored in 0.1 M phosphate buffer solution of pH 7.5 at 4°C. With the low operating potential, the biosensor demonstrated little interference from the possible interferants.
Introduction
The most important endocrine disorder of carbohydrate metabolism is diabetes mellitus (Adlan and Bondugulapati 2010). Diabetes mellitus is the most frequent cause of heart disease, kidney failure, and blindness, and widely recognized as one of the leading causes of death and disability in the world (Rahman et al. 2010). The significance of glucose in human metabolism is well known, as is the fact that the defects in glucose level lead to complications of diabetes (Shankaran et al. 2003). This metabolic disorder is reflected by blood glucose concentrations higher or lower than the normal range of 80 - 120 mg/dL (4.4 - 6.6 mM) (Wang 2008). Moreover, glucose concentration is significant in food analysis (Yildiz et al. 2005). Therefore, it is very important to have a selective, highly sensitive, fast and low-cost technique for glucose determination. Several methods, such as spectrometry (Shi et al. 1997, Steegmans et al. 2004, Dohnal et al. 2010), HPLC (Sesta 2006, Aljane et al. 2007), polarometry (Malik and Cote 2010), and capillary electrophoresis (Wang et al. 2011), have been reported for glucose analysis. However, these conventional methods are time-consuming, costly, less accurate, and require difficult sample pre-treatment. In the past decades there has been much interest in the design and development of biosensors due to their fast response, small size, high sensitivity, and selectivity (Yildiz et al. 2005, Crouch et al. 2005, Wanga et al. 2011).
Biosensors containing enzymes, which have unique ability to recognize target molecules quickly and accurately in a complex system, have been widely applied in chemistry and biology (Yildiz et al. 2005, Rauf et al. 2006). Oxido-reductase enzyme electrodes are a great group of biosensors, accounting for over 90% of the existing amperometric enzyme-based biosensors. The side product of the flavin-oxidase enzymes reactions is generally hydrogen peroxide, formed by the enzyme-catalyzed oxidation of the analyte by dissolved molecular oxygen (Vidal et al. 1999, Vidal et al. 2004).
Conducting polymers have been applied to biosensors since they can be used both as immobilization matrices and as redox systems for the transfer of electrical charge (Singh et al. 2004, Gerard et al. 2002). Also, conducting polymer-based enzyme electrodes have been predicted to exhibit notable operational stability and rapid response time. Since the catalytic center of an enzyme is generally located deep within a protein molecule, the chief purpose is to create redox active films on the electrodes that are capable of efficiently mediating electron transfer between the active sites and the electrodes (Singh et al. 2006). Poly-conjugated conducting polymers such as polypyrrole or polyaniline have been widely studied as a matrix for immobilization of enzymes (Arslan et al. 2006, Çete et al. 2006).
Polypyrrole is one of the most used conducting polymers for biosensor applications because it has good biocompatibility, environmental and chemical stability, and high conductivity, and can be easily polymerized (Gade et al. 2007a, 2007b, 2007c, Singh et al. 2009).
Doping materials such as Nafion, poly(vinyl alcohol), poly(methylmethacrylate), poly(styrene sulphonate), poly (vinyl sulphonate), dodecylbenzene sulphonate, and p- toluene sulphonate can increase the conductivity, stability, and mechanical strength of PPy matrix. It was reported that the incorporation of a large-sized dopant anion, like poly(vinyl sulphonate) (PVS), into PPy films during electropolymerization makes PPy film more porous and sulphonate ions of the PPy–PVS composite films provide a charged surface for electrostatic interaction between the enzyme and the surface (Arora et al. 2007, Gade et al. 2006, Newman et al. 1995).
Immobilization of the enzyme onto the electrode surface is a crucial step in mounting amperometric biosensors. Some immobilization techniques, such as physical or chemical adsorption (Yildirimoğ lu et al. 2009), covalent binding (Wan et al. 2010), Langmuir–Blodgett (Collings and Caruso 1997), and layer-by layer (LBL) (Hodak et al. 1997), have been advanced for the immobilization of enzymes on many matrices. In this study, cross-linking method via glutaraldehyde was chosen for the immobilization of glucose oxidase.
The amperometric detection of hydrogen peroxide is normally performed anodically (e.g. oxidation at + 700 mV with a Pt working electrode), but is drastically influenced by many simply oxidizable interferants available in real samples (Karyakin 2001). The effect of several possible interfering substances on the glucose biosensor based on the PPy–PVS electrode was investigated at + 0.40 V.
The aim of this study is to develop a new glucose biosensor by immobilization of glucose oxidase by cross-linking via glutaraldehyde on electrochemically polymerized polypyrrole-poly(vinyl sulphonate) (PPy–PVS) film. The optimum working conditions as a working potential, the pH and temperature were determined via application of 0.4 V with respect to the Ag/AgCl electrode. Effects of the immobilization process on kinetic parameters, storage, and reuse capability of the enzyme were investigated.
Materials and methods
Instrumentation and reagents
All electrochemical experiments were performed using an Epsilon EC electrochemical analyzer. A conventional three-electrode system was carried out with a Pt plate (0.5 cm2) as the working electrode, an Ag/AgCl (3M KCl) as the reference electrode, and a platinum wire as the counter electrode. The pH values of the buffer solutions were measured with an ORION Model 720A pH-ionmeter. Temperature control was accomplished with a Grant W14 thermostat. The scanning electron micrograph was recorded using a JEOL JSM-6060LV SEM machine. Glucose oxidase (EC 1.1.3.4. from Aspergillus Niger purified from the microorganism and with an activity of 5204 U/ml) and glucose were purchased from Sigma. Pyrrole and PVS was supplied by Fluka. All other chemicals were supplied from Sigma. All the solutions were prepared by the use of distilled water. Glucose stock solution was allowed to mutarotate for at least 24 h at room temperature prior to use and stored at 4oC.
Preparation of Pt/PPy-PVS film electrode
The surface of the Pt plate electrode was covered by the electropolymerization of pyrrole and the poly(vinyl sulphonate). The electrode was immersed in a 10 mL solution of 0.1M pyrrole and 2.5 mL aqueous solution of the sodium salt of poly(vinyl sulphonate) (25%). The solution was purged with argon in order to remove the oxygen. The electropolymerization of pyrrole upon the electrode surface was accomplished by the cyclic voltammetric scans between −1.0 and + 2.0 V at a scan rate of 50mV/s (Chaubey et al. 2000). The electrode was washed with buffer solution after the coating procedure.
Immobilization of glucose oxidase on Pt/PPy-PVS film electrode
Immobilization of glucose oxidase was carried out by the approach of cross-linking via glutaraldehyde. A solution occuring from 50 µL of the stock solution of glucose oxidase (5204 U/ml), 50 µL 0.1M phosphate buffer at a pH of 7.5, 1 mg of bovine serum albumin, and 30 µL 2.5% glutaraldehyde solution was droppped onto the surface of PPy–PVS film. The electrode was dried at room temperature, and washed with buffer solution (pH 7.5 0.1M phosphate buffer) several times in order to remove the non-immobilized excess enzyme and glutaraldehyde. The immobilized enzyme electrode was kept in a refrigerator at 4oC in phosphate buffer when it was not in use (Karyakin 2001).
Amperometric biosensor measurements
The amount of glucose can be determined by measuring the anodic current of oxidation of H2O2, produced in the reaction as given in (Witt et al. 2000).
Figure 1. Schematic diagram of GOD reaction; glucose oxidase catalyzes the oxidation of β-D-glucose by molecular oxygen to δ-gluconolactone.
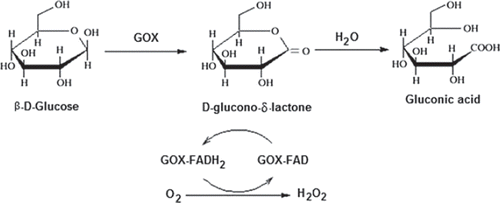
The anodic oxidation of H2O2 is given by the equation:
H2O2 → O2 + 2H + + 2e − (1)
An aqueous solution containing 0.1M phosphate buffer at (pH 7.5) and 0.1M sodium perchlorate was added to the cell. After the background current reached stable value (ia), glucose solution was added to the cell using a micropipette and stirred for five minutes. The response of the biosensor against glucose was measured (ib) after 3.20 minutes following the application of a constant potential of 0.4 V with respect to the Ag/AgCl electrode. The current values (Δi = ib − ia) were plotted against the concentration of glucose. Research on operational and storage stability as well as effects of pH and temperature was carried out using 5 × 1025 M concentration of glucose.
Results and Discussion
In this paper, we described a biosensor for glucose determination with cross-linking of glucose oxidase (GOX) on polypyrrole-poly(vinyl sulphonate) (PPy-PVS) composite film. The paramaters affecting the performance of the biosensor were examined.
Evaluation of SEM
Scanning electron microscopy (SEM) was used to observe the surface changes of the PPy-PVS film when the enzyme was immobilized. The PPy/PVS and PPy/PVS-GOX electrodes were dried on a moist atmosphere before analysis. The surface morphologies of PPy/PVS-GOX film were completely different compared to the film produced in the absence of glucose oxidase (). It can be seen that the surface morphology of the PPy-PVS film was a cauliflower-like structure. For the PPy/PVS-GOX film, the cauliflower-like structure was significantly flattening.
Effect of amount of glutaraldehyde on the response of biosensor
In the construction of the biosensor, one of the suitable enzyme immobilization methods is cross-linking using glutaraldehyde (Gouda et al. 2002). To improve the performance of the biosensor, various factors influencing the response of the sensor, such as amount of glutaraldehyde, were investigated. Different amounts of glutaraldehyde solution (i.e., 7.5, 15, 30, 60 µL for glutaraldehyde 2.5% glutaraldehyde solution) were used for immobilization of glucose oxidase. The results demonstrated that the activity of the cross-linking system increased when the amount of glutaraldehyde was increased from 7.5 to 30 µL (). The same results with 30µL were observed when 60µL of glutaraldehyde amount was used. These results were explained by the amount of immobilized glucose oxidase. When the concentration of glutaraldehyde was low, the amount of immobilized protein was low (Tan et al. 2009). For this reason, the current response of the glucose biosensor was decreased when the low glutaraldehyde concentration was used. But if the concentration of glutaraldehyde was higher, the immobilization film was thicker, causing a higher diffusion barrier (Tan et al. 2009). In addition, the experiment of reusability was carried out for four different enzyme electrodes. The results showed that the enzyme electrode prepared with 30 µL glutaraldehyde had the best results (). In this study, 30 µL of glutaraldehyde, which was observed to give maximum activity with reasonable mechanical stability, was selected in the further characterization experiments.
Figure 3. Effect of the amount of glutaraldehyde on the amperometric response of glucose biosensor (0.1 M, pH 7.5 phosphate buffer, 25°C).
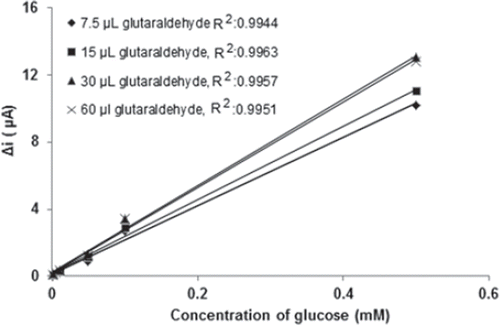
Table I. Effect of the amount of glutaraldehyde on the reproducibility of glucose biosensor.
Effect of working potential on the response of biosensor
Determination of the working potential was carried out on five different potentials (0.1, 0.2, 0.3, 0.4, 0.5 V). Effect of the working potential was studied using varying concentrations (1.0×10−6 M, 1.0×10−5 M, 5.0×10−5 M, 1.0×10−4 M and 5.0×10−4 M) of glucose. shows the effect of working potential on the steady-state current of the biosensor in the potential range from 0.3 to 0.5 V in pH 7.5 phosphate buffer solution. The current response of the biosensor increased when the working potential shifted from 0.3 to 0.5 V. This expected case was explained by increasing oxidation tendency of the electroactive species when the applied potential was increased. In the studies which were made in 0.10 V and 0.20 V, current values were observed to be nearly zero. Because of this, the current values that were measured at these potentials were not shown in the graphic. It is known that interference effects of the substances present in body fluids (e.g., ascorbic acid, uric acid) are more significant at high potentials (Zhang et al. 2007). Therefore, 0.4 V was selected as a working potential.
Effect of pH and temperature on the response of biosensor
The enzyme activity drastically depends on temperature and pH since too high or low values of these may inactivate the enzyme. Because enzyme activity is dependent on the ionization state of the amino acids in the active site, pH plays a significant role in maintaining the proper conformation of an enzyme (Bankar et al. 2009). Optimum pH for immobilized glucose oxidase was determined by measuring the activity of immobilized enzyme in buffers of different pH values ranging from 5 to 8. The effect of pH on the behavior of the enzyme electrode was studied with 0.1 M phosphate buffer solution containing 0.05 mM glucose. The response of the biosensor was increased as the pH was increased up to pH 7.5 (). Immobilized glucose oxidase enzyme showed maximum activity at pH 7.5. Although immobilization usually changes the optimum pH of enzyme, this was not observed in the present case (optimum pH was 7.5 in both free and immobilized enzymes). The experimental results showed no change due to immobilization in the ionic environment of the carrier around enzyme actives sites.
Temperature is an important factor which has a significant effect on enzyme activity. The response of the biosensor was evaluated at different incubation temperatures from 20 to 70°C. The effect of temperature on the behavior of the enzyme electrode was studied with 0.1 M phosphate buffer solution at pH 7.5 containing 0.05 mM glucose. As illustrated in , current response of biosensor gradually increased with increasing temperature and reached a maximum at 65°C. However, the maximal activity of free GOX was observed at 55°C. The thermal stability of the enzyme was improved after immobilization, indicating that this immobilized GOX is a promising candidate for the development of heat-resistant glucose sensor.
Enzymes can be denaturated at the long-time incubation temperature at 65°C. Therefore, the temperature of 25°C was chosen as a working temperature for all further experiments. Among glucose biosensors, pH and temperature values other than pH 7.5 and 65°C were employed in the literature (pH 6.0–7.0, 35–40°C) (Retam et al. 2004, Li and Lin 2007). This was attributed to the fact that the polymer used and the type of immobilization were different.
Effect of substrate concentration on the response of biosensor
The effect of the substrate concentration on the reaction rate, catalyzed by immobilized glucose oxidase was studied using varying initial concentrations (1 × 10−6−1 × 10−1M) of glucose (). The detection limit of the biosensor was 1.0 × 10 − 7 M and the response time of the biosensor was 200s. There were two linear parts in the region up to 1.0mM ranging between 1.0× 10−6-5.0×10−5M (R2:0.9977; ) and 5.0×10−5M−1.0mM (R2:0.9963; ). The Michaelis–Menten constant (Km), shows the enzyme–substrate kinetics and Vmax is the maximum rate for enzymatic reaction. Kinetic parameters Km(app) and Imax for the enzyme biosensor were obtained at constant temperature (25°C) and pH (pH 7.5) for varying substrate concentrations. Km(app) and Imax were calculated as 0.44 mM and 30.2 μA/min, respectively, by using 1/[glucose] − 1/Δi graph (Lineweaver-Burk plot) (). For the free enzyme, the maximum current was 12.2 μA/min with Km(app) 0.12 mM. Kinetic constant, Km(app) value for the immobilized glucose oxidase were found to be higher than that of the free glucose oxidase. The increase in the value of Km(app) (decreased affinity) could be attributed to the lower accessibility of the active sites of immobilized GOX to the glucose molecules. Moreover, the increase in the Km(app) value may be due to the less porous structure of the matrice. However, Km(app) values for immobilized glucose oxidase were comparable that of free enzyme.
Figure 7. The effect of glucose concentration upon the amperometric response of the biosensor (Michealis-Menten plot, in pH 7.5 phosphate buffer; operating potential is +0.4 V, 25°C).
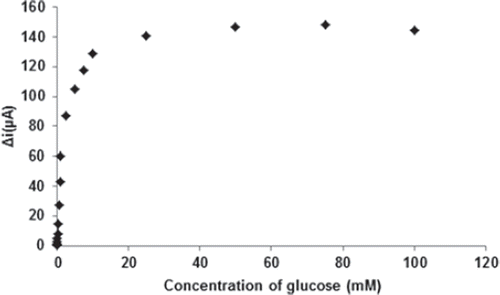
Figure 8. The calibration curves of the glucose biosensor ranging between (a) 1.0× 10-6-5×10-5M; (b) 5×10-5M-1mM (0.1 M, pH 7.5 phosphate buffer, 25°C).
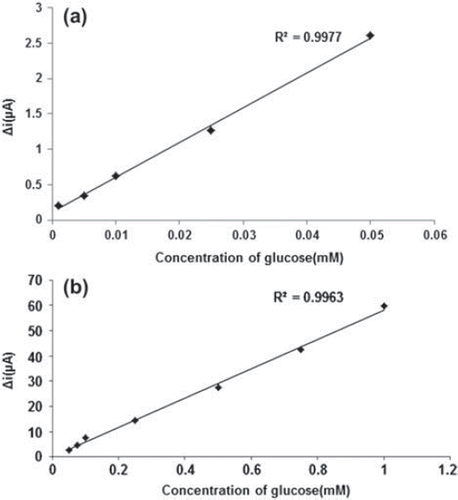
Figure 9. The effect of glucose concentration upon the amperometric response of the biosensor (Lineweaver-Burk plot, in pH 7.5 phosphate buffer and at a 0.4 V operating potential, 25°C).
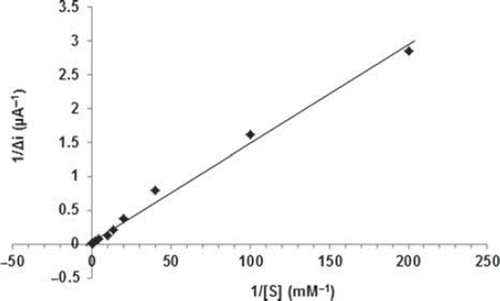
Km(app) values for immobilized glucose oxidase presented in the literature are 6.25 mM, 0.076 mM, and 0.186 mM (Gade et al. 2007a, Zhang et al. 2010, Arslan et al. 2011). The Km value of the system determines the affinity of the enzyme for its substrate, with a smaller value of Km indicating increased affinity of enzyme for its substrate. For fabrication of the biosensor, different matrices and methods of immobilization of enzymes were employed, and these could result in different conformational changes in the enzyme structures, given that the enzyme kinetics is environment-sensitive. Hence, the variation in value of Km could be attributed to these facts (Sunil et al. 2007).
Operational stability and storage stability
The stability of an enzyme is of significant importance for scheduling its application in a particular reaction. In general, an enzyme is not stable in aqueous solution during storage and the activity is gradually reduced (Hendji et al. 1993). Immobilized enzymes have enhanced stability compared to soluble enzymes, and can easily be separated from the reaction. Long-term stability is one of the significant features required for the satisfactory application of a biosensor. The biosensor was used at optimum activity conditions in 27 activity assays in one day to determine the operational stability. The response of the PPy/PVS-GOX glucose biosensor exhibited good reproducibility with a relative standard deviation (RSD) of 2.48% (). Storage stability of the biosensor was determined by performing activity assays within 93 days. As shown in , an activity loss of 37% was observed on the 93rd day. These results as to both the storage and operational stability of the sensor are extremely promising to assemble a glucose biosensor.
Effect of interferants on the response of biosensor
The effect of several possible interfering substances on the glucose biosensor based on the PPy/PVS-GOX electrode was investigated at 0.40V versus Ag/AgCl electrode in 0.10M phosphate buffer solution at pH 7.5. Several interferants, such as uric acid, ascorbic acid, paracetamol, cysteine, dopamine, and choline, were tested to study the relative response of the PPy/PVS-GOX electrode. The concentration of glucose and interferants were determined in terms of their physiological levels. The influence of the interfering compound, reported in as deviation of response percentage for a glucose concentration of 5.0 mM, was calculated from (iG + I−iG)/ iG + I × 100, where iG + I and iG were the steady-state current recorded for solutions containing glucose and interferant or glucose alone, respectively. shows that uric acid, ascorbic acid, and paracetamol increased the response of the biosensor by 2–4%, while others had no effect.
Table II. Effect of potential interferants on the glucose biosensor.
Determination of glucose in human serum
The determination of glucose in the serum sample was performed on the PPy/PVS-GOX electrode utilizing a standard addition method. Five serum samples obtained from hospitalized patients were analyzed. The concentration of the glucose in the standard solutions corresponded to 1:15 diluted serum samples. All concentrations of glucose in detection solutions were in the linear response range. After the current response was determined in the 9.0 mL 0.10 M pH 7.5 phosphate buffer and 1.0 mL of 0.1 M sodium perchlorate solution containing sample of 50 µL, a certain volume of glucose solutions of the stock glucose solution were successively added to the cell for the standard addition method. The data points obtained with the PPy/PVS-GOX electrode were the averages of the three measurements. The results were matched with referenced values obtained by the automated standard colorimetric-enzymatic technique in the hospital. shows the comparison of the determined values and the referenced values. The results obtained with the biosensors showed a good correlation with those obtained by the reference method and allowed us to ascertain the practical applicability of the proposed biosensor.
Table III. Determination of glucose in blood serum samples.
Conclusion
In this work, glucose oxidase was successfully immobilized onto poly(pyrrole-polyvinyl sulphonate) (PPy-PVS) composite film. The experimental results clearly showed that the biosensor exhibited perfect performance for the determination of glucose. It was seen that the glucose biosensor was highly sensitive and selective and its operational stability and long-term storage stability were found to be good. This composite (PPy-PVS) offered better points for developing an efficient chemical bond with enzymes, which is very helpful for the prevention of enzyme leakage and responsible for good operational stability. In addition, PPy-PVS could supply a biocompatible and electrochemical microenvironment for immobilization of the enzyme, making this material a good candidate for the fabrication of highly sensitive and selective glucose biosensors.
Acknowledgements
We acknowledge the support of this project by Gazi University Research Fund (FEF 05/2011 - 33).
Declaration of interest
The authors report no conflicts of interest. The authors alone are responsible for the content and writing of the paper.
References
- Adlan MA, Bondugulapati LN, Premawardhana LD. 2010. Glucose intolerance and diabetes mellitus in endocrine disorders – two case reports and a review. Curr Diabetes Rev. 6(5):266–73.
- Aljane F, Toumi I, Ferchichi A. 2007. HPLC determination of sugars and atomic absorption analysis of mineral salts in fresh figs of Tunisian cultivars. African Journal of Biotechnology 6(5):599–602.
- Arora K, Prabhakar N, Chand S, Malhotra BD. 2007. Immobilization of single stranded DNA probe onto polypyrrole-polyvinyl sulfonate for application to DNA hybridization biosensor. Sensors and Actuators B 126:655–663.
- Arslan F, Ustabaş S, Arslan H. 2011. An amperometric biosensor for glucose determination prepared from glucose oxidase immobilized in polyaniline-polyvinylsulfonate film. Sensors 11: 8152–8163.
- Arslan F, Yasar A, Kılıç E. 2006. An amperometric biosensor for xanthine determination prepared from xanthine oxidase immobilized in polypyrrole film. Artif. Cell Blood Subs. 34:1–16.
- Bankar SB, Bule MV, Singhal RS, Ananthanarayan L. 2009. Glucose oxidase: An overview. Biotechnology Advances 27:489–501.
- Çete S, Yaşar A, Arslan F. 2006. An amperometric biosensor for uric acid determination prepared from uricase immobilized in polypyrrole film. Artificial Cells, Blood Substitutes and Biotechnology 34:367–380.
- Chaubey A, Gerard M, Singhal M, Singh VS, Malhotra BD. 2000. Immobilization of lactate dehydrogenase on electrochemically prepared polypyrrole–polyvinylsulphonate composite films for application to lactate biosensors. Electrochimica Acta. 46:723–729.
- Collings AF, Caruso F. 1997. Biosensors: Recent advances. Reports on Progress in Physics 60:1397–1445.
- Crouch E, Cowell DC, Hoskins S, Pittson RW, Hart JP. 2005. A novel, disposable, screen-printed amperometric biosensor for glucose in serum fabricated using a water-based carbon ink. Biosensors and Bioelectronics 21:712–718.
- Dohnal L, Kalousová M, Zima T. 2010. Comparison of three methods for determination of glucose. Prague Medical Report 111(1):42–54.
- Gade VK, Shirale DJ, Gaikwad PD, Savale PA, Kakde KP, Kharat HJ, Shirsat MD. 2006. Immobilization of GOD on electrochemically synthesized Ppy–PVS composite film by cross-linking via glutaraldehyde for determination of glucose. Reactive & Functional Polymers 66:1420–1426.
- Gade VK, Shirale DJ, Gaikwad PD, Savale PA, Kakde KP, Kharat HJ, Shirsat MD. 2007a. Immobilization of GOD on Ppy-PVS composite film for determination of glucose: A comparative study of phosphate and acetate buffers. International Journal of Polymeric Materials 56:1051–1065.
- Gade VK, Shirale DJ, Gaikwad PD, Kakde KP, Savale PA, Kharat HJ, Shirsat MD. 2007b. Synthesis and characterization of Ppy-PVS, Ppy-pTS,and Ppy-DBS composite films. International Journal of Polymeric Materials 56:107–114.
- Gade VK, Shirale DJ, Gaikwad PD, Kakde KP, Savale PA, Kharat HJ, Pawar BH, Shirsat MD. 2007c. Synthesis and characterization of Ppy-PVS, P(NMP)-PVS and their co-polymer Ppy-P(NMP)-PVS films by galvanostatic method. Int. J. Electrochem. Sci. 2:270–277.
- Gerard M, Chaubey A, Malhotra BD. 2002. Application of conducting polymers to biosensors. Biosensors & Bioelectronics 17:345–359.
- Gouda MD, Kumar MA, Thakur MS, Karanth NG. 2002. Enhancement of operational stability of an enzyme biosensor for glucose and sucrose using protein based stabilizing agents. Biosensors and Bioelectronics 17:503–507.
- Hendji AN, Bataillard P, Jaffrezic-Renault N. 1993. Covalent immobilization of glucose oxidase on silanized platinum microelectrode for the monitoring of glucose. Sens. Actuators B: Chem. 15:127–134.
- Hodak J, Etchenique R, Calvo EJ, Singhal K, Bartlett PN. 1997. Layer-by-layer self-assembly of glucose oxidase with a poly(allylamine)ferrocene redox mediator. Langmuir 13:2708–2716.
- Karyakin AA. 2001. Prussian blue based nano-electrode arrays for detection of H2O2 with advanced analytical performances. Electroanalysis 13:813–819.
- Li J, Lin X. 2007. Glucose biosensor based on immobilization of glucose oxidase in poly(o-aminophenol) film on polypyrrole-Pt nanocomposite modified glassy carbon electrode. Biosensors and Bioelectronics 22:2898–2905.
- Malik B, Cote GL. 2010. Characterizing dual wavelength polarimetry through the eye for monitoring glucose. Biomedical Optics Express 1(5):1247–1258.
- Newman JD, White SF, Tothill IE, Tumer APF. 1995. Catalytic materials, membranes, and fabrication technologies suitable for the construction of amperometric biosensors. Anal. Chem. 67:4594–4599.
- Rahman MM, Ahammad AJS, Jin J-H, Ahn SJ, Lee J-J. 2010. A comprehensive review of glucose biosensors based on nanostructured metal-oxides. Sensors 10:4855–4886.
- Rauf S, Ihsan A, Akhtar K, Ghauri MA, Rahman M, Anwar MA, Khalid AM. 2006. Glucose oxidase immobilization on a novel cellulose acetate–polymethylmethacrylate membrane. Journal of Biotechnology 121:351–360.
- Retam RJ, Cabarcos EL, Mecerreyes D, Lopez-Ruiz B. 2004. Design of an amperometric biosensor using polypyrrole-microgel composites containing glucose oxidase. Biosensors and Bioelectronics 20:1111–1117.
- Sesta G. 2006. Determination of sugars in royal jelly by HPLC. Apidologie 37:84–90.
- Shankaran DR, Uehara N, Kato T. 2008. Metal dispersed sol-gel biocomposite amperometric glucose biosensor. Biosensors and Bioelectronics 18:721–728.
- Shi R, Stein K, Schwedt G. 1997. Spectrophotometric determination of glucose in foods by flow injection analysis with an immobilized glucose oxidase reactor. Z Lebensm Unters Forsch A 204:99–102.
- Singh S, Chaubey A, Malhotra BD. 2004. Preparation and characterization of an enzyme electrode based on cholesterol esterase and cholesterol oxidase immobilized onto conducting polypyrrole films. J. Appl. Polym. Sci. 91:3769–3773.
- Singh M, Kathuroju PV, Jampana N. 2009. Polypyrrole based amperometric glucose biosensors. Sensors and Actuators B 143:430–443.
- Singh S, Solanki KP, Pandey MK, Malhotra BD. 2006. Cholesterol biosensor based on cholesterol esterase, cholesterol oxidase and peroxidase immobilized onto conducting polyaniline films. Sensors and Actuators B 115:534–541.
- Steegmans M, Iliaens S, Hoebregs H. 2004. Enzymatic, spectrophotometric determination of glucose, fructose, sucrose, and inulin/ oligofructose in foods. J AOAC Int. 87(5):1200–1207.
- Sunil K, Arya AB, Arun K, Prusty SP, Singh A, Pratima R, Solanki KP, Manoj M, Malhotra BD. 2007. Cholesterol biosensor based on N-(2-aminoethyl)-3-aminopropyl-trimethoxysilane self-assembled monolayer. Analytical Biochemistry 363:210–218.
- Tan X-C, Zhang J-L, Tan S-W, Zhao D-D, Huang Z-W, Mi Y, Huang Z-Y. 2009. Amperometric hydrogen peroxide biosensor based on immobilization of hemoglobin on a glassy carbon electrode modified with Fe3O4/chitosan core-shell microspheres. Sensors 9:6185–6199.
- Vidal JC, Espuelas J, Garcia E, Castillo JR. 2004. Amperometric cholesterol biosensors based on the electropolymerization of pyrrole and the electrocatalytic effect of Prussian-Blue layers helped with self-assembled monolayers. Talanta 64:655–664.
- Vidal JC, Garcia-Ruiz S, Mendez P, Castillo JR. 1999. Three approaches to the development of selective bilayer amperometric biosensors for glucose by in situ electropolymerization. Analyst 124:319–324.
- Wan D, Yuan S, Li GL, Neoh KG, Kang ET. 2010. Glucose biosensor from covalent mmobilization of chitosan-coupled carbon nanotubes on polyaniline-modified gold electrode. Applied Materials & Interfaces 2(11):3083–3091.
- Wang J. 2008. Electrochemical glucose biosensors. Chem. Rev. 2008108: 814–825.
- Wang X, Zhang Y, Cheng C, Dong R, Hao J. 2011. Glucose in human serum determined by capillary electrophoresis with glucose micro-biosensor. Analyst. 136(8):1753–1759.
- Wanga H, Ohnukia H, Endob H, Izumia M. 2011. Preparation of amperometric glucose biosensor based on 4-mercaptobenzoic acid. Physics Procedia 14:2–6.
- Witt S, Wohlfahrt G, Schomburg D, Hecht H, Kalisz H. 2000. Conserved arginine-516 of Penicillium amagasakiense glucose oxidase is essential for the efficient binding of β-D-glucose. J. Biochem. 347:553–9.
- Yıldırımoğlu F, Arslan F, Çete S, Yas ar A. 2009. Preparation of a polypyrrole polyvinylsulphonate composite film biosensor for determination of cholesterol based on entrapment of cholesterol oxidase. Sensors 9:6435–6445.
- Yıldız HB, Kiralp S, Toppare L, Yagc Y. 2005. Immobilization of glucose oxidase in conducting graft copolymers and determination of glucose amount in orange juices with enzyme electrodes. International Journal of Biological Macromolecules 37:174–178.
- Zhang Y, Guo G, Zhao F, Mo Z, Xiao F, Zeng B. 2010. A novel glucose biosensor based on glucose oxidase immobilized on AuPt nanoparticle-carbon nanotube-ionic liquid hybrid coated electrode. Electroanalysis 22:223–228.
- Zhang Y, Wen G, Zhou Y, Shuang S, Dong C, Choi MM. 2007. Development and analytical application of an uric acid biosensor using an uricase-immobilized eggshell membrane. Biosens. Bioelectron. 22:1791–1797.