Abstract
Context: Psidium cattleianum Sabine (Myrtacea) is rich in vitamin C and phenolic compounds, including epicatechin and gallic acid as the main components.
Objective: To evaluate the antifungal and antioxidant capacity in vitro of the essential oil of araçá (EOA). The acute toxicity of the EOA also was evaluated in mice.
Materials and methods: The leaves of the P. cattleianum were extracted by steam distillation. The antioxidant capacity was evaluated by in vitro tests [1,1-diphenyl-2-picryl-hydrazyl (DPPH), 2,2-azinobis(3-ethylbenzothiazoline-6-sulfonate) (ABTS), ferric ion reducing antioxidant power (FRAP), linoleic acid oxidation, thiobarbituric acid reactive species (TBARS)], and ex vivo analysis [TBARS, δ-aminulevunilate dehydratase (δ-Ala-D) and catalase activity, non-protein thiols (NPSH), and ascorbic acid levels]. The toxicity was studied in mice by a single oral administration of EOA; and the antifungal activity was performed with five strains of fungi.
Results: The EOA exhibited antioxidant activity in the FRAP assay and reduced lipid peroxidation in the cortex (Imax = 32.90 ± 2.62%), hippocampus (IC50 = 48.00 ± 3.00 µg/ml and Imax = 32.90 ± 2.62%), and cerebellum (Imax = 45.40 ± 14.04%) of mice. Acute administration of the EOA by the oral route did not cause toxicological effects in mice (LD50 > 500 µg/ml). The EOA also showed antifungal activity through of the determination minimum inhibitory concentration (MIC) values ranging from 41.67 ± 18.04 to 166.70 ± 72.17 µg/ml for tested strains.
Conclusion: The results of present study indicate that EOA possess antioxidant properties, antifungal and not cause toxicity at tested doses.
Introduction
Psidium cattleianum Sabine (Myrtacea) is known as strawberry guava or araçá. The fruits have white pulp and a tart taste, and are rich in vitamin C (Lapcik et al., Citation2005; Luximon-Ramma et al., Citation2003; Pino et al., Citation2001) and contain a large amount of phenolic compounds including epicatechin and gallic acid as the main components (Medina et al., Citation2011).
Natural antioxidants are in high demand for bio-pharmaceuticals application in several fields of study. In fact, intensive research has been performed for the extraction, characterization, and utilization of natural products/antioxidants, like essential oils (EOs) and plant extracts, which may serve as potent candidates in combating the oxidative-related pathologies (Ozen et al., Citation2011).
In this sense, EOs are aromatic oily liquids obtained from plant material, like flowers, buds, seeds, leaves, twigs, bark, herbs, wood, fruits, and roots (Bakkali et al., Citation2008). The percentage of the chemical components of EOs varies among species and plants parts. These components are chemically derived from terpenes and their oxygenated derivatives (Solórzano-Santos & Miranda-Novales, Citation2012). EO possesses many pharmacological effects described, such as antioxidant (Victoria et al., Citation2012; Wang et al., Citation2013), antimicrobial (Singh et al., Citation2013; Stefanakis et al., Citation2013), and antinociceptive (Amorim et al., Citation2009).
In the context of natural products biological potential, the antioxidant potential of native fruits from the southern of Rio Grande do Sul, Brazil, such as “araçá” (Psidium cattleianum) have been studied (Alvarenga et al., Citation2013; Pereira et al., Citation2013).
Araçá, with yellow or red berries, has a nice balance between soluble solids and acidity, and ripens in Brazil in late summer between February and May (Drehmer & Amarante, Citation2008). Preliminary exploratory studies suggested high antioxidant activity and high phenolic content differing among araçá genotypes (Medina et al., Citation2011). The few investigations of araçá suggest nutritional and functional potential (Galho et al., Citation2007). Although traditionally appreciated for its sensory attributes and expected functional properties, araçá is still poorly characterized, and limited scientific information is available about the fruit.
Few studies were performed with the leaves of araçá. Alvarenga et al. (Citation2013) evaluated the in vivo analgesic and toxicity of a hydroalcoholic extract from P. cattleianum, the results demonstrated that extract demonstrated strong analgesic activity and no toxicity in cultures of LLC-MK2 mammalian fibroblast cells. Aqueous extract of P. cattleianum leaves reduced enamel demineralization, acidogenic potential, microorganism viability, and extracellular polysaccharide production (Brighenti et al., Citation2012), and a chloroform leaf extract activated specific intracellular death-related pathways, leading to caspase-3 activation and induction of apoptosis in SNU-16 cells (Moona et al., Citation2012).
Taken together the potential of EOs and araçá, and the experience of our research group in the search for new biologically active compounds, the aim of this study was to evaluate the chemical composition of EO from the leaves of araçá (EOA), the antioxidant effects and analyze whether it caused acute toxicity when orally administered to mice. Additionally, studies were performed to evaluate the antifungal activity of EOA against fungal strains.
Materials and methods
Animals
Adult male Swiss mice (25–35 g) were used. The mice were kept in separate animal rooms on a 12 h light/dark cycle at a temperature of 22 ± 2 °C and with free access to food and water. The mice were treated according to the guidelines of the Committee on Care and Use of Experimental Animal Resources of the Federal University of Pelotas, Pelotas, Brazil (CEEA 2762).
Plant material and EO material
The leaves of the P. cattleianum red plant were collected from a research orchard (germplasm collection of Embrapa Clima Temperado, Pelotas, RS, Brazil) in February and March 2012 in the morning (voucher specimen PEL no. 26.320). EOA was extracted by steam distillation. The fresh leaves (600 g) were cut with scissors into small pieces and put in a 2000 ml reaction flask. After 3 h of extraction, the organic phase was separated and dried over Na2SO4. The distillations were performed in triplicate to determine the yield. The chemical composition of the crude EO was evaluated using gas chromatography coupled with mass spectrometry (GC–MS).
Analysis of the EOA
The identification and determination of the major chemical constituent ratio of EOA were performed by GC–MS. The oil was dissolved in hexane, and the injected sample volume was 1.0 µl. A Shimadzu GC–MS QP2010 (Tokyo, Japan) and a polyethylene glycol (Carbowax), model Rtx-Wax (RESTEC) (30 m × 0.25 mm i.d., film thickness 0.25 µm) capillary column were used for the analysis. The temperature was first held at 40 °C, and then raised to 250 °C (10 min, 20° C/min). The carrier gas was helium at a flow rate of 3 ml/min. The components of the oil were identified based on the comparison of their retention indices and mass spectra with the fragmentation patterns from computer matching with the NIST/EPA/NIH/2005 library.
Determination of in vitro antioxidant activity of EOA
The EOA was diluted in dimethyl sulfoxide (DMSO) for the in vitro studies. The DPPH radical is a stable free radical commonly used as a substrate to evaluate in vitro antioxidant activity. Radical-scavenging activity was determined by the reaction of the stable DPPH radical with the compounds, in accordance with the method with some modifications (Choi et al., Citation2002). Different concentrations of EOA (10–500 μg/ml) were mixed with a methanol solution containing the DPPH radical. The mixture was incubated for 30 min at 30 °C, and the absorbance was measured at 517 nm. The values are expressed as the percentage of inhibition of DPPH absorbance (% inhibition) in relation to the control values without the EO.
ABTS radical scavenging activity was determined according to the method described by Re et al. (Citation1999) with some modifications. ABTSS+ was produced by react the ABTS stock solution with 2.45 mM potassium persulfate (final concentration) and allowing the mixture to stand in the dark at room temperature for 12–16 h before use. For the study of samples, the ABTSS+ stock solution was diluted with phosphate-buffered saline (5 mM, pH 7.4) to an absorbance of 0.70 at 0.73 nm. Different concentrations of EOA (10–50 μg/ml) were mixed with ABTSS+ solution, and the mixture was left to stand for 30 min at 30 °C; the absorbance was measured at 734 nm. The values are expressed as the percentage of inhibition of ABTSS+ absorbance (% inhibition) in relation to the control values without compound.
The ferric ion (Fe3+) reducing antioxidant power (FRAP) method was used to measure the reducing capacity of EOA. The FRAP assay was carried out as described by Stratil et al. (Citation2006). The FRAP reagent was prepared by mixing 38 mM anhydrous sodium acetate in distilled water (pH 3.8), 20 mM FeCl3ċ6H2O in distilled water, and 10 mM 2,4,6-tri(2-pyridyl)-S-triazine (TPTZ) in 40 mM HCl in proportions of 10:1:1. This reagent was freshly prepared before each experiment. Different concentrations of EOA and FRAP reagent were added to each sample, and the mixture was incubated at 37 °C for 40 min in the dark. The absorbance of the resulting solution was measured at 593 nm by a spectrophotometer.
The capacity of EOA to reduce lipid peroxidation was evaluated by three methods: linoleic acid oxidation, lipid peroxidation induced by sodium nitroprusside (SNP) in regions of mouse brain (cortex, hippocampus, and cerebellum), and basal lipid peroxidation in liver, kidney, and brain of mice.
The linoleic acid peroxidation assay was based on the reaction mixture contained linoleic acid (48.8 mM), Tris-HCl (100 mM, pH 7.5), SNP (100 µM), and a varying concentrations of EOA (10–500 µg/ml). The reaction was initiated by the addition of SNP, incubated for 30 min at 37 °C, and terminated by the addition of trichloroacetic acid (TCA, 5.5%). An aliquot of 250 µL of thiobarbituric acid (TBA, 1%) in 50 mM NaOH was added to 250 of the reaction mixture, followed by heating (90 °C) for 10 min, after this time, the organic phase was extracted with 500 µL of butanol (PA) and centrifuged at 3500g for 10 min and the absorbance of thiobarbituric acid-reacting substances (TBARS) in the supernatant was read at 532 nm and converted into the percentage oxidation (Choi et al., Citation2002).
To evaluate the effect of EOA in lipid peroxidation in tissue of mice, the animals were euthanized, and the hepatic, renal, and cerebral tissues were rapidly removed and placed on ice and the brain regions were dissected. All tissues were kept chilled and homogenized in 50 mM Tris-HCl at pH 7.4 (liver and kidney (1/10) and brain and brain regions (1/5), weight/volume [w/v]). The homogenate was centrifuged for 10 min at 2400 rpm to yield a pellet that was discarded and a low-speed supernatant (S1) for each tissue, which was used for SNP-induced lipid peroxidation and basal lipid peroxidation.
Lipid peroxidation in the cortex, hippocampus, and cerebellum was performed by the formation of TBARS during an acid-heating reaction as previously described by Ohkawa et al. (Citation1979). A 20 µl aliquot of the cortex and cerebellum (S1) and a 10 µl aliquot of the hippocampus S1 were pre-incubated in the presence of EOA (1–500 µg/ml) with and without SNP (100 µM) for 1 h at 37 °C. The mixture was then incubated with sodium dodecyl sulfate (SDS, 8.1%), TBA (0.8%), and acetic acid/HCl (pH 3.4) at 95 °C for 2 h. Malondialdehyde (MDA) was used as a biomarker of fatty-acid peroxidation. The absorbance of the sample was measured at 532 nm, and the results are expressed as percentage of lipid peroxidation.
To extend the studies about the antioxidant potential of the EOA, its effect on the in vitro basal lipid peroxidation on liver, kidney, and brain was evaluated according to the methodology of Ohkawa et al. (Citation1979). An aliquot of 200 µl of S1 was pre-incubated for 1 h at 37 °C with different concentrations of EOA (10–500 µg/ml) or vehicle (DMSO). The mixture was then incubated with SDS (8.1%), TBA (0.8%), and acetic acid/HCl (pH 3.4) at 95 °C for 2 h. MDA was used as a biomarker of lipid peroxidation and the results are expressed as % of lipid peroxidation.
In vivo experiments
Acute toxicity
To investigate the potential acute toxicity caused by the EOA, the mice received a single oral dose of the EOA (100, 200, and 500 mg/kg) or a vehicle (10 ml/kg of canola oil). After administration, the animals were observed for up to 72 h (at the interval of 24 h) to determine the lethal dose (LD50). Body weight gain was recorded as a sign of general toxicity. After 72 h of exposure, the mice were euthanized by cervical displacement, and the brain, liver, and kidney were removed, homogenized (liver and kidney 1/10 v/v and brain 1/5 v/v), and centrifuged. The S1 was separated and used for analysis of TBARS, ascorbic acid, and non-protein thiols (NPSH) levels as well as the (δ-aminulevunilate dehydratase) (δ-Ala-D) and catalase activities (see section Analysis of the EOA).
Ex vivo analysis
Among the lipid peroxidation products used for antioxidant assays, MDA has been most widely used to evaluate the antioxidant activity in lipid peroxidation systems. In this work, MDA formation was used as a marker of lipid peroxidation according to the method reported by Ohkawa et al. (Citation1979).
The δ-Ala-D was assayed according to the method reported by Sassa (Citation1982). δ-Ala-D is a sulfhydryl-containing enzyme and numerous metals and other compounds that oxidized sulfhydryl groups modified its activity.
Catalase (CAT) activity, an enzymatic antioxidant defense, was assayed spectrophotometrically by the method of Aebi (Citation1984), which involves monitoring the disappearance of H2O2 in the homogenate at 240 nm. The enzymatic activity was expressed in units of U CAT/mg protein. Vitamin C, a non-enzymatic antioxidant defense, determination was performed as described by Jacques-Silva et al. (Citation2001) with some modifications. NPSH levels were analyzed by the method of Ellman (Citation1959).
Protein determination
The protein content of the S1 was measured according to the method reported by Lowry et al. (Citation1951) using bovine serum albumin (BSA) as a standard.
Antifungal activity
The antifungal activity of EOA was tested with five fungal strains: Candida albicans, Candida parapsilosis, Candida guilhermondii, Candida lipolytica, and Trichosporon asahii that were obtained from the Department of Microbiology at the Federal University of Pelotas. Fungal strains were maintained on potato dextrose agar (PDA) and subcultured (1% inoculum) in potato dextrose broth at 35 °C for at least 2–4 d before being used in the screening assays.
The minimum inhibitory concentration (MIC) of EOA was determined according to the method of NCCLS (Citation2002). EOA was tested in concentrations ranging from 500 to 0.85 µg/ml, and the assays were repeated at least three times in their entirety to confirm the results. The MIC was recorded as the lowest concentration of EOA that inhibited the fungal growth.
Statistical analysis
Experimental results were given as the mean ± standard error (SE) to show variations among groups. Statistical analyses were performed using one-way ANOVA followed by the Newman–Keuls multiple comparison test when appropriate. All in vitro tests were performed at least three times in duplicate. For the in vivo assays, five to eight animals were used per group. The IC50 values (concentration of sample required to inhibit 50% of the effect) were calculated from the graph of the inhibition percentage versus the EOA concentration. Differences were considered statistically significant at a probability of less than 5% (p < 0.05).
Results
Chemical composition of EOA
This is the first time that the chemical composition of EOA of Rio Grande do Sul, Brazil, was investigated, according to our knowledge. The GC/MS analysis permitted the identification of 97.5% of the compounds presents in EOA, with a prevalence of oxygenated sesquiterpenes (88.13%) followed by non-oxygenated sesquiterpenes (9.09%) (). The major compound found was isocaryophillene (59.62%).
In vitro assay
As shown in , EOA exhibited ferric-reducing ability at concentrations equal and higher than 100 µg/ml. EOA did not demonstrated effect in the DPPH (electron or hydrogen-based transfer antioxidant test) and in the ABTS test (ET-based antioxidant test) (data not shown).
Figure 2. Antioxidant activities of the EOA: (a) ferric ion reducing antioxidant power (FRAP). Each value is expressed as the mean ± SE (n = 3). Asterisks represent significant *p < 0.05 when compared with the respective control without essential oil. The values are expressed in absorbance; the mean value of absorbance (593 nm) of the control is 0.099 ± 0.004. (b) On the linoleic acid, SNP-induced lipid peroxidation assay. Data are presented as the mean ± S.E (n = 3). The values are expressed as induced percent. The IC50 indicates the necessary concentration to inhibit 50% of lipid peroxidation. The Imax is the maximal inhibition offered by the EO. Asterisks represent significant *p < 0.05 compared with the induced for SNP-induced sample (100% lipid peroxidation). ANOVA followed by the Newman–Keuls multiple comparison test when appropriate.
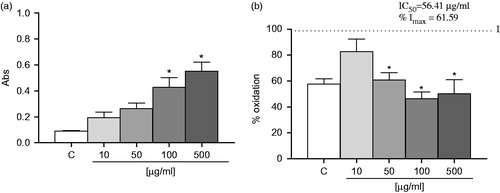
As shown in , EOA effectively inhibits SNP-linoleic acid peroxidation at a concentration equal and higher than 50 μM. EOA presented a value of IC50 of 56.41 ± 5.41 µg/ml and a maximum inhibition of 61.59%.
showed that EOA decreased lipid peroxidation per se at concentrations ranging from of 100 to 500 µg/ml in the liver, with an IC50 value of 48.00 ± 3.00 µg/ml and Imax of 65.10 ± 11.71%. In the kidney (), EOA decreased lipid peroxidation at concentrations of 100 and 500 µg/ml with an Imax value of 32.90 ± 2.62%. In addition, EOA declined lipid peroxidation in the brain and presented an Imax of 45.40 ± 14.40%.
Figure 3. Effect of EOA on lipid peroxidation: (a) liver, (b) kidney, and (c) brain. The values are expressed in nmol MDA/g tissue. Data are presented as mean ± SE (n = 5). Asterisks represent a significant effect as compared with the control group (C). *p < 0.05 by the Student–Newman–Keuls test for post-hoc comparison.
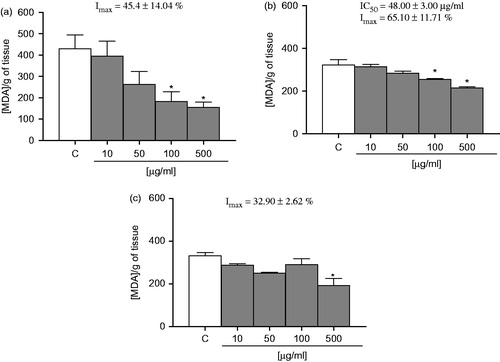
shows the effect of EOA on SNP-induced lipid peroxidation on brain regions. Data demonstrated that SNP significantly induced lipid peroxidation on the brain structures. EOA were effective on reducing the SNP-induced lipid peroxidation at concentrations equal or greater than 10 µg/ml in hippocampus, cortex and cerebellum. In the cortex, EOA presented an IC50 value of 8.33 ± 4.04 µg/ml and a maximal inhibition (Imax) of 80.90 ± 4.00% (4b), in the hippocampus EOA showed an Imax of 45.87 ± 10.20% (4 a) and in the cerebellum 28.00 ± 4.40% ().
Figure 4. Effect of EOA on lipid peroxidation induced by SNP structures brain homogenates: (a) hipocamppus, (b) cortex, and (c) cerebellum. The values are expressed in nmol MDA/g tissue. Data are presented as mean ± SE (n = 5). Asterisks represent a significant effect when compared with induced *p < 0.05, compared with the SNP-induced sample (100% lipid peroxidation) and # p < 0.01 compared with the control group (C) by the Student–Newman–Keuls test for post-hoc comparison.
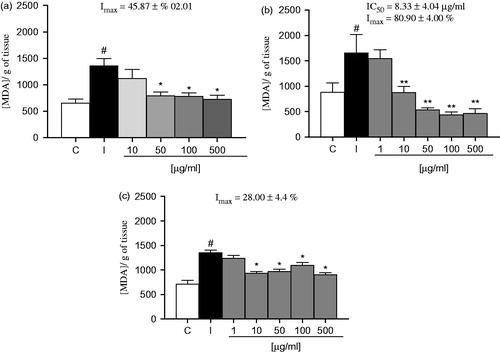
In vivo assay
Acute toxicity
The oral administration of EOA at doses of 100, 200, and 500 mg/kg did not cause death of any animal. The LD50 value obtained for the EOA possibly is greater than 500 mg/kg given that lethality was not observed at this dose. The administration of EOA at these doses did not cause significant reduction in the body weight when compared with the control group (data not shown).
Ex vivo analysis
The levels of TBARS after oral exposure of mice to EOA were significantly reduced in the liver, kidney at doses 200 and 500 mg/kg, and in the brain at dose of 500 mg/kg (). These data suggest that the EOA does not cause any oxidative stress in mice tissue after acute treatment, although it reduces the levels of TBARS in mice liver, kidney, and brain.
Figure 5. Effect of EOA on lipid peroxidation ex vivo: (a) liver, (b) kidney, and (c) brain. The values are expressed in nmol MDA/g tissue. Data are presented as mean ± SE (n = 5). Asterisks represent a significant effect as compared with the control group (C). *p < 0.05 by the Student–Newman–Keuls test for post-hoc comparison.
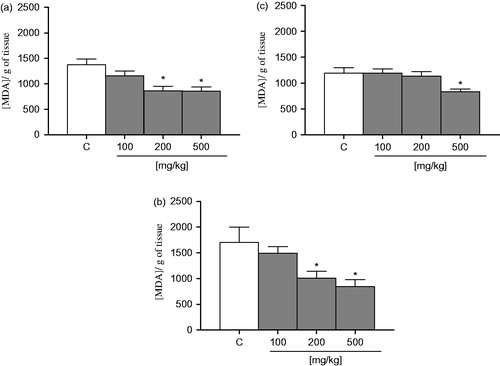
δ-Ala-D is a sulfhydryl-containing enzyme that is inhibited by a variety of sulfhydryl reagents (Folmer et al., Citation2005). In this study, the EOA did not alter the enzyme activity in liver, kidney and brain of mice (data not shown).
CAT activity was not altered in the liver, kidney, or brain of mice treated with EOA at any of the tested doses when compared with the control group. Also, the administration of EOA did not modified vitamin C levels and NPSH in the liver and kidney of mice ().
Table 1. Effect of acute treatment of EOA on catalase activity and ascorbic acid and NPSH levels.
Antifungal activity
In our study of antifungal activity, EOA presented MIC values ranging from 41.67 ± 18.04 to 166.70 ± 72.17 µg/ml for the tested strains (). To our knowledge, this is the first report describing the antifungal activity of EOA.
Table 2. Evaluation of the antifungal activity of EOA against fungal strains.
Discussion
Due to the undesirable effects of synthetic compounds, there has been growing interest in the investigation of natural products for the discovery of active compounds with antimicrobial and antioxidant properties that do not have any negative effects on the human health; one of these natural additives are the EOs.
In this study, EOA presented an antioxidant effect in the FRAP, TBARS in vitro (SNP-induced linoleic oxidation, basal lipid peroxidation in liver, kidney and brain, SNP-induced brain regions oxidation), and TBARS ex vivo. Also, EOA did not present any sign of toxicity and did not alter important oxidative parameters, like δ-Ala-D and catalase activities and vitamin C and NPSH levels. Furthermore, EOA inhibited significantly the growth of different clinically important fungal strains.
The chemical compositional analysis of EOA presents the predominance of non-oxygenated sesquiterpenes and the major compound found was isocaryophillene (59.62%). Chalannavar et al. (Citation2013) studied the chemical composition of the EO of P. cattleianum of South Africa by hydrodistillation, the analysis revealed caryophyllene oxide as the major compound, and a predominance of non-oxygenated sesquiterpenes (36.78%), corroborating with our findings.
There was a great variability in the chemical composition of EOs. Such variability depends on several factors including local climatic and environmental conditions, soil variations, season, geographical location, geology, the stage of the vegetative cycle, part of the plant, and the method used to obtain the EO (Viuda-Martos et al., Citation2008).
The terpenoid compounds present in the chemical composition of EOs are closely associated with their antioxidant function, mainly due to their redox properties exerted by various possible mechanisms: free radical-scavenging activity, hydrogen donors, transition metal chelating activity, and/or singlet oxygen quenching capacity (Liyana-Pathirana & Shahidi, 2006). However, the mechanism set in motion by the antioxidant activity of these compounds is still not clearly understood. According to Amensour et al. (Citation2009), the antioxidant activity of EO is believed to be mainly due to their redox properties, which play an important role in adsorbing and neutralizing free radicals, quenching singlet and triplet oxygen, or decomposing peroxides.
In agreement with our results, EOA presented an antioxidant effect in the FRAP assay. The FRAP assay measures the ability of antioxidant to reduce Fe(III)–triazine complex to Fe(II)–complex (the respective lower valence state). Studies have demonstrated that the reducing power of natural plant extracts and EOs might be strongly correlated with their antioxidant activities (Gülçin et al., Citation2012; Stratil et al., Citation2006; Victoria et al., Citation2012).
Other important findings of our work are the effect of EOA in the lipid peroxidation in the assays of SNP-induce linoleic acid oxidation, in the SNP-induced lipid peroxidation in brain structures, reduced the basal lipid peroxidation in the liver, kidney, and brain, and when orally administered, reduced the levels of MDA in liver, kidney, and brain of mice. Excessive production of free radicals can generate a lipid peroxidation chain reaction, which are responsible for many pathological disorders (Castro et al., Citation2009).
According to Choi et al. (Citation2002), the inhibition of linoleic acid peroxidation by natural products is effective because of the presence of various phytochemicals, such as phenolic compounds, amino acids, ascorbic acid, tocopherols, and pigments that might contribute to some antioxidant activity singly or in combination.
Recently our research group demonstrated the effect of another EO of the Myrtaceae family, the EO of Eugenia uniflora L. leaves, in lipid peroxidation (Victoria et al., Citation2013). Since the chemical composition of both EOs (P. cattleianum and E. uniflora) revealed sesquiterpenes as major compounds, we can infer that the presence of these compounds can be related with the effect of EOs in lipid peroxidation. Sesquiterpenes are formed from the assembly of three isoprene units (C15). The extension of the chain increases the number of cyclizations which allows a great variety of structures (Bakkali et al., Citation2008).
In order to evaluate the possible toxic effect of EOA, mice were orally treated with different doses of EOA. The results demonstrated that the EOA did not present any signal of toxicity. The tissues of animals were used to evaluate the effect of EOA in some oxidative parameters, like TBARS, CAT activity, and ascorbic acid levels. According to the results, the levels of MDA were significantly reduced in liver, kidney, and brain. MDA, one of the products of lipid peroxidation, is malondialdehyde, which can be determined by measuring the amount of TBA reactive species (Reznick & Packer, Citation1994). According to many authors, lipid peroxidation is involved in neurodegenerative disorders like Huntington disease, amyotrophic lateral sclerosis, and Alzheimer disease.
The activity of catalase, an enzymatic defense, and the levels of ascorbic acid and NPSH, a non-enzymatic defense, are considered markers of oxidative stress, and a decrease in its content might indicate an increase in oxidative stress. In this work, the activity of catalase and the levels of ascorbic acid were not changed by the administration of EOA, based on this information it is possible suggest that EOA, orally administered did not induced oxidative stress. Indeed, taken together these data with results of lipid peroxidation, EOA reduced the oxidative stress in mice at the tested doses.
Plants are rich in a wide variety of secondary metabolites, such as phenols, terpenoids, sesquiterpenes, hydrocarbons, flavonoids, tannins, acids, alcohols, aldehydes, and alkaloids, which have been found to have antimicrobial properties in in vitro studies. In addition, minor components play an important part for antimicrobial activity, possibly by producing a synergistic effect between other components. Especially EOs, the aromatic oily liquid, are responsible for antimicrobial activities of medicinal plants, which is effective against microbial deterioration. Supercritical fluid extracts, methanol, and ethanol extracts, and water extracts are also utilized, but generally less effective (Skrovánková et al., Citation2012).
EOA presents interesting effect in the inhibition of important clinical fungal strains like T. asahii, C. parapsilosis, C. albicans, C. lipolytica, and C. guilhermondi. There are several studies that show that coriander, celery, and sweet basil EO have an inhibitory effect on the growth of yeasts (Matasyoh et al., Citation2008). Although the EOs showed antimicrobial activity, the reason behind this capacity is not well documented. This antimicrobial activity could be caused by the major compounds of the EOs or due to a synergistic effect between the major compounds and the minor ones (Carović-Stanko et al., Citation2010).
Possible mechanisms by which mycelial growth may be reduced or totally inhibited have been proposed. Thus, Lucini et al. (Citation2006) indicated mycelial growth inhibition is caused by the monoterpenes present in the EOs. These components could increase the concentration of lipidic peroxides such as hydroxyl, alkoxyl, and alkoperoxyl radicals, which leads to cell death. According to Sharma and Tripathi (Citation2006), the EOs would act on the hypha of the mycelium, provoking exit of components from the cytoplasm, the loss of rigidity, and integrity of the hypha cell wall, resulting in its collapse and death of the mycelium.
Conclusion
The EOA showed antioxidant activity and presented a LD50 higher than 500 mg/kg in mice. EOA demonstrated antimicrobial activity against fungal strains. Thus, based on the promising results shown in this work, other pharmacological studies will be performed with this EO.
Declaration of interest
All authors declare that there are no conflicts of interest. L. S., D. S. A., and R. G. J. are recipients of CNPq fellowships. This work was supported by CNPq (Grants 472644/2010-6), CAPES (23038.008258/2011-93), and FAPERGS (PRONEX 10/0027-4, PqG 1012043).
References
- Aebi H. (1984). Catalase in vitro. Meth Enzymol 105:121–7
- Alvarenga FQ, Mota BCF, Leite MN, et al. (2013). In vivo analgesic activity, toxicity and phytochemical screening of the hydroalcoholic extract from the leaves of Psidium cattleianum Sabine. J. Ethnopharmacol 150:280–4
- Amensour M, Sendra E, Abrini J, et al. (2009). Total phenolic content and antioxidant activity of myrtle (Myrtus communis) extracts. Nat Prod Commun 4:819–24
- Amorim AC, Lima CK, Hovell AM, et al. (2009). Antinociceptive and hypothermic evaluation of the leaf essential oil and isolated terpenoids from Eugenia uniflora L. (Brazilian Pitanga). Phytomedicine 16:923–8
- Bakkali F, Averbeck S, Averbeck D, Idaomar M. (2008). Biological effects of essential oils − A review. Food Chem Toxicol 46:446–75
- Brighenti FL, Gaetti-Jardim E, Danelon M, et al. (2012). Effect of Psidium cattleianum leaf extract on enamel demineralisation and dental biofilm composition in situ. Arch Biol 57:1034–40
- Carović-Stanko K, Orlić S, Politeo O, et al. (2010). Composition and antibacterial activities of essential oils of seven Ocimum taxa. Food Chem 119:196–201
- Castro MR, Lima JV, Freitas DP, et al. (2009). Behavioral and neurotoxic effects of arsenic exposure in zebrafish (Danio rerio, Teleostei: Cyprinidae). Comp Biochem Physiol C Toxicol Pharmacol 150:337–42
- Chalannavar RK, Narayanaswamy VK, Baijnath H, Odhav B. (2013). Chemical constituents of the essential oil from leaves of Psidium cattleianum var. cattleianum. J Med Plants Res 7:783–9
- Choi CW, Kim SC, Hwang SS, et al. (2002). Antioxidant activity and free radical scavenging capacity between Korean medicinal plants and flavonoids by assay-guided comparison. Plant Sci 163:1161–8
- Drehmer AMF, Amarante CVTD. (2008). Post harvest preservation of red strawberry–guavas as affected by maturity stage and storage temperature. Rev Bras Frutic 30:322–6
- Ellman GL. (1959). Tissue sulfhydryl groups. Arch Biochem 82:70–6
- Folmer V, Bolzan RC, Farina M, et al. (2005). Mechanism of delta-aminolevulinate dehydratase inhibition by phenyl selenoacetylene involves its conversion to diphenyl diselenide. Toxicology 206:403–11
- Galho AS, Lopes NF, Bacarin MA, Lima MDGDS. (2007). Composição química e respiratório de crescimento em frutos de Psidium cattleyanum sabine durante o ciclo de desenvolvimento. Rev Bras Frutic 29:61–6
- Gülçin I. (2012). Antioxidant activity of food constituents: An overview. Arch Toxicol 86:345–91
- Jacques-Silva MC, Nogueira CW, Broch LC, et al. (2001). Diphenyl diselenide and ascorbic acid changes deposition of selenium and ascorbic acid in liver and brain of mice. Pharmacol Toxicol 88:119–25
- Lapcik O, Klejdus B, Kokoska L, et al. (2005). Identification of isoflavones in Acca sellowiana and two Psidium species (Myrtaceae). Biochem Syst Ecol 33:983–92
- Liyana-Pathirana CM, Shahidi F. (2006). Antioxidant properties of commercial soft and hard winter wheats (Triticum aestivum L.) and their milling fractions. J Sci Food Agricult 86:477–85
- Lowry OH, Rosemburg NJ, Farr AL, Roudall R. (1951). Protein measurement with Folin-Phenol reagent. J Biol Chem 193:265–75
- Lucini EI, Zunin MP, López ML, Zygadlo JA. (2006). Effect of monoterpenes on lipid composition and sclerotial development of Sclerotium cepivorum Berk. J Phytopathol 154:441–6
- Luximon-Ramma A, Bahorun T, Crozier A. (2003). Antioxidant actions and phenolic and vitamin C contents of common Mauritian exotic fruits. J Sci Food Agric 83:496–502
- Matasyoh LG, Matasyoh JC, Wachira FN, et al. (2008). Antimicrobial activity of essential oils of Ocimum gratissimum L. from different populations of Kenya. Afr J Tradit Complement Altern Med 5:187–93
- Medina AL, Haas LIR, Chaves FC, et al. (2011). Araçá (Psidium cattleianum Sabine) fruit extracts with antioxidant and antimicrobial activities and antiproliferative effect on human cancer cells. Food Chem 128:916–22
- Moona JY, Mosaddika A, Kima H, et al. (2012). The chloroform fraction of guava (Psidium cattleianum Sabine) leaf extract inhibits human gastric cancer cell proliferation via induction of apoptosis. Food Chem 125:369–75
- National Committee of Clinical Laboratory Standards (NCCLS). (2002). In: National Committee for Clinical Laboratory Standards. Reference method for broth dilution antifungal susceptibility testing of yeasts: Proposed standard. M27-A2
- Ohkawa H, Ohishi N, Yagi K. (1979). Assay for lipid peroxides in animal tissues by thiobarbituric acid reaction. Anal Biochem 95:351–8
- Ozen T, Demirtas I, Aksit H. (2011). Determination of antioxidant activities of various extracts and essential oil compositions of Thymus praecox subsp. skorpilii var. skorpilii. Food Chem 124:58–64
- Pereira MC, Steffens RS, Jablonski A, et al. (2013). Characterization, bioactive compounds and antioxidant potential of three Brazilian fruits. J Food Comp Anal 29:19–24
- Pino JA, Marbot R, Vázquez C. (2001). Characterization of volatiles in strawberry guava (Psidium cattleianum Sabine) fruit. J Agric Food Chem 49:5883–7
- Re R, Pellegrini N, Proteggente A, et al. (1999). Antioxidant activity applying an improved ABTS radical cation decolorization assay. Free Radic Biol Med 26:1231–7
- Reznick AZ, Packer L. (1994). Oxidative damage to proteins: spectrophotometric method for carbonyl assay. Methods Enzymol 233:357–63
- Sassa S. (1982). delta-Aminolevulinic acid dehydratase assay. Enzyme 28:133–45
- Skrovánková S, Misurcová L, Machu L. (2012). Antioxidant activity and protecting health effects of common medicinal plants. Adv Food Nutr Res 67:75–139
- Sharma N, Tripathi A. (2006). Fungitoxicity of the essential oil of Citrus sinensis on post-harvest pathogens. World J Microbiol Biotechnol 22:587–93
- Singh C, Singh S, Pande C, et al. (2013). Exploration of antimicrobial potential of essential oils of Cinnamomum glanduliferum, Feronia elephantum, Bupleurum hamiltonii and Cyclospermum leptophyllum against foodborne pathogens. Pharm Biol 51:1607–10
- Solórzano-Santos F, Miranda-Novales MG. (2012). Essential oils from aromatic herbs as antimicrobial agents. Curr Opinion Biotechnol 23:136–4
- Stratil P, Klejdus B, Kuban V. (2006). Determination of total content of phenolic compounds and their antioxidant activity in vegetables evaluation of spectrophotometric. Meth J Agric Food Chem 54:607–16
- Stefanakis MK, Touloupakis E, Anastasopoulos E, et al. (2013). Antibacterial activity of essential oils from plants of the genus Origanum. Food Control 34:539–46
- Victoria FN, Brahm AS, Savegnago L, Lenardão EJ. (2013). Involvement of serotoninergic and adrenergic systems on the antidepressant-like effect of E. uniflora L. leaves essential oil and further analysis of its antioxidant activity. Neurosci Lett 544:105–9
- Victoria FN, Lenardão EJ, Savegnago L, et al. (2012). Essential oil of the leaves of Eugenia uniflora L.: Antioxidant and antimicrobial properties. Food Chem Toxicol 50:2668–74
- Viuda-Martos M, Ruiz-Navajas Y, Fernández-López J, Pérez-Álvarez J. (2008). Antifungal activity of lemon (Citrus lemon L.), mandarin (Citrus reticulata L.), grapefruit (Citrus paradisi L.) and orange (Citrus sinensis L.) essential oils. Food Control 19:1130–8
- Wang C, Yuan H, Bao X, Lan M. (2013). In vitro antioxidant and cytotoxic properties of ethanol extract of Alpinia oxyphylla fruits. Pharm Biol 51:1419–25