Abstract
Context: Sea cucumbers have been consumed as tonic, food, and nutrition supplements for many years.
Objective: The objective of this study is to investigate the antiobesity and lipid-lowering effects of sea cucumber extracts in in vitro and in vivo models and elucidate the mechanism of action of the extracts on obesity and dyslipidemia.
Materials and methods: The 60% ethanol extracts from the body walls of 10 different sea cucumbers were investigated for the inhibition of pancreatic lipase (PL) activity in vitro. The optimal active extract (SC-3) was further chemically analyzed by LC-MS and UV. And 0.1% and 0.2% of SC-3 was mixed with a high-fat diet to treat C57/BL6 mice for 6 weeks or 2 weeks as preventive and therapeutic study. The body weight, serum, and liver lipid profile in the mice were investigated.
Results: The crude extract of Pearsonothuria graeffei Semper (Holothuriidae) inhibited the PL activity by 36.44% of control at 0.5 μg/mL. SC-3 and echinoside A inhibited PL with an IC50 value at 2.86 μg/mL and 0.76 μM. 0.1% of SC-3 reduced the body weight (23.0 ± 0.62 versus 26.3 ± 0.76 g), the serum TC (2.46 ± 0.04 versus 2.83 ± 0.12 mmol/L), TG (0.19 ± 0.08 versus 0.40 ± 0.03 mmo/L), and LDL-c (0.48 ± 0.02 versus 0.51 ± 0.02 mmol/L), and liver TC (1.19 ± 0.17 versus 1.85 ± 0.13 mmol/mg) and TG (6.18 ± 0.92 versus 10.87 ± 0.97 mmol/mg) contents of the obese C57BL/six mice on a high-fat diet.
Discussion and conclusion: Sea cucumber may be used for developing antiobesity and antihyperlipidemia drugs.
Introduction
Obesity is one of the most common public health threats worldwide, particularly in western countries (Haslam & James, Citation2005). More than 1.1 billion people worldwide are above their ideal body weight, and every year, over 300 000 people in the United States die because of obesity (Melnikova & Wages, Citation2006). Obesity is accompanied by severe health issues such as diabetes, dyslipidemia, hypertension, and atherosclerosis. Few weight-reducing drugs are available on the market. Orlistat, a pancreatic lipase (PL) inhibitor, is the only drug approved by the U.S. Food and Drug Administration for long-term use. However, orlistat may cause unpleasant side effects such as steatorrhea, fecal incontinence, and urgent bowel movements (Ballinger & Peikin, Citation2002; Drent & van der Veen, Citation1995; Hsieh et al., Citation2005). Therefore, there is an urgent demand for a new weight-reducing drug among patients.
PL is a vital enzyme in the digestion of dietary fat and is responsible for the hydrolysis of 50–70% of total dietary fat (Birari & Bhutani, Citation2007). Dietary fat is absorbed by the intestine only when it has been hydrolyzed by PL. The PL inhibition is one of the most widely studied mechanisms for determining the potential efficacy of natural products as antiobesity agents. The success of orlistat encouraged the development of PL inhibitors as weight-reducing drugs. In new weight-reducing therapies with more potency or fewer side effects, several natural PL inhibitors, such as platycodin saponins, scabiosaponins, chikusetsusaponins, tea saponins, assamicain A, oolonghomobisflavan A, and oolonghomobisflavan B, have been identified as inhibitors of PL activity in vitro (de la Garza et al., Citation2011; Han et al., Citation1999,Citation2005; Roh & Jung, Citation2012; Slanc et al., Citation2009; Zhao & Kim, Citation2004; Zheng et al., Citation2004). However, the compounds are difficult to develop as antiobesity drugs because of limited sources.
Liver X receptors (LXRs), including LXR-α and LXR-β, are sterol-responsive nuclear transcription factors. LXRs play essential roles in the regulation of cholesterol, triglycerides, fatty acids, and glucose homeostasis (Baranowski, Citation2008). LXR agonists may lower cholesterol by inducing target gene expression (Beyea et al., Citation2007; Jiang et al., Citation2014; Terasaka et al., Citation2003).
Sea cucumbers are marine invertebrates that have been consumed as tonic food and nutrition supplements for many years in China. It is reported that sea cucumbers are rich in collagen, saponins, and polysaccharides. Recent studies have reported that sea cucumbers have antioxidant and immunoenhancement properties. In addition, sea cucumbers contain cytotoxic components that may be used in the treatment of leukemia and gastric, prostate, and breast cancers (Bohlooli et al., Citation2012; Gao et al., Citation2014; Ma et al., Citation2012; Wijesinghe et al., Citation2013). Moreover, sea cucumbers are used in the treatment of metabolic diseases. Dietary supplements of sea cucumber ameliorate hepatic lipid accumulation in fatty-liver rats, lower serum lipids, and cause weight loss in diet-induced obesity mice (Zhang et al., Citation2012a,Citationb). However, the action mechanism of sea cucumbers on obesity and dyslipidemia remains largely unknown. In the present study, we isolated and prepared extracts from 10 sea cucumber species and analyzed their efficacy and mechanism on metabolic disorders in a high-fat-diet-induced obese mouse model.
Materials and methods
Materials
Ten dry adult sea cucumber species (), T. ananas, Acaudina leucopraocta H. L. Clark (Caudinidae), Holothuria leucospilota Brandt (Holothuriidae), Holothuria pardalis Selenka, Holothuria hilla Lesson, P. graeffei, Holothuria nobilis Selenka, Stichopus chloronotus Brandt (Stichopodidae), Holothuria verrucosa Selenka, and H. fuscogliva, were purchased from Beihai City (Guangxi, China) in July 2012, and authenticated by Prof. Xiaoqi Zeng in Fisheries College, Ocean University of China. Lipase (type II from porcine pancreas), 4-nitrophenyl butyrate, and orlistat were purchase from Sigma-Aldrich (St. Louis, MO). The standard substance echinoside A was purchased from Shanghai Research Center of Standardization of Chinese Medicines (Shanghai, China).
Figure 1. The photos of 10 dry adult sea cucumbers. (A) Acaudina leucopraocta H. L. Clark; (B) Thelenota ananas (Jaeger); (C) Stichopus chloronotus Brandt; (D) Pearsonothuria graeffei Semper; (E) Holothuria pardalis Selenka; (F) Holothuria verrucosa Selenka; (G) Holothuia leucospilota (Brandt); (H) Holothuia fuscogliva Cherbonnier; (I) Holothuia nobilis (Selenka); (J) Holothura hilla Lesson.
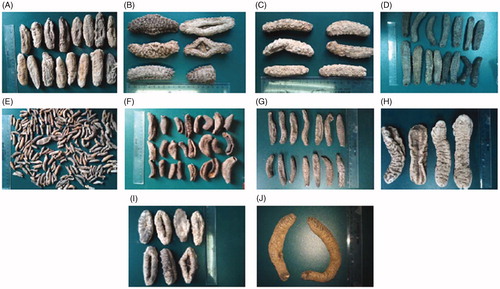
Preparation of the extracts
The body walls of sea cucumbers were ground into powders, and 1.0 kg of the powder was extracted three times by refluxing with 60% ethanol at 85 °C. The filtrates were combined and vacuum evaporated to remove ethanol. Subsequently, the concentrated extracts were diluted with water and extracted three times with the same amount of methylene chloride and water-saturated n-butanol, respectively. The water-saturated n-butanol portion was collected, evaporated in vacuum, and then lyophilized to obtain the extracts (137.0 g), which was named for SC-3. The yield from the raw material was approximately 13.7%.
Liquid chromatography–mass spectrometry (LC-MS) assay of SC-3
LC-MS analysis was performed using Shimadzu HPLC equipment (Shimadzu Inc., Tokyo, Japan) and a Thermo LCQ Fleet instrument (Thermo Scientific Co., Waltham, MA) with an Agilent Zorbax SB-C18 column (Agilent Technologies Inc., Atlanta, GA) (5 μm, 4.6 × 150 mm). The mobile phase was a linear gradient starting at 35% acetonitrile in 0.1% aqueous trifluoroacetic acid for 5 min and then increasing to 95% acetonitrile in 45 min. The flow rate was set to 1.0 mL/min. The outlet of the HPLC system was split (3:1) to the ESI–MS interface of the mass analyzer in the negative ion mode. ESI–MS analyses were performed under the following optimized conditions: source voltage, 4.5 kV; capillary voltage, −37 V; capillary temperature, 320 °C, sheath gas (N2) flow, 40 arbitrary units (arb); and auxiliary gas (N2) flow, 10 arb. Full-scan MS spectra (range, 200–2000 m/z) were initially recorded during the chromatographic run. The pseudomolecular ions of each reaction product were then identified. MS/MS spectra were recorded by isolating the target pseudomolecular ion in the ion trap followed by collision-induced dissociation (CID). The collision energy required for this process was set at 35% of the total available collision energy.
Analysis of the total saponin content of SC-3
The total saponins in the SC-3 were assayed using the vanillin–perchloric acid colorimetric method. 1.0 mL of SC-3 (1 mg/mL) was diluted in 1.0 mL of H2O, 0.4 mL of this dilution was transferred to a test tube, and then 0.2 mL of 5% vanillin–acetic acid and 0.8 mL of perchloric acid were added immediately to the test tube sample. The sample was thoroughly mixed and then heated at 60 °C in a water bath for 15 min. Next, the samples were placed in an ice water bath to cool and kept at room temperature for 10 min. A triterpene purified from P. graeffei, 3β,17α-dihydroxy-8(9),11(12)-diene-holost, was used as a reference standard in a range of 50–350 μg/mL. All the samples and standards were prepared in triplicate, and absorbance was measured at 591 nm against a blank control containing all reagents except the sample. Total saponins were calculated from the linear regression equation plotted from the standard curve revealing the percentage in SC-3.
Total polysaccharides content analysis of SC-3
The total polysaccharides in the SC-3 were assayed using the phenol–sulfuric acid colorimetric method (Masuko et al., Citation2005). Hence, 1.0 mL of SC-3 (1 mg/mL) was diluted in 1.0 mL of ddH2O to which 1 mL of 5% phenol and 5 mL of 98% H2SO4 were added immediately. The sample was mixed and placed at room temperature for 5 min. Next, the samples were placed in boiling water for 30 min and then cooled at room temperature. A range of 10–250 μg/mL of glucose was used as the reference standard. All the samples and standards were prepared in triplicate and absorbance was measured at 490 nm against a blank control containing all the reagents except the sample. Total polysaccharides were calculated from the linear regression equation, plotted from the standard curve and indicated as their percentage in SC-3.
Total amino acid analysis of SC-3
Total amino acid content in SC-3 was analyzed according to the Bradford assay method (Deters et al., Citation2005) using a protein assay kit (Beyotime, Beijing, China). Total amino acids were shown as the % of SC-3.
HPLC analyses of the principal component echinoside A of SC-3
A HPLC method was developed to determine the principal component echinoside A of the extract. For this, 10 mg SC-3 sample was placed in a 10 mL volumetric flask. Then, 8 mL of distilled water was added to this, and the flask was sonicated for 3 min, cooled to room temperature, and diluted to 10 mL. The solution was filtered with 0.45 μm microporous membrane filter for use in the HPLC injection. Chromatographic analyses were performed on an Agilent1200 series HPLC instrument (Agilent Technologies Inc., Atlanta, GA) containing a quaternary HPLC pump, a column heater, diode array detector, and an Agilent Chem-Station (Agilent Technologies Inc., Atlanta, GA) for data collection and manipulation. Reverse phase separations of the saponin glycosides were performed on an Agilent Zorbax SB-C18 column (Agilent Technologies Inc., Atlanta, GA) (5 μm, 150 × 4.6 mm). The mobile phase was a linear gradient starting at 35% acetonitrile in 0.1% aqueous trifluoroacetic acid for 5 min, and then increasing to 95% acetonitrile in 45 min. The flow rate was set to 1.0 mL/min. UV data were collected at 207 nm. The content of echinoside A was determined from the peak area in the HPLC fingerprint and calculated from the linear regression equation obtained from the standard curve, indicating as the percentage in SC-3.
Diets and animals
High-fat (HF) diets (60% of calories derived from fat) and chow diets (10% of calories derived from fat) were obtained from Research Diets (D12492 and D12450B, New Brunswick). The animal experiments were approved by the Animal Care and Use Committee of Shanghai University of Traditional Chinese Medicine (approval no.: 2013016). Four-week-old female C57BL/6 mice (2013016) were purchased from the SLAC Laboratory (Shanghai, China). The mice were kept in a 12 h light/dark cycle at 22–23 °C. For 6-week preventive obesity experiments, the mice were randomly divided into four groups after a 1-week adaption period (n = 7). Groups were fed with HF diet, or chow diets, or HF diet plus 0.1% SC-3 (low-dose group), or HF diet plus 0.2% SC-3 (high-dose group). For the 2-week therapeutic obesity experiment, mice were fed with HF diet for 3 months, and then randomly divided into three groups (n = 7) and fed with HF diet or HF diet plus 0.1% SC-3 (low-dose group), or HF diets plus 0.2% SC-3 (high-dose group). The control mice were fed normal diet throughout the experimental period.
Analysis of fecal lipid content
Feces were collected at weeks 4, 5, and 6 of the preventive experiment. Fecal samples were pulverized, weighed, vortexed, and then extracted with methanol:chloroform (2:1, v:v) for 24 h at 4 °C. The organic phase was separated, dried at room temperature, and dissolved in 50 μL of isopropanol, and the samples were then analyzed using a Hitachi 7020 automatic analyzer (Hitachi, Tokyo, Japan) (Folch et al., Citation1957; Jung et al., Citation2006).
Intraperitoneal glucose tolerance test
At the end of the animal experiments, the mice were fasted for 10 h, and the blood glucose level was measured using the tail vein blood. Then, the glucose tolerance was evaluated using a glucose tolerance test, in which the mice received an intraperitoneal injection of glucose (1 g/kg/body weight). The blood glucose was measured at 15, 30, 60, and 90 min following the injection.
Analysis of serum chemicals
Heart blood was collected after the mice were fasted for 10 h. Serum samples were separated from the blood after centrifugation at 3000 rpm for 10 min. Serum triglyceride (TG), total cholesterol (TC), high-density lipoprotein cholesterol (HDL-c), and low-density lipoprotein cholesterol (LDL-c) were measured using a Hitachi 7020 automatic analyzer (Hitachi Ltd., Tokyo, Japan).
Analysis of the liver lipid content
The mice livers were collected, frozen in liquid nitrogen, and stored at −80 °C. The liver tissues were weighed, dissociated using ultrasound waves in a lysis buffer (20 mM Tris-HCl, 150 mM NaCl, 1% Triton; pH = 7.5), and extracted using the same volume of chloroform. The chloroform layer was dried, dissolved in isopropanol, and analyzed.
Morphological analyses of the abdominal white adipose tissue
The structure of the abdominal white adipose tissue (WAT) was analyzed using a scanning electron microscope. The adipose tissue was fixed with 10% neutral formalin and postfixed in 1% osmium tetroxide. Samples were determined using a Philip XL-30 scanning electron microscope (Philips, Eindhoven, Holland) with a magnifying power of 200× (Zhang et al., Citation2012a,Citationb).
Measurement of the inhibition of the activation of porcine PL
A solution was prepared using an enzyme buffer (10 mM Tris-HCl, pH = 8.0) and porcine PL. Hence, 0.1 mg PL was suspended in a buffer, centrifuged for 10 min at 1000 rpm, and the supernatant was used for the experiment. For each reaction system, 0.4 mg inhibitors (extracts from the 10 species of cucumber, Orlistatand echinoside A) were combined in a buffer, then 4-nitrophenyl butyrate (4-NPB, 0.06 mM) was added to this solution, vortexed for 10 s, incubated at room temperature for 10 min, and analyzed at 400 nm by using a BioTek plate reader (BioTek Instruments, Inc., Winooski, VT).
Enzyme inhibition kinetics were analyzed using a constant inhibitor concentration (SC-3: 0, 0.5, and 5 μg/mL; echinoside A: 0, 1, and 10 μM) and using five concentrations of 4-NPB (0–0.1 mM). The reaction was incubated at room temperature for 6 min and analyzed at 400 nm. The maximum velocity (Vmax) and the Michaelis–Menten constant (Km) were determined by plotting the initial velocity data against each concentration of 4-NPB (Grove et al., Citation2012).
Cell culture
HepG2 cells were cultured in a 12-well plate to 80% confluence in DMEM containing 10% fetal bovine serum (Hyclone, Logan, UT) at 37 °C in 5% CO2. SC-3 (2 mg/L) and echinoside A (2.5 μM) were added to the medium and the cells were incubated for 24 h.
Quantitative real-time polymerase chain reaction (PCR)
The total RNA from liver tissues and HepG2 cells was extracted using RNAiso Plus (Takara, Dalian, China) according to the manufacturer’s instructions. Genomic DNA contamination was removed using DNase I. The first cDNA strand was synthesized using a cDNA synthesis kit (Fermentas, Madison, WI). An ABI StepOnePlus Real-Time PCR system (Applied Biosystems, Waltham, MA) was used to analyze gene expression levels. The expression levels of all genes were normalized using β-actin as an internal control. The primers involved in this experiment are listed in and .
Table 1. Sequences of the primers used in real-time PCR (mice).
Table 2. Sequences of the primers used in real-time PCR (HepG2 cells).
Statistical analysis
The data are presented as mean ± SEM values. Data analysis was performed using SPSS12.0 for Windows (SPSS Inc., Chicago, IL), the data processing software. Statistical analysis was conducted using a one-way analysis of variance (ANOVA). A p value of <0.05 was considered statistically significant.
Results and discussion
In the present study, we observed that the sea cucumber extracts of P. graeffei reduced the body weight and serum lipids in the high-fat diet-fed mice. The enzymatic analysis showed that sea cucumber extracts and their major component echinoside A inhibit PL activity significantly. Furthermore, we observed that the P. graeffei extracts largely upregulated LXR-β and ABCA1 mRNA expression in the liver of obese mice.
T. anansa, P. graeffei, and H. fuscopunctata considerably inhibited PL activity
Sea cucumbers have been considered weight-reducing food in East Asian countries. However, the species of cucumbers that demonstrate these effects remain unclear. Therefore, we tested the PL-inhibitory effects of the saponin-enriched extracts that were prepared from 10 common edible sea cucumber species in vitro. As sea cucumber is considered weight-reducing food, we examined the role of its extracts in inhibiting the activity of PL, a vital enzyme in fat digestion, and as a drug target for obesity therapy (Anderson et al., Citation1967; Birari & Bhutani, Citation2007; Thomson et al., Citation1997). The enzymatic assay results showed that only the T. anansa, P. graeffei, and H. fuscoglive extracts largely inhibited PL activity, whereas the A. leucopraocta, A. leucospilota, H. pardalis, H. hilla, H. nobilis, S. chloronotus, and H. verrucosa extracts did not exert any effects on the PL activity in vitro, suggesting that some sea cucumbers may exhibit PL inhibiting activity and can be used in antiobesity therapy ().
Figure 2. Effects of the extracts of sea cucumbers on pancreatic lipase activity in vitro. The activities were compared with that of the vehicle control. Orlistat was used as a positive control with concentration of 1 μM. Concentrations for all extracts were 2.5 μg/mL. Values are mean ± SEM for five reactions in each group. *p < 0.01 versus control.
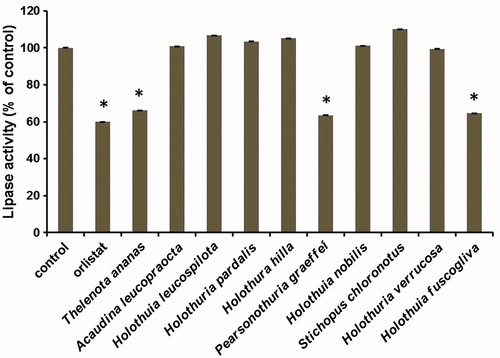
Characterization of SC-3 content
Next, we further analyzed P. graeffei extracts, which exhibited significant inhibitory effect on PL. The SC-3 extract was characterized using multiple methods. The extract comprised 65.98% saponins, 17.32% total polysaccharides, and 14.29% total amino acids.
LC–MS analysis showed that echinoside A, holothurin A, holothurin A1, and 24-dehydroechinoside A are the major saponins of SC-3 (). After the regression equations of the echinoside A standard were established, the echinoside A content in SC-3 was 35.62%.
SC-3 inhibits weight gain in C57BL/six mice
Sea cucumbers are rich in collagen, saponins, and polysaccharides (Abedin et al., Citation2013; Luo et al., Citation2013; Van Dyck et al., Citation2010). Saponins of sea cucumber have been reported to contain therapeutic properties and are used in treating various diseases such as cancer, hepatic steatosis and diabetes (Hu et al., Citation2012; Osbourn et al., Citation2011; Tian et al., Citation2013). Therefore, we investigated the role of SC-3 in reducing body weight by performing two sets of experiments on obese C57BL/six mice. C57BL/six mice can develop hyperlipidemia and hyperglycemia when fed a high-fat diet. In the preventive study, the mice were treated for 6 weeks. During treatment, the weight gain of the mice in the low-dose (0.1% SC-3) and high-dose (0.2% SC-3) groups were inhibited by 12.5% and 19.6%, respectively, and this difference was significant compared with that in the HF-fed mice (). However, the food intake amounts did not differ significantly among all of the groups ().
Figure 4. SC-3 affected body weight and white adipocyte in C57BL/six mice. Body weight gain (A) and food intake in preventive experiment (B). Body weight gain (C) and food intake in therapeutic experiment (D). The scanning electron microscope images of abdominal white adipocyte mouse from chow diets (E), HF (F), and 0.2% SC-3 (G) group. (H) The quantitation of adipocyte size. Values are mean ± SEM for seven mice in each group; # and ## for the low-dose group (0.1% SC-3) versus the HF-fed group (HF); * and ** for the high-dose group (0.2% SC-3) versus the HF-fed group (HF), * and # p < 0.05; ** and ## p < 0.01.
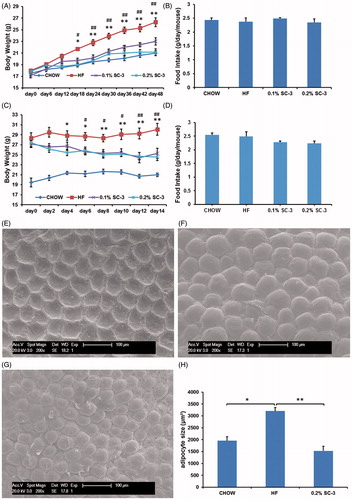
Next, we tested the therapeutic effects of SC-3 on the obese mice. After a 2-week treatment, both low and high doses of SC-3 effectively reduced the body weight of the obese mice (). The body weight decreased from 27.14 ± 0.67 g to 25.28 ± 0.99 g in the low-dose group, and 27.42 ± 0.84 g to 24.57 ± 0.75 g in the high-dose group although the food intake amounts did not differ significantly among the groups (). These data indicated that SC-3 can reduce weight and the inhibition of weight gain was not influenced by the food intake.
SC-3 inhibits the size of white adipocyte
Increased adipocyte size is one of the causes of obesity. Reducing the white-fat mass plays a crucial role in obesity therapy (Weisberg et al., Citation2003). Therefore, we observed changes in the WAT by using a scanning electron microscope. The adipocytes of mice in the high-dose group () were smaller than those of the mice in the HF-diet-fed group (), similar to that in the chow-diet-group (). Quantification data showed that the adipocyte size in SC-3-treated mice was 1528.14 μm2 compared with 3203.06 μm2 in the HF-fed group (). These results suggested that SC-3 inhibited the growth of white adipocytes, which may have resulted in weight reduction.
SC-3 improves glucose tolerance
To test the effects of SC-3 on blood glucose, fasting blood glucose and glucose tolerance tests were performed in preventive- and therapeutic-experiment mice. Compared with HF, a high dose of SC-3 considerably lowered the fasting blood glucose levels and the blood glucose level at 90 min after intraperitoneal glucose injection (1.0 g/kg) in preventive- and therapeutic-experiment mice (). A low dose of SC-3 exerted similar effects on the fasting blood glucose levels and glucose tolerance test, but no such effect was observed on the glucose level at 90 min after intraperitoneal glucose injection in the therapeutic experiment ().
Figure 5. SC-3 improved glucose tolerance, serum lipids and liver lipids. Fasting blood glucose levels (0 min) and glucose tolerance test (GTT), the mice were measured blood glucose after fasted 10 h as blood glucose levels of 0 min. Then, intraperitoneal inject glucose (1 g/kg) and blood glucose levels were tested at regular intervals of 15, 30, 60, and 90 min. (A) GTT test in preventive experiment; (B) GTT test in therapeutic experiment. (C) SC-3 reduced TC, TG, LDL-c, and HDL-c in serum in preventive experiment, heart blood was taken after fasting 10 h. (D) SC-3 reduced TC, TG, and LDL-c in serum in therapeutic experiment, heart blood was taken after fasting 10 h. The TC (E) and TG (F) in liver of the low-dose group and the high-dose group are less than the group of HF. Values are mean ± SEM for seven mice in each group; # and ## for the low-dose group (0.1% SC-3) versus the HF-fed group (HF); * and ** for the high-dose group (0.2% SC-3) versus the HF-fed group (HF), * and #p < 0.05; ** and ##p < 0.01.
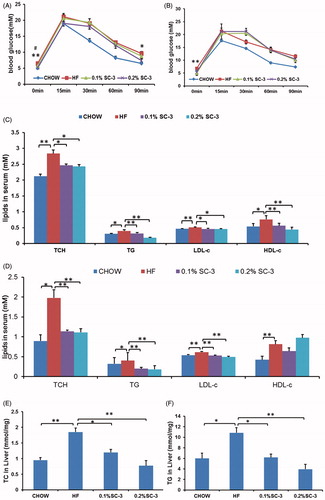
SC-3 reduced serum and liver lipids
We performed the serum lipid profile assays in the mice. We observed that SC-3 reduced the TC, TG, LDL-c, and HDL-c contents in the preventive experiment (), and TC, TG, and LDL-c contents in the therapeutic experiment (). Next, we analyzed the TG and TC contents in the livers and observed that the extracts significantly reduced the TC and TG contents in the livers (), suggesting that sea cucumber extracts ameliorated dyslipidemia in the HF-diet-fed mice. This finding is in agreement with those of Hu et al. (Citation2012), who reported that the saponins of P. graeffei ameliorates obesity, hepatic steatosis, and glucose intolerance.
SC-3 inhibits lipid absorption in the intestine
Because we observed that the sea cucumber extracts reduced the body weight in both preventive and therapeutic experiments, we supposed that the metabolic-disorder-ameliorating effects may result from weight control in the mice. To determine the underlying mechanism, we then analyzed the fecal lipids (TG and TC) to determine whether sea cucumber extracts inhibit lipid absorption in the intestines. Feces were collected at weeks 4, 5, and 6 of the preventive experiment. The results showed that the fecal TG and TC contents of the mice in the high-dose SC-3 group increased by 29.6% and 19.9%, respectively, compared with the controls (), suggesting that the weight-reducing effects of the sea cucumber extracts may have resulted from the inhibition of lipid absorption in the intestines of the mice. However, the fecal TC content increased by only 16.4% in the low-dose SC-3 group (); however, no such increase was observed in the TG content ().
Figure 6. SC-3 inhibits the activity of porcine pancreatic lipase. The contents of fecal TC (A) and TG (B). (C) Pancreatic lipase activity. The pancreatic lipase activity in vitro was determined using 4-nitrophenyl butyrate as substrate (4-NPB, 0.06 mM). The activity was compared with that of vehicle control. (D) Kinetic analysis. Three plots were analysis of vehicle control, 0.5 μg/mL and 0.5 μg/mL using 4-nitrophenyl butyrate (4-NPB, 0–0.1 mM). For (A) and (B), values were mean ± SEM for seven mice in each group. *p < 0.05; **p < 0.01. For (C) and (D), values were mean ± SEM for five reactions in each group.
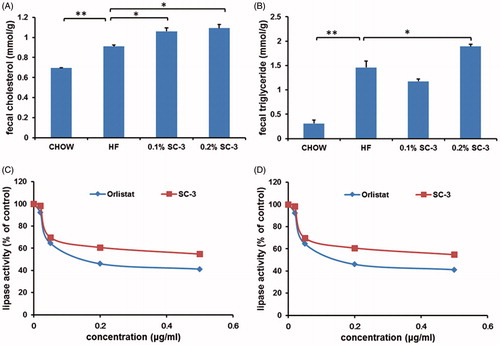
SC-3 and echinoside A inhibits PL activity
The weight-reducing strategies employed in this study were the inhibition of lipid absorption in the intestines and the suppression of calorie intake. Because the food intake amount was not changed by inclusion of the sea cucumber extract in diets, lipid absorption was tested for weight reduction. The absorption of lipids in the intestine was reduced when the PL activity was inhibited. We further analyzed the enzyme kinetics of PL and observed that SC-3 independently inhibited PL with an IC50 value at 2.86 μg/mL () compared with orlistat with an IC50 value at 0.47 μg/mL (). We then determined the inhibitory kinetics of SC-3 on 4-nitrophenyl butyrate (4-NPB) concentration (). Analysis of the Michaelis–Menten plot indicated that Vmax values were close in all groups treated with control (0.037 ± 0.001 μmol/L/min/mg PL), 0.5 μg/mL SC-3 (0.035 ± 0.001 μmol/L/min/mg PL), and 5 μg/mL SC-3 (0.037 ± 0.001 μmol/L/min/mg PL). The Km of the three groups were 0.003 ± 0.001 μmol/L (control), 0.014 ± 0.002 μmol/L (0.5 μg/mL SC-3), and 0.029 ± 0.003 μmol/L (5 μg/mL SC-3), and the Km increased with the increase in the SC-3 concentrations (p < 0.01). These results suggested that SC-3 competitively inhibits PL with respect to the substrate concentration.
Echinoside A is a major component of SC-3 () that was reported to inhibit the growth of hepatocellular carcinoma cells HepG2 and H22 (Zhao et al., Citation2012). We supposed that echinoside A inhibits PL activity. Therefore, we purified echinoside A and tested its activity. The test results indicated that echinoside A independently inhibited PL with an IC50 value at 0.76 μM (), and orlistat inhibited PL with an IC50 value at 0.61 μM (echinoside A/orlistst = 1.25, ). The inhibitory kinetics of echinoside A were determined with respect to 4-nitrophenyl butyrate concentration (). The Michaelis–Menten plot analysis indicated that the Vmax values were approximately equal in the control (0.051 ± 0.003 μmol/L/min/mg PL), 1 μM echinoside A (0.047 ± 0.006 μmol/L/min/mg PL), and 10 μM echinoside A (0.038 ± 0.003 μmol/L/min/mg PL) groups. The Km of the three groups were 0.014 ± 0.004 μmol/L (control), 0.032 ± 0.012μmol/L (1 μM echinoside A), and 0.054 ± 0.012 μmol/L (10 μM echinoside A), and the Km values increased proportionally with the increase in echinoside A concentrations (p < 0.01). These results suggest that echinoside A is a competitive inhibitor of PL with respect to substrate concentration and is likely responsible for the weight-reducing effects of sea cucumber extracts.
PL, also known as pancreatic triacylglycerol lipase, is a crucial enzyme in fat digestion (Lowe, Citation1997). PL can hydrolyze dietary fats and convert triglyceride substrates found in ingested oils to monoglycerides and free fatty acids (Crandall & Lowe, Citation2001). In the present study, SC-3 inhibited the PL activity and reduced the absorption of food lipids in the intestines. Further enzyme kinetic studies showed that SC-3 is a competitive inhibitor of PL and its efficiency was similar to that of orlistat. The data suggest that inhibition of the PL activity is the mechanism behind the weight-reducing effects of SC-3. Echinoside A is one of the major components of SC-3. We found that echinoside A inhibited PL activity and its inhibitory efficacy was similar to that of orlistat, indicating that echinoside A is a major effective component of sea cucumbers. Additional studies are warranted to verify the weight-reducing effects and to exclude the side effects of echinoside A in vivo.
Figure 7. Echinoside A inhibits the activity of porcine pancreatic lipase. (A) Structure of echinoside A. (B) Pancreatic lipase activity. The pancreatic lipase activity in vitro was determined using 4-nitrophenyl butyrate as substrate (4-NPB, 0.06 mM). The activity was compared with that of vehicle control. (C) Kinetic analysis. Three plots were analysis of vehicle control, 1 μM and 10 μM using 4-nitrophenyl butyrate (4-NPB, 0–0.1 mM). For (B) and (C), values were mean ± SEM for five reactions in each group.
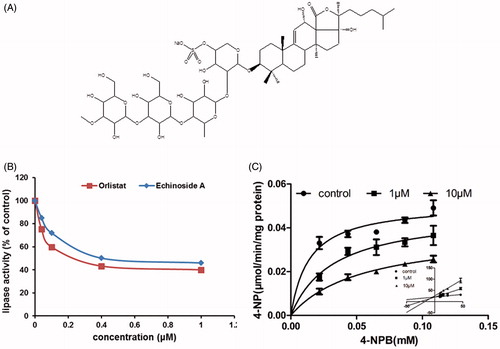
SC-3 upregulates the expression of LXR-β and ABCA1in mice
Since nuclear receptor transcription factors play crucial roles in lipid homeostasis, we analyzed the expression of the mRNAs of LXRs, peroxisome proliferator-activated receptors (PPARs), and their target genes in the liver of SC-3-treated mice. These results showed that the mRNA expression of LXR-β and its target gene, ATP-binding cassette transporter 1 (ABCA1) was upregulated, whereas those of PPARs, LXR-α, SREBP-1, Cy7PA1, FAS, and SCD-1 remained unchanged, suggesting that SC-3 may lower serum cholesterol through the upregulation of LXR-β signaling (). LXR-β regulates the expression of an array of genes in all aspects of cholesterol transport and metabolism (Baranowski, Citation2008). In addition, SC-3 increased the expression of the LXR target gene ABCA1 that facilitates cholesterol efflux from the cell membrane to its extracellular receptors (Oram, Citation2003; Sparrow et al., Citation2002). Thus, we propose that enhanced LXR-β signaling may be a partial mechanism of the hypocholesterol effects of SC-3.
Figure 8. Effects of samples on the relative mRNA expression levels on the liver of obese mouse and HepG2 cells. (A) The gene expression level in liver of SC-3-treated mouse was compared with that of HF control mouse. Values are presented as means ± SEM for six mice in each group. (B) The gene expression level of SC-3-treated HepG2 cells was compared with that of control. Values are presented as means ± SEM for four wells in each group. (C) The gene expression level of echinoside A-treated HepG2 cells was compared with that of control. Values are presented as means ± SEM for four wells in each group. β-Actin was used as an internal control. *p < 0.05, **p < 0.01.
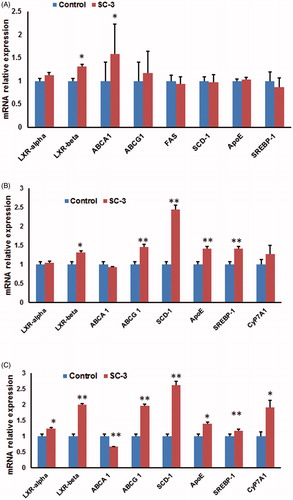
SC-3 and echinoside A upregulate the expression of LXR-β in HepG2 cells
To confirm the effects of SC-3 on LXR-β pathway, we tested the role of SC-3 in increasing the LXR-β expressions in human hepatoma HepG2 cells. SC-3 upregulated the expressions of LXR-β, ABCG 1, SCD-1, ApoE, and SREBP-1; however, those of LXR-α, ABCA 1, and CyP7A1 remained unchanged (). As echinoside A is a crucial component of SC-3, we further tested whether echinoside A increases the expression of LXR-β in HepG2 cells. Echinoside A upregulated the expression of LXR-α, LXR-β, ABCG 1, SCD-1, ApoE, SREBP-1, and CyP7A1 (). The results suggested that the upregulation of LXR-β and its target genes by SC-3 may be responsible for the lipid-lowering effects of sea cucumbers.
Recently many natural compounds have been identified as PL inhibitors. However, none of them have been approved as anti-obesity drugs yet. One of the reasons is that these natural products are low in abundance (Birari & Bhutani, Citation2007). Our data showed that sea cucumber saponins were PL inhibitors and rich in P. graeffei. It may provide a new source for the anti-obesity and hypolipidemic drug.
Conclusion
In conclusion, we observed that SC-3 reduced the body weight and suppressed the adipocyte size in HF-diet-fed C57BL/six mice. The extracts significantly inhibited lipid absorption in the intestines of the mice. Furthermore, we observed that SC-3 and its major saponin component echinoside A inhibited PL activity and increased the expression of LXR-β and ABCA1 in the mouse liver. Our data suggest that saponins in sea cucumbers can be used in diet therapy and represent a source for the development of novel antiobesity drugs.
Declaration of interest
The authors report that they have no conflicts of interest. This research program was supported by the National High Technology Research and Development Program of China (863 program) (2013AA093003) and the Program for Professors of Special Appointment (Eastern Scholar) in Shanghai Institutions of Higher Learning (2012-90).
References
- Abedin MZ, Karim AA, Ahmed F, et al. (2013). Isolation and characterization of pepsin-solubilized collagen from the integument of sea cucumber (Stichopus vastus). J Sci Food Agric 93:1083–8.
- Anderson RE, Bottino NR, Reiser R. (1967). Pancreatic lipase hydrolysis as a source of diglycerides for the stereospecific analysis of triglycerides. Lipids 2:440–2.
- Ballinger A, Peikin SR. (2002). Orlistat: Its current status as an anti-obesity drug. Eur J Pharmacol 440:109–17.
- Baranowski M. (2008). Biological role of liver X receptors. J Physiol Pharmacol 59:31–55.
- Beyea MM, Heslop CL, Sawyez CG, et al. (2007). Selective up-regulation of LXR-regulated genes ABCA1, ABCG1, and APOE in macrophages through increased endogenous synthesis of 24(S),25-epoxycholesterol. J Biol Chem 282:5207–16.
- Bohlooli S, Jafari N, Jahed S. (2012). Cytotoxic effect of freeze-dried extract of Ecballium elaterium fruit on gastric adenocarcinoma (AGS) and esophageal squamous cell carcinoma (KYSE30) cell lines. J Gastrointest Cancer 43:579–83.
- Birari RB, Bhutani KK. (2007). Pancreatic lipase inhibitors from natural sources: Unexplored potential. Drug Discov Today 12:879–89.
- Crandall WV, Lowe ME. (2001). Colipase residues Glu64 and Arg65 are essential for normal lipase-mediated fat digestion in the presence of bile salt micelles. J Biol Chem 276:12505–12.
- de la Garza AL, Milagro FI, Boque N, et al. (2011). Natural inhibitors of pancreatic lipase as new players in obesity treatment. Planta Med 77:773–85.
- Deters AM, Lengsfeld C, Hensel A. (2005). Oligo- and polysaccharides exhibit a structure-dependent bioactivity on human keratinocytes in vitro. J Ethnopharmacol 102:391–9.
- Drent ML, van der Veen EA. (1995). First clinical studies with orlistat: A short review. Obes Res 3:623S–5.
- Folch J, Lees M, Sloane Stanley GH. (1957). A simple method for the isolation and purification of total lipides from animal tissues. J Biol Chem 226:497–509.
- Gao Y, Islam MS, Tian J, et al. (2014). Inactivation of ATP citrate lyase by Cucurbitacin B: A bioactive compound from cucumber, inhibits prostate cancer growth. Cancer Lett 349:15–25.
- Grove KA, Sae-tan S, Kennett MJ, et al. (2012). (−)-Epigallocatechin-3-gallate inhibits pancreatic lipase and reduces body weight gain in high fat-fed obese mice. Obesity (Silver Spring) 20:2311–13.
- Han LK, Takaku T, Li J, et al. (1999). Anti-obesity action of oolong tea. Int J Obes Relat Metab Disord 23:98–105.
- Han LK, Zheng YN, Yoshikawa M, et al. (2005). Anti-obesity effects of chikusetsusaponins isolated from Panax japonicus rhizomes. BMC Complement Altern Med 5:9.
- Haslam DW, James WP. (2005). Obesity. Lancet 366:1197–209.
- Hsieh CJ, Wang PW, Liu RT, et al. (2005). Orlistat for obesity: Benefits beyond weight loss. Diabetes Res Clin Pract 67:78–83.
- Hu X, Li Z, Xue Y, et al. (2012). Dietary saponins of sea cucumber ameliorate obesity, hepatic steatosis, and glucose intolerance in high-fat diet-fed mice. J Med Food 15:909–16.
- Jiang HL, Niu JJ, Zhang WF, et al. (2014). The role of central nervous system on hypoglycemia and the feasibility of the brain theory in traditional Chinese medicine on treatment of diabetes mellitus. J Integr Med 12:1–6.
- Jung UJ, Lee MK, Park YB, et al. (2006). Effect of citrus flavonoids on lipid metabolism and glucose-regulating enzyme mRNA levels in type-2 diabetic mice. Int J Biochem Cell Biol 38:1134–45.
- Lowe ME. (1997). Structure and function of pancreatic lipase and colipase. Annu Rev Nutr 17:141–58.
- Luo L, Wu M, Xu L, et al. (2013). Comparison of physicochemical characteristics and anticoagulant activities of polysaccharides from three sea cucumbers. Mar Drugs 11:399–417.
- Ma X, Kundu N, Collin PD, et al. (2012). Frondoside A inhibits breast cancer metastasis and antagonizes prostaglandin E receptors EP4 and EP2. Breast Cancer Res Treat 132:1001–8.
- Masuko T, Minami A, Iwasaki N, et al. (2005). Carbohydrate analysis by a phenol-sulfuric acid method in microplate format. Anal Biochem 339:69–72.
- Melnikova I, Wages D. (2006). Anti-obesity therapies. Nat Rev Drug Discov 5:369–70.
- Oram JF. (2003). HDL apolipoproteins and ABCA1: Partners in the removal of excess cellular cholesterol. Arterioscler Thromb Vasc Biol 23:720–7.
- Osbourn A, Goss RJ, Field RA. (2011). The saponins: Polar isoprenoids with important and diverse biological activities. Nat Prod Rep 28:1261–8.
- Roh C, Jung U. (2012). Screening of crude plant extracts with anti-obesity activity. Int J Mol Sci 13:1710–19.
- Slanc P, Doljak B, Kreft S, et al. (2009). Screening of selected food and medicinal plant extracts for pancreatic lipase inhibition. Phytother Res 23:874–7.
- Sparrow CP, Baffic J, Lam MH, et al. (2002). A potent synthetic LXR agonist is more effective than cholesterol loading at inducing ABCA1 mRNA and stimulating cholesterol efflux. J Biol Chem 277:10021–7.
- Terasaka N, Hiroshima A, Koieyama T, et al. (2003). T-0901317, a synthetic liver X receptor ligand, inhibits development of atherosclerosis in LDL receptor-deficient mice. FEBS Lett 536:6–11.
- Thomson AB, De Pover A, Keelan M, et al. (1997). Inhibition of lipid absorption as an approach to the treatment of obesity. Methods Enzymol 286:3–44.
- Tian X, Tang H, Lin H, et al. (2013). Saponins: The potential chemotherapeutic agents in pursuing new anti-glioblastoma drugs. Mini Rev Med Chem 13:1709–24.
- Van Dyck S, Gerbaux P, Flammang P. (2010). Qualitative and quantitative saponin contents in five sea cucumbers from the Indian ocean. Mar Drugs 8:173–89.
- Weisberg SP, McCann D, Desai M, et al. (2003). Obesity is associated with macrophage accumulation in adipose tissue. J Clin Invest 112:1796–808.
- Wijesinghe WA, Jeon YJ, Ramasamy P, et al. (2013). Anticancer activity and mediation of apoptosis in human HL-60 leukaemia cells by edible sea cucumber (Holothuria edulis) extract. Food Chem 139:326–31.
- Zhang B, Xue C, Hu X, et al. (2012a). Dietary sea cucumber cerebroside alleviates orotic acid-induced excess hepatic adipopexis in rats. Lipids Health Dis 11:48.
- Zhang Y, Fan S, Hu N, et al. (2012b). Rhein reduces fat weight in db/db mouse and prevents diet-induced obesity in C57Bl/6 mouse through the inhibition of PPARgamma signaling. PPAR Res 2012:374936.
- Zhao HL, Kim YS. (2004). Determination of the kinetic properties of platycodin D for the inhibition of pancreatic lipase using a 1,2-diglyceride-based colorimetric assay. Arch Pharm Res 27:1048–52.
- Zhao Q, Xue Y, Wang JF, et al. (2012). In vitro and in vivo anti-tumour activities of echinoside A and ds-echinoside A from Pearsonothuria graeffei. J Sci Food Agric 92:965–74.
- Zheng Q, Koike K, Han LK, et al. (2004). New biologically active triterpenoid saponins from Scabiosa tschiliensis. J Nat Prod 67:604–13.