Abstract
Context: Estrogen is well-known as an important factor in the physiological functions and pathological processes of breast. Estrogen receptor β (ERβ) is expressed in the majority of breast cancers at lower levels compared with the normal breast tissue.
Objective: The effect of ERβ on the characteristics of breast tumor cells and its prognostication for the use of ERβ-selective therapy were investigated here for the first time.
Materials and methods: ERβ was overexpressed in ERα positive MCF-7 breast cancer cells by gene transfection. The proliferation, motility, and xenografts growth of MCF-7 cells were investigated by MTT assays, wound-healing assay and animal study.
Results: Results demonstrated that ERβ-GFP localized in both the cytoplasm and the nucleus in the presence of 17β-estradiol (E2), with stronger fluorescence-signal intensity in the nucleus, 2.8-times higher than that in the cytoplasm. The ERβ overexpressed MCF-7 cells resulted in a 38.7% decreased growth rate and motility in vitro. Furthermore, ERβ overexpression enhanced the antiproliferative effects of phytoestrogen, antiestrogen, and histone deacetylase inhibitor. Exogenous ERβ expression reduced tumor volume by 99% at 27 days postadministration, indicated that overexpression of ERβ led to retardation of tumor formation and growth in immunodeficient mice.
Discussion and conclusion: This study provided a relatively new evidence to support that ERβ is an important modulator of proliferation and motility of breast cancer cells, and implied for the first time a possibility for the use of novel ERβ-selective therapies in breast cancer treatment.
Introduction
Estrogen is well-known as a key regulator of growth and differentiation in a broad range of target tissues, including the reproductive tract, mammary gland, and the central nervous and skeletal systems (CitationPettersson & Gustafsson, 2001). It is also known to be involved in many pathological processes such as breast and endometrial cancer (CitationHenderson et al., 1988). Estrogen receptor (ER) α remains the most important biomarker in breast cancer as it indicates the likelihood of patients to benefit from endocrine therapy. Although ERα status has been established as an important biomarker in breast cancer (CitationAli & Coombes, 2002), the discovery of a second estrogen receptor ERβ in 1996 (CitationKuiper et al., 1996; CitationMosselman et al., 1996) added a new dimension to the role of estrogen in various pathologic processes. These ERs share a high degree of primary structure similarity, but they are encoded on different chromosomes. They also have diverse expression patterns and distinct phenotypes as shown in ERα and ERβ knockout (αERKO and βERKO) mice (CitationDeroo & Korach, 2006). Thus, these ERs have different transcriptional activities with certain ligands, cell-types, and promoter contexts, which explain some of the major differences in their tissue-specific biological actions (CitationZhao et al., 2008).
ERβ is expressed in both normal and malignant human breast tissue (CitationEnmark et al., 1997; CitationMoore et al., 1998) although its role is unclear. In contrast to ERα, published data suggested that ERβ expression declines with the breast tumorigenesis (CitationBardin et al., 2004; Leygue et al., 1997; CitationRoger et al., 2001). This downregulation of ERβ in carcinogenesis and tumor progression suggests that it is a tumor suppressor. CitationNovelli et al. (2008) conducted a prospective immunohistochemical study of ERβ in 936 breast cancers. The study showed that positive expression of ERβ was associated with more aggressive breast cancer phenotypes, namely human epidermal growth factor receptor-2 (HER2)-positive tumors and ERα-negative/progesterone receptor (PR)-negative/HER2-negative (triple negative) tumors. This relationship has also been observed by others (CitationHonma et al., 2008; CitationSkliris et al., 2006). Thus, ERβ pathway may be a therapeutic target in these tumors. In addition, there is no correlation between ERβ expression and conventional prognosticators (CitationHonma et al., 2008; CitationNovelli et al., 2008). Therefore, ERβ may become an independent prognosticator. CitationHonma et al. (2008) reported that ERβ was an independent predictor for recurrence, disease-free survival and overall survival in 442 cases treated with adjuvant tamoxifen therapy. More important, ERβ positivity was associated with significantly better survival in patients with ERα-negative/PR-negative or triple-negative tumors. These tumors are widely believed to be hormone unresponsive, with poor prognosis. Furthermore, CitationGruvberger-Saal et al. (2007) showed that, in the ERα-negative subgroup, ERβ expression was associated with longer disease-free survival on tamoxifen treatment. Finally, CitationShaaban et al. (2008) reported similar results of ERβ by immunohistochemical study in 757 breast tumors. Thus, in addition to ERα and PR status, immunohistochemical examination of ERβ is clinically important in patients with breast cancer treated with tamoxifen monotherapy. ERβ may become a potential biomarker for endocrine response.
Uncovering the contribution of ERβ in estrogenic regulation of breast tumor growth is important for understanding the mechanism and treatment of breast cancer. The majority of clinical breast tumors are positive for both ERα and ERβ (CitationHonma et al., 2008; CitationNovelli et al., 2008) and the expression of ERβ in breast tumors is known to be epigenetically silenced or downregulated by both deacetylation of histone tails and gene methylation (CitationDuong et al., 2006; CitationRody et al., 2005; CitationZhao et al., 2003). Therefore, we chose MCF-7 breast cancer cell line for this investigation to mimic the clinical scenario because it is positive in both ERα and ERβ with lower expression of ERβ. To evaluate the role of ERβ in the growth, motility and tumorigenesis of breast cancer cells in vitro and in vivo, a stable overexpression of ERβ was established in the ERα-positive MCF-7 breast cancer cell line in this study. The enhanced green fluorescent protein (EGFP) was C-terminally tagged to ERβ for visualizing the expression, function, and location of ERβ in the tumor cells. The characteristics of ERβ-transfected MCF-7 cells, including proliferation, motility, and ability to grow in immunodeficient mice were investigated. We also investigated if the overexpression of ERβ will enhance the antiproliferative efficacy of antiestrogen, phytoestrogen, and histone deacetylase inhibitor.
Methods
Plasmids and chemicals
Plasmid pCMV5-hERβ was provided by Dr. Guowei Gu (Calabria, Italy). Plasmid pEGFP-C3 was provided by Professor Tao Xi (Nanjing, China). Trizol reagent, G418 and DNA Marker-B were purchased from Bio Basic Inc. 17β-Estradiol was purchased from Sigma-Aldrich (Shanghai, China). M-MLV Reverse Transcriptase and Taq DNA polymerase were purchased from Promega (Shanghai, China). Methyl thiazolyl tetrazolium (MTT) and other reagents for cell cultivation were all purchased from Gibco (Life Technologies, Shanghai, China). Sofast™ Transfection Reagent was purchased from Sunma Biotech Corp. (Xiamen, China). Primers for RT-PCR assay were synthesized by Shanghai Sangon Biological Engineering Technology & Services Co., Ltd. (Shanghai, China). Other chemical reagents used in the study were certified analytical-reagent grade (Shanghai Chemical Reagent Company, Shanghai, China).
Plasmids transfection
The short form of ERβ encoding the COOH-terminal 485 amino acids was cloned into pEGFP-C3 to create pEGFP-ERβ, ensuring that the C terminus of the GFP was fused to the N terminus of the human ERβ. We did not use the long form of ERβ (530 amino acids) because it is lethal to the cells (CitationHartman et al., 2006). Human breast carcinoma cell line MCF-7 cells are well-known to be the best characterized ERα-positive cell line in terms of known genes regulated by estrogens that promote proliferation. MCF-7 cells used in these experiments expressed ERβ at very low level, which was confirmed in the next experiment. MCF-7 was purchased from Cell Bank of Shanghai Institute of Biochemistry & Cell Biology, Chinese Academy of Sciences (Shanghai, China) and maintained in RMPI 1640 media with 10% newborn bovine serum (NBS). Cells were cultured with 5% CO2 at 37°C in a humidified incubator. For stable transfection of pEGFP-ERβ, MCF-7 cells were seeded in six well plates with 3.0 × 104 cells/well. After 24 h, the pEGFP-ERβ construct was transfected into MCF-7 cell using Sofast™ Transfection Reagent according to the manufacturer’s instruction. The selection of transfected cells with 500 µg/mL G418 was started 48 h after transfection and then screened to obtainy limiting dilution analysis. For antibiotic selection and screen by limiting dilution analysis, positive cells could be identified by detecting green fluorescence. After 4 weeks, stably ERβ-transfected MCF-7 cells (MCF-7-ERβ) were maintained. Meanwhile, a control cell line was established by transfecting an empty expression plasmid pEGFP-C3 (MCF-7-GFP).
Characterization of expression and activity of ERβ by RNA extraction and RT-PCR
For ligand stimulation, MCF-7-ERβ and MCF-7-GFP cells were maintained in phenol-red free RPMI 1640 containing 10% dextran-coated charcoal-treated newborn bovine serum (DCC-NBS) for 10 days and treated with control vehicle ethanol or 10−9 M E2 (equal affinity for ERα and ERβ) for 24 h. Total RNA of 106 cells was isolated from MCF-7 cells using the TRIzol reagent as described by the manufacturer. From 1 μg total RNA, cDNA was synthesized using the M-MLV Reverse Transcriptase and oligo(dT)16 primer in a 20 µl reaction volume. For detection of mRNA by standard RT-PCR, 1 µl cDNA was amplified in a 25 µl reaction mix of 1.5 U Taq DNA polymerase, 10 pmol of each primer, 1 × PCR buffer, 1.5 mM MgCl2 and 0.4 mM of each dNTP. Primers used to amplify ERβ were 5′-TAGTGGTCCATGCCAGTTAT-3′ (position 266–286) and 5′-GGGAGCCACACTTCACCAT-3′ (position 640-658, NCBI Accession No. NM 001437.2, X99101), giving a PCR product of 393 bp. For Cathepsin D, primer sequences were 5′-CTCTGTGGAGGACCTGATTGC-3′ (position 123–143) and 5′-AGGCTGACGACGCTGACTGG-3′ (position 480-499, NCBI Accession No. NM 001909.3), giving a PCR product of 377 bp. For the reference gene β-actin, primer sequences were 5′-CTGTGGCATCCACGAAACTA-3′ (position 813–832) and 5′-CGCTCAGGAGGAGCAATG-3′ (position 987–1004, NCBI Accession No. NM 001101.3), giving a PCR product of 192 bp. The PCR program was 95°C for 5 min, followed by 32 PCR cycles (94°C for 40 s, 58°C for 30 s, 72°C for 30 s) and a final extension for 5 min at 72°C. PCR products (4 µl) were analyzed by electrophoresis through a 1% agarose gel and visualized by GoldView staining under UV illumination.
Cell-proliferation study
Cells were maintained for 10 days in phenol-red free RPMI 1640 containing 10% DCC-NBS and then seeded in 96 well plates with 1,000 cells/well in 10% DCC-NBS phenol-red free RPMI 1640. Treatment with control vehicle ethanol or 10−9 M E2 began at the same time. After 0, 2, 3, 4, and 5 days, relative numbers of viable cells were determined by MTT assay. Cell growth was expressed as a percentage of the value at day 0.
To test if the ERβ-transfected MCF-7 cells were functional, the effects induced by different ligands such as phytoestrogen genistein, antiestrogen raloxifene, and histone deacetylase inhibitor (HDACi) trichostatin A (TSA) were analyzed. The cells were plated in depleted medium at 500 cells/well into 96-well plates. After 3 days, the cells were exposed for 72 h to the indicated concentrations of the chemicals. To analyze the proliferation of breast cancer cells, MTT assay was used according to the manufacturer’s instruction.
Motility study by wound-healing assay
Cells were seeded in 6 well plates (3 × 105 cells/well) in phenol-red free RPMI 1640 containing 10% DCC-NBS. Twenty-four hours after plating, ethanol or 10−9 M E2 treatment was started. After 24 h of treatment, wound-induced migration was triggered by scraping the cells with a blue tip, and the scratch was imaged immediately (0 h). The cells were imaged again 48 and 72 h after the scratch. The percentage of wound filling (healing) was calculated by measuring the remaining unfilled space (gap) on the images. The ratio of the gap at 48 and 72 h over the gap at 0 h was used to determine the percentage of wound remaining.
Experimental animals and xenograft model
The female athymic BALB/c-nu nude mice used in this study were 4–6 weeks old and weight of about 18–22 g. They were purchased from Charles River Laboratories (Shanghai, China). MCF-7-ERβ and MCF-7-GFP cells (3 × 106) suspended in 200 μL normal medium were transplanted subcutaneously into the right and left armpit respectively of 6 BALB/c-nu nude mice without matrigel. Animals were treated with 0.5 mg/kg E2 (i.m.) one day before transplantation and once a week after transplantation to stimulate the growth of MCF-7 tumors. Tumor diameters were measured twice weekly. Tumor volumes were calculated according to the equation (width2 × length)/2. All animal studies were conducted in accordance with the principles and procedures outlined in the Animal Management Rules of the Ministry of Health of the People’s Republic of China (document NO.55 5001) and the guidelines for the Care and Use of Laboratory Animals under an approved animal protocol of China Pharmaceutical University. After 4 weeks, the mice were sacrificed and all tumors were separated, fixed in 4% paraformaldehyde, and stored in 75% ethanol for pathological examination by H&E staining.
Statistical analysis
The results are expressed as means ± SD with 95% confidence intervals. Tumor-growth curves were constructed from the mean tumor volume at each time point of measurement. One-way ANOVA analysis was used to compare differences between groups. Significance was presented as *p < 0.05; **p < 0.01; ***p < 0.001; and NS, nonsignificant.
Results
Expression and location of ERβ in MCF-7 cells
The EGFP was C-terminally tagged to human ERβ for observing the expression and location of ERβ in MCF-7 cells. The screened monoclone-transfected cells were observed under a microscope. shows the reflected light imaging (A, C) and fluorescence imaging (B, D) 4 weeks after transfection. and are the cells transfected with only GFP for control. and are the cells transfected with GFP-ERβ. The bright fluorescence signals in both GFP and GFP-ERβ-transfected cells indicated the successfully stable gene transfection, which was further identified by RT-PCR in later experiments. The good correlation between the reflected light imaging and fluorescence imaging confirmed the successful monoclone screening. Compared with the cell profiles in the reflected light imaging, the fluorescence signals were observed to be localized both in the cytoplasm and the nucleus. Because the activated ERβ could translocate into the nucleus, most of the GFP signal is centered in nucleus in the GFP-ERβ-transfected cells ( compared with the control GFP-transfected cells, which is uniformly distributed over the whole cells (. The fluorescence intensity in the nucleus of GFP-ERβ-transfected cells is 2.8 times than that in the cytoplasm. The translocation confirmed the functionality of the ERβ-GFP protein.
Figure 1. Expression and location of ERβ-GFP fusion protein in MCF-7 cells in vitro under fluorescence microscope. (A) reflected light micrograph of MCF-7-GFP Cells. (B) corresponding fluorescence micrograph of MCF-7-GFP Cells. (C) reflected light micrograph of MCF-7-ERβ Cells. (D) corresponding fluorescence micrograph of MCF-7-ERβ Cells. The C terminus of the GFP was fused to the N terminus of the human ERβ. ERβ-GFP fusion protein stably expressed in ERβ-transfected MCF-7 cells localized both in the cytoplasm and the nucleus, with stronger GFP signal in the nucleus. (Original magnification ×400). (E) relatively numerical values of mean fluorescence intensity in nucleus and in cytoplasm in ERβ-transfected MCF-7 cells compared with that of background. Data were given as mean ± SD. **p < 0.01 versus corresponding mean fluorescence intensity in cytoplasm.
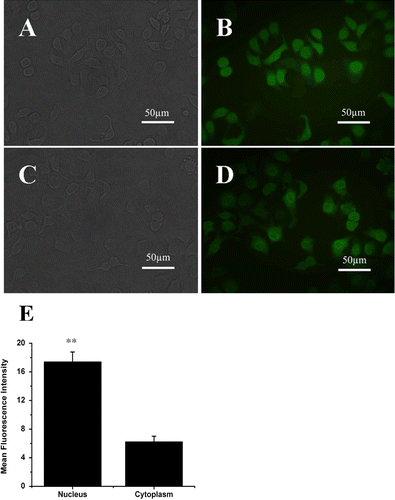
Relative mRNA expression and functionality of ERβ
ERβ expression of MCF-7-ERβ cells was examined by RT-PCR (32 cycles). The gene expression of ERβ mRNA in relative to β-actin levels was determined by GoldView staining of RT-PCR products. shows the expression levels of ERβ in different cell types including MCF-7 wild type, MCF-7-GFP, and MCF-7-ERβ, with or without E2 treatment. A weak expression of ERβ was observed in wild type, which confirmed the low expression of ERβ in this breast cancer cell line. MCF-7-GFP cells displayed similar weak signal, indicating that the GFP transfection did not significantly alter the ERβ basal expression. As expected, a high expression of ERβ mRNA could be detected in MCF-7-ERβ cells (P < 0.01). The semiquantitative analysis of the expression levels is depicted in . With 10−9 M E2 treatment, the expression levels of ERβ mRNA in wild type, MCF-7-GFP, and MCF-7-ERβ cells were all higher than that in the corresponding cell types without E2 treatment (p < 0.05).
Figure 2. Expression of ERβ and cathepsin D mRNA. (A) MCF-7 cells were stably transfected with pEGFP-C3 or pEGFP-hERβ. After 24 h of treatment with 10−9 M E2 (+E2) or control vehicle ethanol (−E2), ERβ and CTSD expressions were detected by RT-PCR with β-actin as the reference gene. The PCR products have a size of 393 and 377 bp for ERβ and CTSD, respectively. (Marker. DNA Marker-B; wild type. Nontransfected MCF-7 cells; GFP. Cells stably transfected with pEGFP-C3; ERβ. Cells stably transfected with pEGFP-hERβ). (B) Semiquantitative calculation of relative mRNA expression of ERβ normalized to β-Actin levels. (C) Semiquantitative calculation of relative mRNA expression of CTSD normalized to β-Actin levels. Each experiment was performed three times independently and results were expressed as means ± SD. ** p ≤ 0.01, ***p ≤ 0.001 versus corresponding wild type.
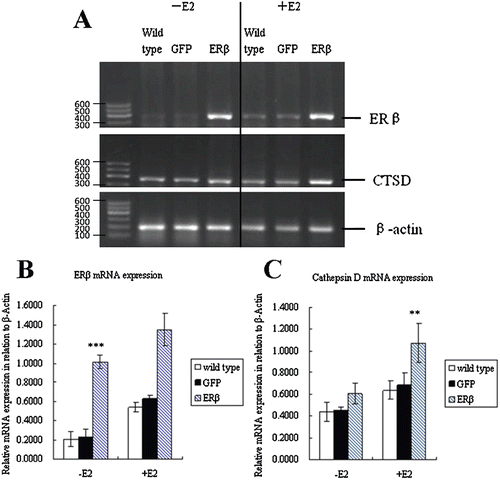
To further assess the functionality of the expressed ERβ-GFP protein, we examined the modulation ability of ERβ to the expression of gene cathepsin D (CTSD). CTSD is a well-known estrogen-related gene and the results are shown in and . These data demonstrated that ERβ has the ability to upregulate the expression of cathepsin D (p < 0.05). This implied that ERβ expressed in MCF-7-ERβ cells is transcriptionally active and GFP tagging did not affect the biological function of ERβ. In addition, cathepsin D levels in ERβ transfectants, wild-type cells and GFP transfectants were additionally elevated after treatment with E2 (p < 0.05). Thus, the expression of cathepsin D could be modulated partially by ERβ in a ligand-dependent manner. A previous study demonstrated that the promoter of cathepsin D contains an estrogen response element (ERE) for transcriptional activation through an ERα-transcription complex (CitationXing & Archer, 1998). Our results clearly suggested that cathepsin D is a shared genetic target of both ERα and ERβ.
Inhibition of cell growth by ERβ
Given that ERs are known to regulate cellular proliferation in different molecular mechanisms, we examined the effect of ERβ expression on cellular proliferation of MCF-7 cells by MTT assay. As shown in , control cells (wild-type cells and MCF-7-GFP cells) had a similar growth pattern in the presence or absence of E2. MCF-7-ERβ cells showed a significantly slower rise in cell viability than wild type or MCF-7-GFP cells. The expression of ERβ resulted in a 38.7% reduction in cell proliferation of MCF-7 cells in the absence of E2 compared with wild-type cells and MCF-7-GFP cells at day 5. Addition of E2 augmented the inhibition of cell proliferation to 57.1% in MCF-7-ERβ cells at day 5 compared with cells without E2. These data confirm that ERβ is a negative modulator of cell growth to ERα-positive MCF-7 breast cancer cell. In addition, E2 treatment enhanced the inhibition of proliferation, which indicates that the modulation of ERβ to cell growth is also partially ligand dependent.
Figure 3. Cell growth of MCF-7 cells stably expressing GFP or ERβ. MCF-7 cells were stably transfected with pEGFP-C3 or pEGFP-hERβ. Wild type and transfected MCF-7 cells overexpressing GFP or ERβ were cultured up to 5 days in serum-free medium in the presence of 10−9 M E2 (+E2) or control vehicle ethanol (−E2). Relative viable cell numbers were determined by MTT assay on day 0, 2, 3, 4, and 5. Viable cell numbers are expressed as percentage relative to day 0. Each experiment was performed three times independently and results were expressed as means ± SD. *p ≤ 0.05, ***p ≤ 0.001 versus corresponding wild type.
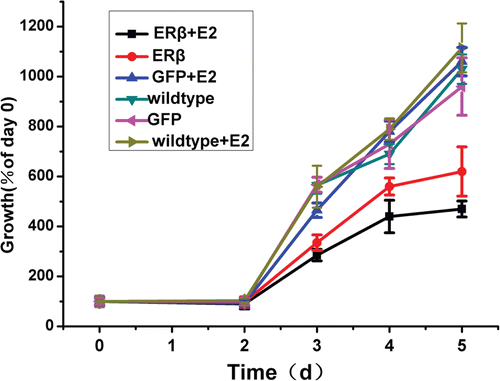
ERβ enhances the antiproliferative effects of genistein, raloxifene, and trichostatin A
As the presence of ERβ in mammary tumors has been shown to correlate with increased disease-free survival in patients, we treated MCF7-ERβ cells with genistein, a modest selective ERβ agonist, whose binding activity is 20-fold stronger to ERβ than ERα. We also treated the cells with the antiestrogen drug raloxifene and the histone deacetylase inhibitor trichostatin A (TSA). MCF-7-GFP cells and wild type were used as controls. When MCF7-ERβ cells were treated with increasing concentrations of genistein, the presence of ERβ increased both the efficacy and potency of genistein (. ERβ shifted the IC50 of genistein from 48.5 µM (wild type) and 22 µM (MCF-7-GFP) to 8 µM. A similar increase in efficacy was observed for antiestrogen, raloxifene. With raloxifene treatment, ERβ decreased the IC50 from 13 µM (wild type) and 8.5 µM (MCF-7-GFP) to 4.5 µM (. Finally, we analyzed the synergistic action of TSA and ERβ. As shown in , the IC50 of TSA in MCF7-ERβ, MCF-7-GFP, and wild-type cells were 3, 40, and 36 nM, respectively.
Figure 4. ERβ enhances the antiproliferative effects of genistein, raloxifene, and TSA to inhibit breast cell proliferation. (A) genistein inhibits proliferation of MCF-7 cells to a greater extent when ERβ is expressed. MCF-7-ERβ, MCF-7-GFP, and wild-type MCF-7 cells were treated with increasing concentrations of genistein for 72 h and the relative viable cell numbers were determined by MTT assay. Each experiment was performed three times independently and results were expressed as means ± SD. Similar experiments were conducted on MCF-7-ERβ, MCF-7-GFP, and wild type MCF-7 cells treated with raloxifene (B) and TSA (C). Each experiment was performed three times independently and results were expressed as means ± SD: **p ≤ 0.05, **p ≤ 0.01 versus IC50 of corresponding agent in MCF-7 wild-type cells; #p ≤ 0.05, versus IC50 of corresponding agent in MCF-7-GFP Cells.
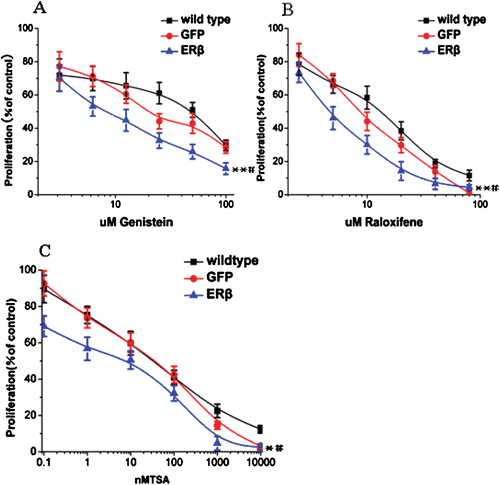
Motility of MCF-7 cells is affected by expression of ERβ
Tumor development involves not only proliferation but also invasion. Thus, the motility of ERβ-transfected cells was here investigated. The healing-induced migration experiments were performed on MCF-7-ERβ cells, with wild type and MCF-7-GFP cells as controls. Wild type and transfected cells were forced to migrate to the space created by scraping the monolayer with a tip. shows the migration of different cells at different period after scraping. After 48 h of migration, MCF-7-ERβ cells as well as wild type and MCF-7-GFP cells had filled about 55% of the wound in the absence of E2. There was no significant difference between MCF-7-ERβ and control cells. However, in the presence of E2, MCF-7-ERβ cells expressing higher levels of ERβ exhibited significantly slower migration with only about 33% of the wound filled, whereas control cells filled about 75% of the wound (p < 0.01). The same trend was maintained after 72 h of migration. This phenomenon suggested that the inhibition of motility by ERβ in MCF-7 cells is ligand dependent.
Figure 5. Motility of MCF-7 cells stably expressing GFP or ERβ. MCF-7 cells were stably transfected with pEGFP-C3 or pEGFP-hERβ. Wild-type and transfected MCF-7 cells overexpressing GFP or ERβ were cultured in serum-free medium in the presence of 10−9 M E2 (+E2) or control vehicle ethanol (−E2). After 24 h of treatment, wound-induced migration was triggered by scraping the cells with a blue tip, and the scratch was imaged immediately (0 h). The cells were imaged again at 48 h and 72 h after the scratch. Each experiment was performed three times independently. (A) images of a representative assay at 0, 48, and 72 h are shown here. (B) The percent of wound filling was calculated by measuring the remaining gap on the images. The ratio of the gap space at 48 h and 72 h over the gap space at 0 h gives the percentage of wound filling. Results were obtained from three separate experiments and are expressed as means ± SD. ***p ≤ 0.001 versus corresponding wild type.
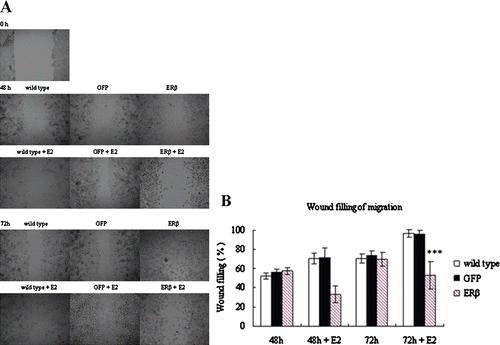
Tumor formation and growth of MCF-7 in nude mice
Finally, the effects of ERβ expression on tumor formation and growth in a BALB/c-nu nude mice xenograft model were investigated. Because MCF-7 cells are estrogen dependent, the mice were treated with 0.5 mg/kg estradiol (i.m.) one day before transplantation to stimulate tumor-cell growth. MCF-7-ERβ and MCF-7-GFP cells were transplanted subcutaneously into the right and left armpit, respectively, as described in the methods section. As shown in , ERβ expression obviously inhibited the growth of MCF-7 tumor xenografts in the mice. Exogenous ERβ expression reduced tumor volume by 99% at 27 days postadministration (. Although MCF-7-GFP inoculation resulted in 6/6 tumors formation, only 2/6 tumors developed in the MCF-7-ERβ inoculation. No MCF-7-ERβ tumor tissue could be found in four animals at necropsy. Furthermore, the two developed MCF-7-ERβ tumors did not grow larger after 15 days and even shrank with time. Thus, ERβ could inhibit tumor formation and tumor growth of MCF-7 cells in mice xenografts.
Figure 6. ERβ inhibits tumor formation and growth of MCF-7 cells in mice xenografts. 3 × 106 MCF-7-ERβ and MCF-7-GFP monoclonal cells suspended in 200 μL normal medium were transplanted subcutaneously into the right and left armpit, respectively, of six female BALB/c-nu nude mice without Matrigel. Of the six mice implanted with MCF-7-ERβ and MCF-7-GFP, MCF-7-GFP inoculation resulted in the development of 6/6 tumors, whereas MCF-7-ERβ inoculation resulted in only 2/6 tumors developing. (A) Representative image of the developed xenografts at day 15. The arrow points to the site of grafting of MCF-7 cells stably transfected with GFP or ERβ. (B) Tumor growth of the developed grafting of MCF-7 cells stably transfected with GFP or ERβ. Tumor diameters were measured twice weekly and tumor volumes were calculated according to equation (width2 × length)/2. *p ≤ 0.05, ***p ≤ 0.001 versus corresponding xenografts grafting of MCF-7 cells stably transfected with ERβ.
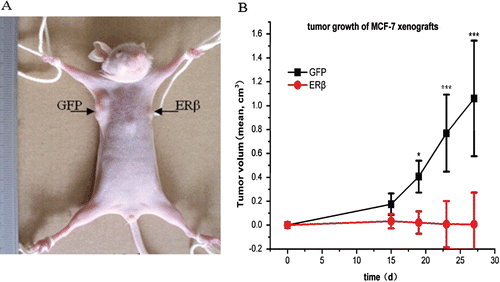
As a consequence of the inhibition of tumor formation resulting from ERβ expression, no tumor tissues of monoclonal MCF-7-ERβ cell xenografts could be obtained to perform further pathological examination. Therefore, we performed a second experiment using ERβ-transfected polyclonal MCF-7 cells. The ERβ-transfected and GFP-transfected polyclonal MCF-7 cells (2 × 106) suspended in 200 μL normal medium were transplanted subcutaneously into the right and left armpit, respectively, of six female BALB/c-nu nude mice without Matrigel. Although both MCF-7-GFP and MCF-7-ERβ inoculation resulted in 6/6 tumors developing, the growth of MCF-7-ERβ xenografts was also significantly inhibited, as determined by the tumor-volume observation (. After 4 weeks, the mice were sacrificed and all tumors were separated and fixed in 4% paraformaldehyde and stored in 75% ethanol for pathological examination. Compared with MCF-7-GFP tumors (, there were more necrosis areas in MCF-7-ERβ tumors (. The ensemble of the above results demonstrated that introducing ERβ into MCF-7 cells could prevent tumor formation and tumor growth in BALB/c-nu nude mice xenografts, suggesting that ERβ-selective therapies could become a viable alternative to current breast cancer treatment paradigms.
Figure 7. Representative image and pathological examination of xenografts of polyclonal MCF-7 cells stably transfected with GFP or ERβ. 2 × 106 ERβ-transfected and GFP-transfected polyclonal MCF-7 cells suspended in 200 μl normal medium were transplanted subcutaneously into the right and left armpit, respectively, of six female BALB/c-nu nude mice without Matrigel. (A) Representative image of xenografts at day 23. (B) Representative image of pathological examination of MCF-7-GFP xenografts by H&E stain (original magnification ×100). (C) Representative image of pathological examination of MCF-7-ERβ xenografts by H&E stain (original magnification ×100).
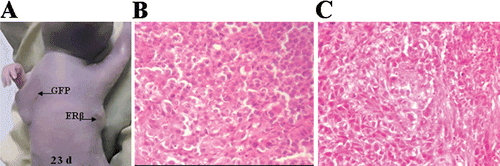
Discussion
The aim of this study was to elucidate the role of ERβ in regulating the basic features of breast cancer cells, including their proliferation, motility, and tumor growth. We selected estrogen-dependent MCF-7 cell line, which is a widely used model for hormone-dependent breast cancer expressing high levels of ERα and low levels of ERβ. In this study, we stably introduced ERβ cDNA into MCF-7 cells to characterize the functional significance and role of ERβ in this breast cancer model. To visualize the expression of ERβ, we also tagged it at the N-terminus with EGFP. Thus, the expression, function, and location of ERβ could be detected by green fluorescence signal. By this method, we found that ERβ localized in both the cytoplasm and the nucleus (. The functionality of transfected ERβ was demonstrated by the stronger GFP signal in nucleus after E2 treatment (, suggesting the receptor’s translocation from the cytoplasm into the nucleus. In addition, cathepsin D mRNA was upregulated in cells overexpressing ERβ ( and ). Stable overexpression of ERβ in MCF-7 cells inhibited the proliferation and motility of MCF-7 cells in vitro ( and ). Furthermore, the overexpression of ERβ prevented tumor formation and growth in vivo ().
In general, the exposure of MCF-7 cells to 17β-estradiol promotes cell proliferation and tumor growth (CitationGottardis et al., 1988). In this study, the MCF-7 cells expressing high level of ERβ showed about 38.7% decrement in proliferation compared with wild-type and MCF-7-GFP cells even in absence of E2. The growth of MCF-7 cells overexpressing ERβ was further reduced by E2, demonstrating a ligand-dependent action of this receptor in our cell model. This effect could be attributed to the ability of ERβ-modulating gene regulation by both ligand-dependent and ligand-independent mechanisms (CitationWilliams et al., 2008).
It is known (CitationRochefort et al., 1998) that E2 regulates many genes involved in cell adhesion, which plays a significant role in the motility of cells or the capability of tumors to metastasize. The potential modulation of ERβ on cell motility was investigated in this study. We indeed observed that ERβ significantly inhibited the motility of the MCF-7 breast cancer cells. This finding is consistent with the previous observation in ERα-negative MDA-MB-231 breast cancer model exogenously expressing ERβ by CitationLazennec et al. (2001). In addition, we found that the effect of ERβ on cell motility was estrogen dependent, which was different from the estrogen-independent effect observed in MDA-MB-231 cells. The molecular mechanism underlying the decreased motility of these cells by ERβ is not clear at the present time. Possibly, ERβ increases integrin expression and clustering, and consequently enhances adhesion and decreases migration of breast cancer cells (CitationLindberg et al., 2010). The decreased motility of ERβ overexpressed MCF-7 cells observed in this study supported the above hypothesis.
Interestingly, stably ERβ-transfected MCF-7 cells do not overlap and grow above or below each other (data not shown). As soon as the cells are confluent, some of the cells will float and die. However, wild type and stably GFP-transfected MCF-7 cells could continue to grow above or below each other. Usually, the contact inhibition and ibition in malignant cells are lost or decreased. Thus, malignant cells could overlap and grow above or under each other. Based on this phenomenon, we speculated that the expression of ERβ could allow MCF-7 cells to recover some characteristics of nonmalignant cells. This hypothesis needs further study.
Phytoestrogens are naturally occurring estrogens (CitationPeng et al., 2009). These estrogens have been shown to stimulate proliferation of breast cancer in xenograft models, and the stimulatory effects to MCF-7 breast cancer cells were observed after genistein treatment (CitationAllred et al., 2001; CitationHsieh et al., 1998). In another study performed with chemically induced endogenous tumors in rats, inhibitory effects of phytoestrogen have been observed (CitationMessina & Barnes, 1991). One explanation for these inconsistent findings is that the antiproliferative and tumor-suppressive effects of some phytoestrogens are mediated by ERβ, whereas ERα stimulates proliferation. In this study, we observed a synergistically inhibitory effect of genistein and ERβ in MCF-7 cells, which is in accordance with the hypothesis concluded by Johan CitationHartman et al. (2009). The antiestrogen tamoxifen has been reported to enhance the antiproliferative effect of ERβ in breast cancer (CitationHodges-Gallagher et al., 2008). Here, we observed another antiestrogen raloxifene, which have been reported to preferentially activate genes modulated by ERβ (CitationLevy et al., 2008) to enhance the antiproliferative effects of ERβ dramatically.
We next explored the effect of overexpressed ERβ on tumor formation of xenograft model of nude mice. ERβ expression obviously inhibited the formation and growth of MCF-7 cells as tumor xenografts in immunodeficient mice. As shown by other studies using viral-transfection systems, ERβ inhibits tumor formation of MCF-7 cells with a positive relationship between the virus-mediated multiplicity of infection of ERβ and the loss of tumor-formation capacity in a mouse model (CitationParuthiyil et al., 2004). The major critique of viral-transfection studies is that uncontrolled overexpression of the transfected gene leads to nonphysiologically high protein levels. Our stable transfection of ERβ overcomes the disadvantage of viral transfection and provides useful data to study the role of ERβ. One reason of the tumor suppression by ERβ may be due to the inhibition of cell proliferation by ERβ (CitationBehrens et al., 2007; CitationParuthiyil et al., 2004). However, tumor growth in vivo is not only determined by the cell growth. It is dependent on several biological processes such as angiogenesis, paracrine and endocrine growth factor signaling, proliferation and immune cells. Usually, tumors cannot grow larger than 1 mm in diameter without the formation of new blood vessels. In this study, more necrotic areas in ERβ-transfected MCF-7 tumors were observed compared with GFP-transfected MCF-7 tumors (). The lack of angiogenesis in the ERβ-transfected MCF-7 tumors may be the reason for the observed necrosis. Thus, the tumor-suppressive effects of ERβ in our study are most likely a consequence of the combined effect of reduced angiogenesis and proliferation. Furthermore, inhibition of growth-factor expression and secretion by ERβ suggests that the paracrine effects mediated by ERβ expression may be essential in the reduction of tumor growth. In addition, CitationWilliams et al. (2008) observed that LRH-1 (NR5A2), which controls the expression of aromatase, is upregulated by ERα and inhibited by ERβ. The high level of ERβ may decrease the expression of aromatase, and thus decline the local estrogen production, which is thought to be the most important estrogen source for breast tumor development. This study provided more evidence to support that ERβ is an important modulator of proliferation and motility of breast cancer cells, and implied for the first time a possibility for the use of novel ERβ-selective therapies in breast cancer treatment.
Conclusion
Our results indicated that ERβ is an important and promising receptor and target in breast cancer management, providing a unique opportunity to use ERβ for the prevention, treatment, and prognosis of breast cancer. Novel ERβ-selective therapies include ERβ-selective agonists similar to genistein, compounds whhich could reinduce the expression of ERβ such as histone deacetylase inhibitors similar to TSA, and gene therapy to introduce ERβ into malignant cells. More ERβ agonists with high safety and efficacy such as TAS-108 (CitationKumagai et al., 2009) and Trilostane (CitationPeng et al., 2009) may be developed in the next decade. Combining the well-established treatment method such as antiestrogens with ERβ could be an effective therapeutic strategy. Findings of this study showed that ERβ brings new hope for breast cancer patients.
Of course, inevitably, limitations of the conclusion existed for that only one cell type (MCF-7) was used in this report. One or more cell types need to be explored in the future to confirm the results.
Acknowledgments
The authors thank Dr. Guowei Gu (Calabria, Italy) for the gift of Plasmid pCMV5-hERβ. They are also grateful to Professor Tao Xi (Nanjing, China) for the gift of plasmid pEGFP-C3 and other supports during this study. They acknowledge the participation of Hong Zhou to some experiments during their graduate courses.
Declaration of interest
The study was supported by Natural Science Foundation Committee (NSFC 30672015, 30700779, 30800257, 30970776) and the major project from the Ministry of Science and Technology for new drug development (2009ZX09310-004).
References
- Ali S, Coombes RC. (2002). Endocrine-responsive breast cancer and strategies for combating resistance. Nat Rev Cancer, 2, 101–112.
- Allred CD, Allred KF, Ju YH, Virant SM, Helferich WG. (2001). Soy diets containing varying amounts of genistein stimulate growth of estrogen-dependent (MCF-7) tumors in a dose-dependent manner. Cancer Res, 61, 5045–5050.
- Bardin A, Boulle N, Lazennec G, Vignon F, Pujol P. (2004). Loss of ERbeta expression as a common step in estrogen-dependent tumor progression. Endocr Relat Cancer, 11, 537–551.
- Behrens D, Gill JH, Fichtner I. (2007). Loss of tumourigenicity of stably ERbeta-transfected MCF-7 breast cancer cells. Mol Cell Endocrinol, 274, 19–29.
- Deroo BJ, Korach KS. (2006). Estrogen receptors and human disease. J Clin Invest, 116, 561–570.
- Duong V, Licznar A, Margueron R, Boulle N, Busson M, Lacroix M, Katzenellenbogen BS, Cavaillès V, Lazennec G. (2006). ERalpha and ERbeta expression and transcriptional activity are differentially regulated by HDAC inhibitors. Oncogene, 25, 1799–1806.
- Enmark E, Pelto-Huikko M, Grandien K, Lagercrantz S, Lagercrantz J, Fried G, Nordenskjöld M, Gustafsson JA. (1997). Human estrogen receptor beta-gene structure, chromosomal localization, and expression pattern. J Clin Endocrinol Metab, 82, 4258–4265.
- Gottardis MM, Robinson SP, Jordan VC. (1988). Estradiol-stimulated growth of MCF-7 tumors implanted in athymic mice: a model to study the tumoristatic action of tamoxifen. J Steroid Biochem, 30, 311–314.
- Gruvberger-Saal SK, Bendahl PO, Saal LH, Laakso M, Hegardt C, Edén P, Peterson C, Malmström P, Isola J, Borg A, Fernö M. (2007). Estrogen receptor beta expression is associated with tamoxifen response in ERalpha-negative breast carcinoma. Clin Cancer Res, 13, 1987–1994.
- Hartman J, Lindberg K, Morani A, Inzunza J, Ström A, Gustafsson JA. (2006). Estrogen receptor beta inhibits angiogenesis and growth of T47D breast cancer xenografts. Cancer Res, 66, 11207–11213.
- Hartman J, Ström A, Gustafsson JA. (2009). Estrogen receptor beta in breast cancer–diagnostic and therapeutic implications. Steroids, 74, 635–641.
- Henderson BE, Ross R, Bernstein L. (1988). Estrogens as a cause of human cancer: The Richard and Hinda Rosenthal Foundation award lecture. Cancer Res, 48, 246–253.
- Hodges-Gallagher L, Valentine CD, El Bader S, Kushner PJ. (2008). Estrogen receptor beta increases the efficacy of antiestrogens by effects on apoptosis and cell cycling in breast cancer cells. Breast Cancer Res Treat, 109, 241–250.
- Honma N, Horii R, Iwase T, Saji S, Younes M, Takubo K, Matsuura M, Ito Y, Akiyama F, Sakamoto G. (2008). Clinical importance of estrogen receptor-beta evaluation in breast cancer patients treated with adjuvant tamoxifen therapy. J Clin Oncol, 26, 3727–3734.
- Hsieh CY, Santell RC, Haslam SZ, Helferich WG. (1998). Estrogenic effects of genistein on the growth of estrogen receptor-positive human breast cancer (MCF-7) cells in vitro and in vivo. Cancer Res, 58, 3833–3838.
- Kuiper GG, Enmark E, Pelto-Huikko M, Nilsson S, Gustafsson JA. (1996). Cloning of a novel receptor expressed in rat prostate and ovary. Proc Natl Acad Sci USA, 93, 5925–5930.
- Kumagai Y, Fujita T, Ozaki M, Yokota S, Maeda M, Shida M, Otani Y, Yamaya H, Tsuruta H. (2009). Safety, tolerability and pharmacokinetics of TAS-108, a novel anti-oestrogen, in healthy post-menopausal Japanese women: A phase I single oral dose study. Basic Clin Pharmacol Toxicol, 104, 352–359.
- Lazennec G, Bresson D, Lucas A, Chauveau C, Vignon F. (2001). ER beta inhibits proliferation and invasion of breast cancer cells. Endocrinology, 142, 4120–4130.
- Levy N, Tatomer D, Herber CB, Zhao X, Tang H, Sargeant T, Ball LJ, Summers J, Speed TP, Leitman DC. (2008). Differential regulation of native estrogen receptor-regulatory elements by estradiol, tamoxifen, and raloxifene. Mol Endocrinol, 22, 287–303.
- Leygue E, Dotzlaw H, Watson PH, Murphy LC. (1998). Altered estrogen receptor alpha and beta messenger RNA expression during human breast tumorigenesis. Cancer Res, 58, 3197–3201.
- Lindberg K, Ström A, Lock JG, Gustafsson JA, Haldosén LA, Helguero LA. (2010). Expression of estrogen receptor beta increases integrin alpha1 and integrin beta1 levels and enhances adhesion of breast cancer cells. J Cell Physiol, 222, 156–167.
- Messina M, Barnes S. (1991). The role of soy products in reducing risk of cancer. J Natl Cancer Inst, 83, 541–546.
- Moore JT, McKee DD, Slentz-Kesler K, Moore LB, Jones SA, Horne EL, Su JL, Kliewer SA, Lehmann JM, Willson TM. (1998). Cloning and characterization of human estrogen receptor beta isoforms. Biochem Biophys Res Commun, 247, 75–78.
- Mosselman S, Polman J, Dijkema R. (1996). ER beta: Identification and characterization of a novel human estrogen receptor. FEBS Lett, 392, 49–53.
- Novelli F, Milella M, Melucci E, Di Benedetto A, Sperduti I, Perrone-Donnorso R, Perracchio L, Venturo I, Nisticò C, Fabi A, Buglioni S, Natali PG, Mottolese M. (2008). A divergent role for estrogen receptor-beta in node-positive and node-negative breast cancer classified according to molecular subtypes: An observational prospective study. Breast Cancer Res, 10, R74.
- Paruthiyil S, Parmar H, Kerekatte V, Cunha GR, Firestone GL, Leitman DC. (2004). Estrogen receptor beta inhibits human breast cancer cell proliferation and tumor formation by causing a G2 cell cycle arrest. Cancer Res, 64, 423–428.
- Peng J, Sengupta S, Jordan VC. (2009). Potential of selective estrogen receptor modulators as treatments and preventives of breast cancer. Anticancer Agents Med Chem, 9, 481–499.
- Pettersson K, Gustafsson JA. (2001). Role of estrogen receptor beta in estrogen action. Annu Rev Physiol, 63, 165–192.
- Rochefort H, Platet N, Hayashido Y, Derocq D, Lucas A, Cunat S, Garcia M. (1998). Estrogen receptor mediated inhibition of cancer cell invasion and motility: An overview. J Steroid Biochem Mol Biol, 65, 163–168.
- Rody A, Holtrich U, Solbach C, Kourtis K, von Minckwitz G, Engels K, Kissler S, Gätje R, Karn T, Kaufmann M. (2005). Methylation of estrogen receptor beta promoter correlates with loss of ER-beta expression in mammary carcinoma and is an early indication marker in premalignant lesions. Endocr Relat Cancer, 12, 903–916.
- Roger P, Sahla ME, Mäkelä S, Gustafsson JA, Baldet P, Rochefort H. (2001). Decreased expression of estrogen receptor beta protein in proliferative preinvasive mammary tumors. Cancer Res, 61, 2537–2541.
- Shaaban AM, Green AR, Karthik S, Alizadeh Y, Hughes TA, Harkins L, Ellis IO, Robertson JF, Paish EC, Saunders PT, Groome NP, Speirs V. (2008). Nuclear and cytoplasmic expression of ERbeta1, ERbeta2, and ERbeta5 identifies distinct prognostic outcome for breast cancer patients. Clin Cancer Res, 14, 5228–5235.
- Skliris GP, Leygue E, Curtis-Snell L, Watson PH, Murphy LC. (2006). Expression of oestrogen receptor-beta in oestrogen receptor-alpha negative human breast tumours. Br J Cancer, 95, 616–626.
- Williams C, Edvardsson K, Lewandowski SA, Ström A, Gustafsson JA. (2008). A genome-wide study of the repressive effects of estrogen receptor beta on estrogen receptor alpha signaling in breast cancer cells. Oncogene, 27, 1019–1032.
- Xing W, Archer TK. (1998). Upstream stimulatory factors mediate estrogen receptor activation of the cathepsin D promoter. Mol Endocrinol, 12, 1310–1321.
- Zhao C, Dahlman-Wright K, Gustafsson JA. (2008). Estrogen receptor beta: An overview and update. Nucl Recept Signal, 6, e003.
- Zhao C, Lam EW, Sunters A, Enmark E, De Bella MT, Coombes RC, Gustafsson JA, Dahlman-Wright K. (2003). Expression of estrogen receptor beta isoforms in normal breast epithelial cells and breast cancer: Regulation by methylation. Oncogene, 22, 7600–7606.