Abstract
Objectives. Electrocautery is an appreciated surgical tool, which however, generates immense heat and fat-tissue melting. In cardiac surgery, liquefied fat collects on the surface of blood in the pericardial cavity and becomes aspirated by the heart-lung machine for aortic recycling. Deposits seen in the brain microcirculation after surgery are caused by lipid embolism. This study investigates lipid chemistry, whether heat from electrocautery generates fatty-acid fragmentation and decomposition. Design. Pericardial fat tissue was sampled from cardiac-surgery patients and from piglets. The human tissue was exposed to electrocautery, or to fixed temperatures in an in vitro model. Fatty-acid decomposition was explored by solid-phase microextraction gas chromatography and the distribution of fatty acids was measured. Results. Fatty-acid decomposition demonstrated a temperature-effect relationship (p=0.007). At 350°C the proportion of polyunsaturated fatty acids became heavily reduced or were abolished (p=0.016). Electrocautery resulted in similar changes. Conclusions. Electrocautery induces a profound fatty-acid fragmentation to form short-chained compounds. The chemical and toxic nature of these compounds remains to be determined, including their clinical implications at blood recycling in cardiac surgery.
Neurocognitive decline is a complication to cardiac surgery, with variable reported rates of between 3% and 90% depending on observational methods (Citation1). One of several discussed mechanisms refers microembolization of contaminating lipids in recycled pericardial suction blood (PSB) (Citation2). Lipid deposits were found in cerebral vessels in patients who died shortly after cardiac surgery, described as ‘small capillary arteriolar dilations’ (Citation2).
It is obvious to the surgeon that electrocautery melts fat tissue. Another source of lipids is from exposed sternal bone marrow. Lipids in PSB are known to primarily consist of triglycerides (Citation3), and reflect the basic components of adipose tissue. Lipids accumulate on the surface of blood in the pericardial cavity, a phenomenon clearly observed at surgery. In the past, PSB was separated from direct return because of profound neurological complications that was attributed to lipid embolism (Citation4), whereas with modern technology the convenience of PSB recycling is again appreciated. Although improved, the routine cardiopulmonary bypass equipment of today is inadequate in its clearing of contaminating lipids, with only about 45% reduction rate (Citation5).
Electrocautery generates considerable heat with temperatures known to exceed 350°C (Citation6). Smoke formation may pose a health risk to exposed professionals (Citation7). From a technical perspective heat is a well known method to fragment molecules in the petroleum industry (e.g. thermal cracking). Also, heat-induced decomposition and oxidation of unsaturated fatty acids (FA) may affect the safety of lipid-containing food (Citation8–11). It is plausible to assume that similar chemical fragmentation and oxidation occur in response to electrocautery. This hypothesis was here elucidated by exposing human pericardial fat tissue to electrocautery or fixed temperatures in an in vitro model. The composition of FA was analyzed by gas chromatography, and their decomposition products were explored by headspace solid-phase microextraction gas chromatography (SPME-GC).
Materials and methods
Fat sampling
Pericardial fat tissue that had been cut away to gain access for the left internal mammary artery was collected from three male patients undergoing routine cardiac surgery, 67.7 ± 2.9 years of age. The patients had signed a written consent and the study was approved by the local ethics committee. Tissue samples weighed on average 10.6 ± 1.8 g and were kept frozen until analysis. Animal tissue was collected from four standard breed-type piglets. The pericardium and heart were immediately retrieved after slaughter from which representative samples were cut out and frozen. Live animals were not handled within the study. Animal-derived fat allowed larger amounts of tissue for testing of the experimental design prior to human-fat analyses.
Electrocautery exposure
A portion of each human fat sample was exposed to electrocautery in an in vitro model. A Valleylab Force-2 device (Valleylab Boulder, Colorado, USA) with a standard metal blade tip was used. The energy was set at ‘program-3’ and ‘coagulation-40’ which was identical to that used during routine harvesting of the internal mammary artery. The fat sample was positioned on a stainless steel bed that served as ground electrode. The bed was curved and sloped so that liquid fat accumulated at its base and dripped into a 10 ml glass tube.
Fixed-temperature exposures
The remaining human tissue sample was subdivided into three equal portions, of which one served as room-temperature control as described below. The tissue was exposed to fixed temperatures of 200°C and 350°C. Animal fat was handled in a corresponding way but with an extended temperature series: 100°C, 200°C and 400°C. At 100°C only minor chemical changes were detected and at 400°C the fat was close to its flame point, which explains the difference to how the human fat was exposed. For both types of fat, the tissue was slowly heated until 100°C to allow water evaporation during 10 min, followed by additional heating to reach the individual target temperature. A digital temperature-log (Testo 175-T3, Testo AG, Lenzkirch, Germany) monitored the exposure. The target temperature was held for 5 min and was then allowed to cool to room temperature. The liquid fat portion was decanted to test tubes and refrozen until further analysis. The fat used as control was derived by chloroform extraction at room temperature. The solvent was then evaporated under nitrogen.
FA decomposition
Decomposition and oxidation products were determined by SPME-GC. In principle, with this technique volatile compounds are collected on a thin fiber tip and analyzed in a gas chromatograph. Reference is made to chemistry science for further information (Citation12). In brief, an 85 µm SPME fiber (Carboxen/polydimethylsiloxane, Supelco, Bellefonte, PA, USA) was selected and used together with a gas chromatograph (HP GC 5890 series II) equipped with a SPB-1000 capillary column (30 m x 250 µm ID x 0.25 µm film thickness, Supelco, Bellefonte, PA, USA). The helium-carrier gas flow was 0.8 ml/min in splitless mode. The gas chromatograph temperature settings were; injector 280°C, flame-ionization detector 250°C, and the program used an initial 40°C held for 2 min followed by 5°C/min increase to 100°C and 10°C/min increase towards the final temperature of 195°C held for 8 min. The gradual heating is expressed by the term “retention time”.
FA composition
For FA measurement, the control and heat-extracted fat were subjected to acidic hydrolysis and transmethylation, and the FA methyl esters were separated by gas-liquid chromatography as previously described (Citation13).
Statistics
For multiple group comparisons Friedmans ANOVA was applied. The concordance in reaction of polyunsaturated FA, at fixed temperature or electrocautery exposures, was analyzed by sign test probability. Mean values ± sem are given throughout. A p-value less than 0.05 was considered significant. SPSS version 18 was used (SPSS Inc., Chicago, IL, USA).
Results
SPME-GC
Electrocautery gave a substantial change of the SPME-GC chromatogram compared with control human fat (). In the model of fixed temperature exposure, decomposition was evident at 200°C and became more pronounced at 350°C. The effect of electrocautery expressed an apparent left-shift of peaks towards early SPME-GC retention times compared with the fixed exposure to 350°C (). The peak at 12 min retention time seen in all panels of refers to the internal standard (see Materials and methods). The formed peaks express volatile short-chained molecules derived from FA decomposition and oxidation whereas FA are not detected by this method.
Figure 1. Example of a SPME-GC chromatogram of human pericardial fat tissue that had been exposed to fixed temperatures or electrocautery, as indicated. Each peak indicates a volatile compound derived from FA decomposition and the amplitude its relative amount. The horizontal axis represents the retention time, during which the sample is gradually heated to allow evaporation of specific compounds. Compounds that evaporate at lower temperatures are seen to the left in each panel. Less volatile compounds require a higher temperature which is reached later during the run and are seen to the right along the retention-time axis. The peak at 12-min retention time refers to the internal standard used for output-signal calibration. Please, see Materials and Methods for further details.
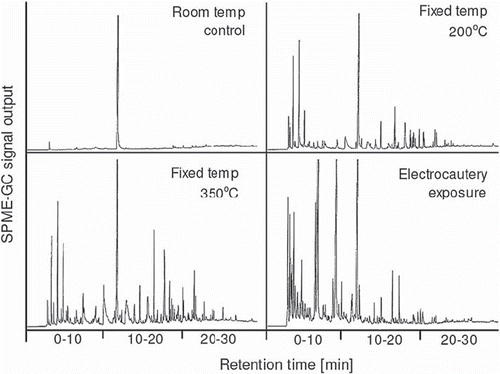
In the pig model, lipids appeared chemically stable at 100°C, whereas signs of FA decomposition were observed at 200°C. In the number of peaks of the SPME-GC chromatograms, similar to those of human fat in , were counted and subdivided in retention-time intervals. At 200°C the peaks formed a pattern with a predominant increase in short retention times, illustrating the decomposition into low molecular-size compounds which evaporate at low temperatures. The overall difference between retention-time groups was expressed by p=0.022 (Friedmans ANOVA). At 400°C the decomposition increased over the entire spectrum of retention times. At this temperature the flame-point was nearly reached. The overall difference between temperatures was associated with p=0.007 (Friedmans ANOVA).
Figure 2. Peak counts on the SPME-GC chromatogram for pig fat exposed to different temperatures. The experiments are similar to the example shown for human fat in . For numeric data extraction the chromatograms were subdivided into three retention-time windows; 0–10, 10–20, and 20–30 min. The number of identifiable peaks was counted within each window. The peak referring to the internal standard at 12-min retention time was disregarded. A high count indicates a large number of compounds. Mean ± sem values, n=4. Please, see the legend to for explanatory details.
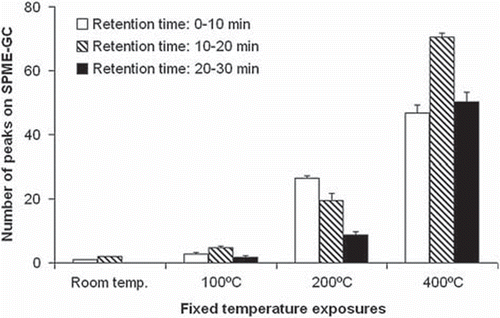
FA analysis
When human fat tissue was exposed to electrocautery the distribution of FA changed in relation to their chemical structure. For individual FA no significant change was statistically confirmed due to few observations. However, for polyunsaturated FA as a group, electrocautery caused a consistent reduction seen for all seven of the individual FA analyzed (p=0.016, Sign test probability, ).
Table I. FA composition of human pericardial fat and the response to electrocautery.
The phenomenon observed with electrocautery was confirmed in the fixed-temperature model of human fat tissue (, upper panel). At 200°C all polyunsaturated FA were reduced compared to that at room temperature. At 350°C they were further reduced or had disappeared below detection level (p=0.016, Sign test probability), similar to that observed for electrocautery. There was also a small increase in the pool of saturated palmitic acid (16:0).
Figure 3. Fatty-acid (FA) proportions in pericardial tissue from humans and pigs in response to different temperature exposures, and with subdivision into FA saturation levels, as indicated. The data describe the overall FA composition of the sample. Fat samples were pooled for analysis, with n=3 and n=4 for human and pig tissue, respectively.
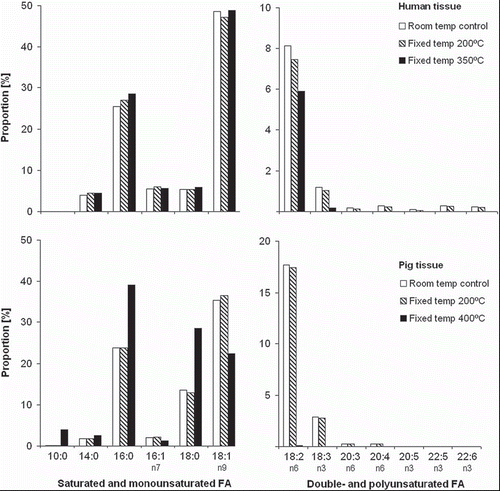
For pig pericardial fat tissue no obvious alterations were observed at 200°C (, lower panel). However, at the peak temperature of 400°C all polyunsaturated FA had disappeared and there was a relative increase in many saturated FA, in particular palmitic (16:0) and linoleic acid (18:0). Furthermore, the short-chained saturated FA capric acid (10:0) was formed.
Discussion
The present study tested the hypothesis that FA decomposition and oxidation occurs when pericardial fat tissue is exposed to heat and electrocautery. This is of particular importance in cardiac surgery as PSB is found to be heavily contaminated by lipids (Citation14). The blood is returned to the systemic circulation via the cardiotomy filtration, although the majority of contaminating lipids are known to pass the filter (Citation5). Lipid deposits have been demonstrated in the cerebral microcirculation with a confirmed association with PSB recycling (Citation2). The lipids, known to consist of triglycerides (Citation3), partly originate from the use of electrocautery and fat-tissue melting.
Our results confirm what is chemically anticipated. At high temperatures FA becomes unstable and polyunsaturated FA are more vulnerable in this respect, a reactivity which is due to their double bindings. This phenomenon was clearly observed in this study, as the polyunsaturated FA decomposed to form shorter molecules. The SPME-GC chromatograms showed a vast variety of volatile compounds, demonstrated for both human and pig pericardial tissue. The decomposition of polyunsaturated FA required a temperature of at least 200°C. At the tested peak temperatures most of the polyunsaturated FA disappeared or were below detectable levels. Electrocautery produced a similar phenomenon although the polyunsaturated FA were not completely abolished. Electrocautery can be assumed to produce variable temperatures depending on the distribution of currents and heat in the tissue and the distance from the cutting knife. Sparks and charcoal formation reveals temperatures far above those tested in the fixed temperature models. The SPME-GC chromatograms indicated a substantial pool of compounds with early retention time characteristics suggesting short-chained molecules. This pattern can be presumed to reflect extreme heat. At room temperature the triglycerides of pericardial fat tissue were without any signs of volatile compounds.
The food industry is well aware of the potential risks in handling fat, especially if it contains polyunsaturated FA. Of particular interest is the risk associated with FA peroxidation, which occurs during heating in the presence of oxygen. Oxygen radicals are biologically reactive and are known to cause cellular damage (Citation9–11). However, the toxic impact of electrocautery-induced changes can only be speculated upon. At present, the primary concerns regarding electrocautery seem to address the fumes to which health professionals are exposed, with identified volatile compounds (Citation7). Electrocautery is a widely used tool in surgery. Presumably, chemically modified lipids may have local adverse effects at wound healing. In cardiac surgery an additional dimension is addressed, from the aortic recycling of lipid-contaminated PSB and embolism. Therefore, the issue of cerebral lipid embolization may extend beyond the effect of a mere mechanical vessel obstruction to also include chemical implications.
This study is limited from its in vitro design, which however, is a requisite in view of the technical aim of identifying FA decomposition in response to electrocautery. The mechanism was confirmed by fixed temperature models. In these analyses, because of the required fat amount, fat was not collected and isolated from PSB during surgery. Also, the number of subjects was few and the statistical power was hence limited. Our study was restrained in identifying the phenomenon of FA decomposition only. The produced compounds were not chemically characterized, nor were their possible toxic effects addressed.
It is concluded that electrocautery-derived heat results in a pronounced FA decomposition. Short-chained compounds are formed, the nature of which is not known at present. It is difficult to have an opinion about the clinical relevance of our finding and many questions remain to be answered. Nevertheless, the issue of PSB handling during cardiac surgery may deserve attention.
Acknowledgements
The authors are very grateful to Siv Tengby for invaluable help with the FA analyses, and to Eva Sejby, Barbro Simu and Bengt Vessby for expert assistance. This study had support from the Medical Faculty at Umea University and the Heart Foundation of North Sweden. The study had no support from any commercial funds.
Declaration of interest: The authors report no conflicts of interest. The authors alone are responsible for the content and writing of the paper.
References
- van Dijk D, Keizer AM, Diephuis JC, Durand C, Vos LJ, Hijman R. Neurocognitive dysfunction after coronary artery bypass surgery: A systematic review. J Thorac Cardiovasc Surg. 2000;120:632–9.
- Brown WR, Moody DM, Challa VR. Cerebral fat embolism from cardiopulmonary bypass. J Neuropathol Exp Neurol. 1999;58:109–19.
- de Vries AJ, Gu YJ, van Oeveren W. The rationale for fat filtration during cardiac surgery. Perfusion. 2002;17:29–33.
- Caguin F, Carter MG. Fat embolization with cardiotomy, with the use of cardiopulmonary bypass. J Thorac Cardiovasc Surg. 1963;46:665–72.
- Jewell AE, Akowuah EF, Suvarna SK, Braidley P, Hopkinson D, Cooper G. A prospective randomised comparison of cardiotomy suction and cell saver for recycling shed blood during cardiac surgery. Eur J Cardiothorac Surg. 2003;23: 633–6.
- Kinoshita T, Kanehira E, Omura K, Kawakami K, Watanabe Y. Experimental study on heat production by a 23.5-kHz ultrasonically activated device for endoscopic surgery. Surg Endosc. 1999;13:621–5.
- Moot AR, Ledingham KM, Wilson PF, Senthilmohan ST, Lewis DR, Roake J, . Composition of volatile organic compounds in diathermy plume as detected by selected ion flow tube mass spectrometry. ANZ J Surg. 2007;77:20–3.
- Sanchez-Muniz FJ. Oils and fats: Changes due to culinary and industrial processes. Int J Vitam Nutr Res. 2006;76: 230–7.
- Frankel EN. Lipid oxidation. The Oily Press LTD: Dundee, Scotland; 1998.
- Nair U, Bartsch H, Nair J. Lipid peroxidation-induced DNA damage in cancer-prone inflammatory diseases: A review of published adduct types and levels in humans. Free Radic Biol Med. 2007;43:1109–20.
- Indart A, Viana M, Clapés S, Izquierdo L, Bonet B. Clastogenic and cytotoxic effects of lipid peroxidation products generated in culinary oils submitted to thermal stress. Food Chem Toxicol. 2007;45:1963–7.
- Mortensen G, Sorensen J, Stapelfeldt H. Light-induced oxidation in semihard cheeses. Evaluation of methods used to determine levels of oxidation. J Agric Food Chem. 2002;50: 4364–70.
- Boberg M, Vessby B, Croon LB. Fatty acid composition of platelets and of plasma lipid esters in relation to platelet function in patients with ischaemic heart disease. Atherosclerosis. 1985;58:49–63.
- Appelblad M, Engström KG. Fat content in pericardial suction blood and the efficacy of spontaneous density separation and surface adsorption in a prototype system for fat reduction. J Thorac Cardiovasc Surg. 2007;134:366–72.