Abstract
The prevalence of obesity is increasing at an alarming rate, but, unfortunately, only a few drugs are currently available on the market. In the present study, the methanolic extract of Ginkgo biloba L. (Ginkgoaceae) was investigated as an inhibitor of pancreatic lipase (PL) in an attempt to explain its hypolipidaemic activity. In vitro assay of G. biloba leaves extract revealed a substantial PL inhibition activity (IC50 = 16.5 µg/mL). Further investigation was performed by employing theoretical docking simulations and experimental testing to uncover the active constituents responsible for G. biloba anti-lipase activity. Virtually, terpene trilactones, including ginkgolides and bilobalide, were found to fit within the binding pocket of PL via several attractive interactions with key amino acids. Experimentally, ginkgolides A, B, and bilobalide were found to inhibit PL significantly (IC50 = 22.9, 90.0, and 60.1 µg/mL, respectively). Our findings demonstrated that the hypolipidaemic effects of G. biloba extract can be attributed to the inhibition of PL by, at least in part, terpene trilactones. In conclusion, this work can be considered a new step towards the discovery of new natural safe hypolipidaemic PL inhibitors.
Abbreviations | ||
PL | = | Pancreatic lipase |
GBE | = | Ginkgo biloba extract |
TTLs | = | terpene trilactones |
PNPB | = | p-nitrophenyl butyrate |
Introduction
Obesity is a worldwide problem, which is rapidly affecting both developed and developing countries. According to a recent report from the World Health Organization, it is estimated that worldwide >1 billion adults are overweight, at least 300 million of them clinically obese [Citation1,Citation2]. Almost two-thirds of the American adults [Citation3,Citation4], 60% of the English people above 16 years [Citation5], and 60% of Australians above 25 years [Citation6] are overweight or obese. Overweight and obesity are important risk factors for the development of diabetes mellitus, coronary heart diseases, hypertension, hyperlipidaemia, arteriosclerosis, and other chronic diseases [Citation7,Citation8]. Reversing the current trends of increasing overweight and obesity prevalence is one of the targets of health policy in many countries [Citation9].
Current guidelines indicate that a combination of dietary therapy, physical activity, and behavioural therapy are effective strategies for weight loss and weight management [Citation10]. However, despite the national goals promoting diet and lifestyle, the obesity problem in the United States has doubled since 1980. Clearly, additional medications are required to fulfil this need in the treatment of obesity.
Several strategies have been implemented to find treatments for the critical problem of obesity. One therapeutic approach for the prevention of obesity is to retard the absorption of fatty acid by the inhibition of lipase in the digestive organs [Citation11,Citation12]. Pancreatic lipase (triacylglycerol acyl hydrolase, PL), which catalyzes the hydrolysis of triacylglycerides in the gastrointestinal tract, is the key enzyme for lipid absorption. It is responsible for the hydrolysis of 50–70% of total dietary fats. This enzyme is composed of single-chain glycoprotein of 449 amino acids, which divided into two folding units, the larger N-terminal domain and a C-terminal domain [Citation13,Citation14]. The N-terminal domain is the catalytic domain and the C-terminal domain binds the colipase, a cofactor required for activity [Citation13,Citation14]. PL inhibition is one of the most widely studied mechanisms for the determination of the potential efficacy of natural products as anti-obesity agents [Citation15]. Orlistat, a hydrogenated derivative of lipstatin derived from Streptomyces toxytricini, is a potent inhibitor of PL and one of the best-selling drugs worldwide [Citation16]. The drug has proved to be effective for the treatment of obesity, but side effects due to its potency, such as faecal incontinence, have also arisen [Citation17]. The success of orlistat has prompted research for the identification of new PL inhibitors derived from natural products, such as Anthemis palaestina Boiss., Ononis natrix L. [Citation18], Sapindus rarak DC. [Citation19], and oolong tea [Citation20]. In the course of a search for natural PL inhibitors from herbal medicines [Citation18], the leaves of Ginkgo biloba L. (Ginkgoaceae) was chosen for more detailed investigation, since the plant extract showed a fat mass reduction, hypolipidaemic effect, and a potential weight reduction effect [Citation21–25].
G. biloba has been used for medical purposes for centuries in traditional Chinese medicine. The standard extracts of G. biloba leaves are now more commonly used as dietary supplements or phytomedicines in Western countries. Both experimental and clinical studies have verified the cerebrovascular, cardiovascular, and neuroprotective effects of G. biloba extract (GBE) [Citation26,Citation27]. Currently, the most common clinical uses of GBE include vascular dementia, Alzheimer’s disease, memory enhancement, intermittent claudication, and tinnitus of vascular origin [Citation28]. The extract contains large number of active compounds, the most important of which are terpene trilactones (TTLs) and flavonol glycosides [Citation28]. The mixture of biologically active ingredients in GBE accounts for the pleiotropic effects, including antioxidant effects [Citation29], inhibition of platelet aggregation and thromboxane B2 production [Citation30], vasodilation [Citation31], and modulation of cholesterol metabolism [Citation32]. The active ingredients of the mixture, such as the flavonoids, have antioxidant properties, prevent stroke and transient ischemic attack, and inhibit membrane lipid peroxidation [Citation33]. In addition, ginkgolide B has found to be a potent inhibitor of platelet-activating factor [Citation34]. Recently, it has been found that the alcoholic leave extract of G. biloba has fatty acid synthase inhibition activity [Citation21]. Fatty acid synthase enzyme is reported as a potential new therapeutic target for the treatment of obesity [Citation21].
The fat mass reduction and hypolipidaemic activities observed for G. biloba prompted us to evaluate its potential PL inhibitory activity and to find the active components that could directly bind and inhibit PL. Findings from this study should help in understanding the mechanism of action of G. biloba as hypolipidaemic agent; furthermore, it should provide new insights towards the discovery of new natural safe PL inhibitors. The current study commenced by computer-aided molecular docking of TTLs, one of the major constituents of the plant, into the binding pocket of PL in order to reach to preliminary conclusions about terpenes/PL-binding energetics. Eventually, the leaves extract and the docked active components were tested in vitro against PL to evaluate their inhibition potential.
Material and methods
Materials
All of the chemicals used in these experiments were of reagent grade and obtained from the following sources: porcine PL type II; Tris–HCl buffer; orlistat; ginkgolide A; ginkgolide C; bilobalide; and p-nitrophenyl butyrate (PNPB) from Sigma (St. Louis, MO); acetonitrile and methanol from Merck (Whitehouse Station, NJ).
Molecular modelling
Software and hardware: The following software packages were utilized in the present research.
CS ChemDraw Ultra 7.01, Cambridge Soft Corp. (http://www.cambridgesoft.com), Cambridge, USA
OMEGA (Version 2.3.2), OpenEye Scientific Software (www.eyesopen.com), USA [Citation35]
FRED (Version 2.1.5), OpenEye Scientific Software, (www.eyesopen.com), Santa Fe, USA [Citation36]
DS visualizer 2.0, Accelrsy Inc. (www.accelrys.com), San Diego, USA
Docking experiment
The chemical structure of TTLs (ginkgolides A, B, C, J, K, L, M, and bilobalide, ) was sketched in Chemdraw Ultra (7.01) and saved in MDL molfile format. Subsequently, an ensemble of energetically accessible conformers was generated using OMEGA2 software [Citation35]. OMEGA builds initial models of structures by assembling fragment templates along sigma bonds. Conformations for the fragments are recovered from pre-generated libraries within the software. Once an initial model of a structure is built, OMEGA generates additional models by enumerating ring conformations and invertible nitrogen atoms. The generated conformers are saved in SD format.
Docking simulations
The 3D coordinates of PL were retrieved from the Protein Data Bank (PDB code: 1LPB, resolution; 2.46 Å) [Citation37]. Hydrogen atoms were added to the protein using the DS visualizer templates for protein residues. The protein structure was utilized in subsequent docking experiments without energy minimization. The docking study was conducted in the presence of crystallographically explicit water molecules. The drug was docked into the binding site of PL employing FRED software [Citation36], which takes a multiconformer database of one or more ligands, a target protein structure, a box defining the active site of the protein based on the co-crystallized ligand and several optional parameters as input. The ligand conformers and protein structure are treated as rigid during the docking process. FRED’s docking strategy is to exhaustively score all possible positions of each ligand in the active site [Citation36]. The conformational ensemble of the terpenes trilactone generated using OMEGA software was used as input in the FRED software. We employed the docking settings that succeeded in reproducing the experimental pose of the co-crystallized ligand (CllP; ) [Citation37]. Accordingly, the following FRED parameters were employed:
Addbox: An optional parameter that adjusts the geometry of the box defining the active site by extending each edge of the box by the specified number of Angstroms. The binding site in the current docking experiment was generated from the co-crystallized ligand with the targeted protein. The box defining the active site was expanded to 2Å.
Num_poses: This parameter specifies the number of poses to be returned by the exhaustive rigid body search. In the current docking experiment, the number of poses to be returned by exhaustive search was set to the maximum value of 1000.
Num_alt_poses: Only the top-ranking poses will be selected from the list and scored by the scoring functions specified by the user. This flag specifies how many alternate poses, in addition to the top pose, will be presented for consensus scoring. In the current experiment, the number of alternative poses to be retrieved was set to 3.
The docked poses were scored by the Chemgauss2 scoring function and the highest ranking poses were retained for evaluation. Chemgauss2 scoring function represents all atoms as smooth Gaussian functions. Hydrogen bonding interactions are the most significant chemical potentials accounted for by the Chemgauss2 scoring function. The Chemgauss2 function is the sum of the following potentials, all of which are based on smooth Gaussian functions: (1) Shape-based interactions between all heavy atoms. (2) Hydrogen bonding interactions based on favourable interactions between polar hydrogens and lone pairs and a mild repulsion between donor heavy atoms and acceptors (which tends to make the hydrogen bonds linear). (3) Aromatic ring interactions based on favourable interactions between aromatic atoms and the π-electron positions plus repulsive aromatic atom to aromatic atom and π-electron to π-electron interactions [Citation36].
Figure 2. (A) Comparison between the docked pose (green) of the ligand CllP as produced by docking simulation and the crystallographic structure of this ligand (red) within the binding pocket of PL. (B) The solvent accessible surface area of the binding site of PL (1LPB) and the co-crystallized inhibitor (CllP).
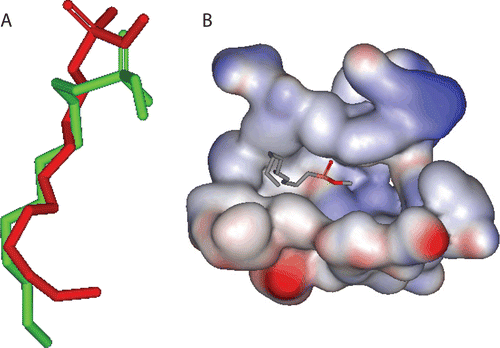
Plant material
The plant materials were collected from G. biloba species cultivated in the northern Jordan and identified by Dr. K. Tawaha, Department of Pharmaceutical Sciences, Faculty of Pharmacy, Jordan University, Jordan. A voucher specimen has been deposited at the Department of Pharmaceutical Sciences/Faculty of Pharmacy, University of Jordan. The leaves of this plant were cleaned of residual soil and air-dried at room temperature. The dried leafy parts were minced to a fine powder using a laboratory mill and passed through a 24-mesh sieve to generate a homogeneous powder, stored at room temperature (22–23°C), and protected from light until use [Citation18].
Plant extraction
Methanolic extractions were conducted using 1 g aliquot of the plant material in 40 mL methanol (80%) at 37°C for 3 h in a shaking water bath. After cooling, the extract was centrifuged at 1507g for 10 min, and the supernatant was recovered. The solvent was evaporated under vacuum at 40°C using a rotary evaporator. The solid residues were collected and stored in dry condition until analysis.
Preparation of compounds and extract for in vitro assay
The tested pure compounds or extract were initially dissolved in DMSO to give seven different stock solutions with a concentration range of 0.078–5.00 mg/mL. Subsequently, 20 µL of each stock solution was used to give final concentration range of 1.56–100 µg/mL in the reaction mixture.
PL inhibition assay
The PL activity was quantified by a colorimetric assay that measures the release of p-nitrophenol as previously described [Citation18,Citation38–40] with minor modification. The enzyme solutions were prepared immediately before use. Crude porcine PL type II (Sigma, EC 3.1.1.3) was suspended in Tris–HCl buffer (2.5 mmol, pH 7.4 with 2.5 mmol NaCl) to give a concentration of 5 mg/mL (200 units/mL) and mixed using a stirrer for 15 min. The solution was then centrifuged at 1500g for 10 min and the clear supernatant was recovered. The 0.10 mL of PL solution was preincubated with different concentrations (1.56–100 µg/mL) of the extract and the selected compounds for 5 min at 37°C, then the PNPB substrate (10 mM in acetonitrile) was added. The volume was diluted to 1 mL using the Tris–HCl buffer before measuring the solution absorbance spectrophotometrically at 410 nm at least five time points: 1–5 min. The release of p-nitrophenol is measured as the increase in absorbance at 410 nm against blank using denaturated enzyme. The PL activity is related to the rate of p-nitrophenol release, which can be estimated from the slope of the linear segment of absorbance vs. time profiles. The final concentration of DMSO was fixed and did not exceed 2.0%. The percentage of residual activity of PL was determined for each compound by comparing the lipase activity of PL with and without the compound. All assays were triplicated; thus inhibition percentages are the mean of triplicate observations. Orlistat, a known inhibitor of PL, was used as a positive control in the assay mixture
Results and discussion
Dietary fat plays the central role in obesity and therefore, to bring weight loss, the amount of fat available to be metabolized must be decreased. Inhibition of PL, the key enzyme for lipid absorption, is considered a valid therapeutic target for obesity. Phytochemicals identified from traditional medicinal plants present an exciting opportunity for the development of newer anti-obesity lipase inhibitor therapeutics. As a part of our screening project for biologically active anti-obesity agents from natural herbal resources, G. biloba has been investigated for its anti-lipase activity, since several studies have reported the hypolipidaemic activity of this plant [Citation23,Citation24]. Testing the anti-lipase activity of the methanolic extract of G. biloba leaves revealed a potent PL inhibition activity in a concentration-dependent manner with IC50 = 16.5 µg/mL. This pronounced inhibition has encouraged us to further explore the active constituent(s) that could be responsible for PL inhibition and thus could explain the hypolipidaemic activity of the plant. The methanolic extract of G. biloba leaves contains TTLs and flavonol glycosides [Citation28] as major constituents. The first major components, the flavonoids glycosides, like kaempferol, quercetin, apigenin, and luteolin [Citation28], are ubiquitous in nature with polar character. On the other hand, the TTLs, the second main bioactive constituents in G. biloba leaves extract, have chemical uniqueness as unsaponifiable lipids present as cyclic esters, which enriches their concentrations in the solvent (80% methanolic solution) selected for their extraction. On the other hand, flavonoids extraction is, generally, favoured with more polar solvents (20–50% alcoholic solutions) [Citation41].
Therefore, TTLs were considered as potential PL inhibitors for further exploration. TTLs include the diterpenes, ginkgolides A, B, C, J, K, and L (further abbreviated as G-A, G-B, G-C, G-J, G-K, and G-L), and the sesquiterpene bilobalide () [Citation28]. Our efforts commenced by evaluating the possibility of TTLs-PL binding via computer-aided molecular-modelling techniques. Accordingly, TTLs were docked into the binding pocket of PL (PDB code: 1LPB). Docking consists of two stages: (i) prediction of the conformation and pose of the bioactive compound into the binding cleft and (ii) estimation of the tightness of target-ligand interactions (scoring) [Citation42]. The final docked conformations are selected according to their scores. The docking study was conducted utilizing the docking engine FRED [Citation36]. FRED was recently reported to illustrate good overall performance, particularly in virtual high-throughput screening experiments. However, simulated molecular docking requires the user to provide FRED with an optimal set of parameters for the docking experiment (see the section “Docking experiment”). Therefore, to identify the optimal docking configuration and scoring function for PL, we extracted the ligand CllP () from crystallographic structure of PL (PDB code: 1LPB) [Citation37] and redocked it again into the same protein (self-docking) via a variety of docking conditions and scoring functions. Chemgauss2 was found to yield the closest model to the crystallographic structure as shown in . Subsequently, TTLs were docked into the binding pocket of PL employing the same optimal docking parameters and scoring function (see the section “Docking experiment”). The seven TTLs were predicted by simulated docking to bind within the active site of PL. shows the estimated binding affinity-rank for each compound calculated using Chemgauss2 scoring function. The preliminary docking study has supported our hypothesis that TTLs could be responsible for the PL inhibitory activity of the plant extract and encouraged us for further investigation to evaluate the inhibitory actions of TTLs. Accordingly, all the docked TTLs were ordered for in vitro activity bioassay. Unfortunately, only four TTLs were obtained: G-A, G-B, G-C, and bilobalide. The in vitro activity was expressed as the concentration of TTLs that inhibited PL activity by 50% (IC50) using PNPB as substrate. Unsurprisingly, three TTLs (G-A, G-B, and bilobalide) showed substantial inhibitory action against PL. As shown in , there is a clear successful ranking correlation between the Chemgauss2 scoring function and the IC50 values for the commercially available TTLs. This finding validates the successfulness of the docking conditions in predicting, approximately, the affinity and thus the bioactivity of the docked available compounds. Orlistat, a well-known potent PL inhibitor, was employed as positive inhibitor and it possessed an IC50 of 0.35 μg/mL.
Table 1. The docked G. biloba terpene trilactones (TTLs) with their IC50 values ranked according to the estimated binding affinity.
On the molecular level, several significant binding interactions can be observed between the docked natural compounds and the PL, as shown in . Comparison of the docked poses of the most potent compound () with the co-crystallized ligand within the binding site of PL () illustrates similarities in their binding profile. Both have potential hydrophobic interactions with the key amino acids Phe-215, Phe-77, and Tyr-114. Moreover, the most potent TTLs (G-A, IC50 = 22.9 µg/ mL) has a network of multiple strong hydrogen bonds with Ser-152, His-263, and Tyr-114, which stabilizes the ligand–protein complex and contributes to the relatively high affinity of G-A. Similarly, the co-crystallized ligand has analogous interactions with Ser-152 and His-263 (). However, it is strongly hydrogen bonded with two more amino acids: Leu-135 and Phe-77. In the structure of PL, His-263, Asp-176, and Ser-152 form a triad representing the lipolytic site. Furthermore, enzymatic activity has shown to be diminished after chemical modification of Ser-152, indicating its essential role for the catalytic activity [Citation13]. Therefore, it is unsurprising that compounds strongly bind to the catalytic triad, especially Ser-152 could inhibit the lipolytic activity. On the other hand, although bilobalide (IC50 = 60.1 µg/mL) has similar pattern of binding interactions (); however, it lacks the Ser-152 hydrogen bond attachment and the potential hydrophobic interactions provided by the tertiary butyl group. This might explain the lower affinity of bilobalide comparing with G-A. Finally, the inactive TTLs (G-C) lacks any hydrogen bonding interaction within the lipolytic site, especially with the catalytic triad, and the hydrophilic face projected outside the binding pockets towards water (). These simulated observations could explain its lack of activity.
Figure 3. (A) Detailed view of the co-crystallized structure (CllP) and the corresponding interacting amino acids within the binding site of pancreatic lipase (PL) (PDB code: 1LPB). (B) Detailed view of the docked ginkgolide A (G-A) structure and the corresponding interacting amino acid moieties within the binding site of PL. (C) Detailed view of the docked bilobalide–PL interactions. (D) Detailed view of the docked ginkgolide C–PL interactions.
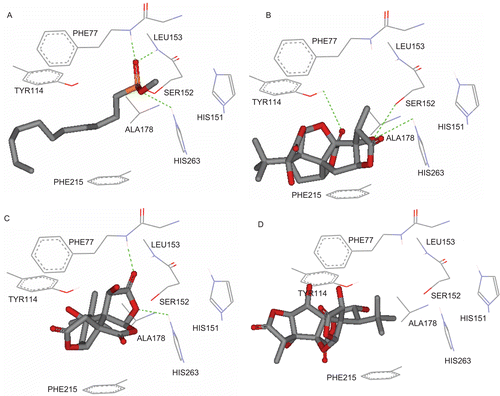
Conclusion
The results of this study clearly proved that G. biloba leaves extract has a substantial PL inhibition activity, which could partially explain its reported hypolipidaemic and fat mass reduction activities. Moreover, we have unequivocally proved through experimental testing and theoretical docking simulations, that the TTLs (ginkgolides A, B, and bilobalide) are PL inhibitors and therefore responsible, at least partially, for the G. biloba PL inhibitory activity. In conclusion, this work can be considered a new step towards the discovery of new natural safe hypolipidaemic PL inhibitors. The kinetic studies for the most active constituents will be carried out in the near future in attempt to correlate these studies with in vivo activities of the phenolic compounds.
Acknowledgements
This project was sponsored by the Deanship of Scientific Research at the University of Jordan, Grant No. (94/2009-2010). The authors wish to thank the Deanship of Scientific Research at the University of Jordan for their generous funds. The authors would like to thank also the OpenEye Scientific Software Corporation for providing free license of FRED software.
Declaration of interest
The authors report no conflicts of interest. The authors alone are responsible for the content and writing of the paper.
References
- Arbeeny, C.M. Addressing the unmet medical need for safe and effective weight loss therapies. Obes. Res. 2004, 12, 1191–1196.
- Harrold, J., Williams, G., Wong, S. Neuroendocrine targets for the treatment of obesity: physiological roles and unrealized opportunities. Curr Med Chem 2003, 3, 141–155.
- Wang, Y., Beydoun, M.A., Liang, L., Caballero, B., Kumanyika, S.K. Will all Americans become overweight or obese? Estimating the progression and cost of the US obesity epidemic. Obesity (Silver Spring) 2008, 16, 2323–2330.
- Wang, Y., Beydoun, M.A. The obesity epidemic in the United States—gender, age, socioeconomic, racial/ethnic, and geographic characteristics: a systematic review and meta-regression analysis. Epidemiol. Rev. 2007, 29, 6–28.
- Hirani, V. Anthropometric measures, overweight and obesity. In: Sproston, K., Primatesta, P. (eds). Health Survey for England 2003. Volume 2: Risk Factors for Cardiovascular Disease. Joint Health Surveys Unit: London, 2004, pp. 143–179.
- Cameron, A.J., Welborn, T.A., Zimmet, P.Z., Dunstan, D.W., Owen, N., Salmon, J., Dalton, M., Jolley, D., Shaw, J.E. Overweight and obesity in Australia: the 1999–2000 Australian Diabetes, Obesity and Lifestyle Study (AusDiab). Med. J. Aust.s 2003, 178, 427–432.
- Bogers, R.P., Bemelmans, W.J., Hoogenveen, R.T., Boshuizen, H.C., Woodward, M., Knekt, P., van Dam, R.M., Hu, F.B., Visscher, T.L., Menotti, A., Thorpe, R.J., Jr., Jamrozik, K., Calling, S., Strand, B.H., Shipley, M.J.; for the BMI-CHD Collaboration Investigators. Association of overweight with increased risk of coronary heart disease partly independent of blood pressure and cholesterol levels: a meta-analysis of 21 cohort studies including more than 300 000 persons. Arch. Intern. Med. 2007, 167, 1720–1728.
- National task force on the prevention and treatment of obesity. Overweight, obesity, and health risk. Arch. Intern. Med. 2000, 160, 898–904.
- Hyde, R. Europe battles with obesity. Lancet 2008, 371, 2160–2161.
- National Heart Lung and Blood Institute in cooperation with The National Institute of Diabetes and Digestive and Kidney Diseases. Clinical Guidelines on the Identification, Evaluation, and Treatment of Overweight and Obesity in Adults. The evidence report, 1998.
- Ballinger, A., Peikin, S.R. Orlistat: its current status as an anti-obesity drug. Eur. J. Pharmacol. 2002, 440, 109–117.
- Yanovski, S.Z., Yanovski, J.A. Obesity. N. Engl. J. Med. 2002, 346, 591–602.
- Winkler, F.K., D’Arcy, A., Hunziker, W. Structure of human pancreatic lipase. Nature 1990, 343, 771–774.
- Tilbeurgh, H.V. Structure of the pancreatic lipase–procolipase complex. Nature 1992, 359, 159–162.
- Gargouri, Y., Ransac, S., Verger, R. Covalent inhibition of digestive lipases: an in vitro study. Biochim. Biophys. Acta 1997, 1344, 6–37.
- Hadváry, P., Lengsfeld, H., Wolfer, H. Inhibition of pancreatic lipase in vitro by the covalent inhibitor tetrahydrolipstatin. Biochem. J. 1988, 256, 357–361.
- Drent, M.L., van der Veen, E.A. Lipase inhibition: a novel concept in the treatment of obesity. Int. J. Obes. Relat. Metab. Disord. 1993, 17, 241–244.
- Bustanji, Y., Mohammad, M., Hudaib, M., Tawaha, K., Al-Masri, I., AlKhatib, H., Issa, A., Alali, F. Screening of some medicinal plants for their pancreatic lipase inhibitory potential. Jordan J. Pharm. Sci. 2010, Jordan J. Pharm. Sci. In Press.
- Morikawa, T., Xie, Y., Asao, Y., Okamoto, M., Yamashita, C., Muraoka, O., Matsuda, H., Pongpiriyadacha, Y., Yuan, D., Yoshikawa, M. Oleanane-type triterpene oligoglycosides with pancreatic lipase inhibitory activity from the pericarps of Sapindus rarak. Phytochemistry 2009, 70, 1166–1172.
- Nakai, M., Fukui, Y., Asami, S., Toyoda-Ono, Y., Iwashita, T., Shibata, H., Mitsunaga, T., Hashimoto, F., Kiso, Y. Inhibitory effects of oolong tea polyphenols on pancreatic lipase in vitro. J. Agric. Food Chem. 2005, 53, 4593–4598.
- Tian, W.X., Li, L.C., Wu, X.D., Chen, C.C. Weight reduction by Chinese medicinal herbs may be related to inhibition of fatty acid synthase. Life Sci. 2004, 74, 2389–2399.
- Cheng, J., Ni, H., Guo, W., Yu, L., Shen, Q., Lin, Y. Chinese medicinal composition effective in reducing blood glucose and blood lipid, and its preparation method and uses. Faming Zhuanli Shenqing Gongkai Shuomingshu 2007, CODEN: CNXXEV CN 101085121 A 20071212. Application: CN 2007-10024578 20070622.
- Dubey, A.K., Devi, A., Kutty, G., Shankar, R.P. Hypolipidemic activity of Ginkgo biloba extract, EGB 761 in hypercholesterolemic wistar rats. Iran J. Pharmacol. Ther. 2005, 4, 9–12.
- Christen, Y. Use of Ginkgo biloba extract for increasing muscle mass and reducing fat mass. Faming Zhuanli Shenqing Gongkai Shuomingshu 2005, CODEN: CNXXEV CN 1668321 A 20050914. Application: CN 2003-816570 20030715.
- Duan, Z., Guo, S., He, D., Yan, X. Traditional Chinese medicine extract capable of inhibiting lipase activity. Faming Zhuanli Shenqing Gongkai Shuomingshu 2009, 24 pp. CODEN: CNXXEV CN 101361886 A 20090211 Application: CN 2007-10120014 20070807.
- Mahady, G.B. Ginkgo biloba for the prevention and treatment of cardiovascular disease: a review of the literature. J. Cardiovasc. Nurs. 2002, 16, 21–32.
- Yao, P., Song, F., Li, K., Zhou, S., Liu, S., Sun, X., Nussler, A.K., Liu, L. Ginkgo biloba extract prevents ethanol induced dyslipidemia. Am. J. Chin. Med. 2007, 35, 643–652.
- Singh, B., Kaur, P., Gopichand, Singh, R.D., Ahuja, P.S. Biology and chemistry of Ginkgo biloba. Fitoterapia 2008, 79, 401–418.
- Chen, J.W., Chen, Y.H., Lin, F.Y., Chen, Y.L., Lin, S.J. Ginkgo biloba extract inhibits tumor necrosis factor-alpha-induced reactive oxygen species generation, transcription factor activation, and cell adhesion molecule expression in human aortic endothelial cells. Arterioscler. Thromb. Vasc. Biol. 2003, 23, 1559–1566.
- Kudolo, G.B., Wang, W., Barrientos, J., Elrod, R., Blodgett, J. The ingestion of Ginkgo biloba extract (EGb 761) inhibits arachidonic acid-mediated platelet aggregation and thromboxane B2 production in healthy volunteers. J. Herb. Pharmacother. 2004, 4, 13–26.
- Koltermann, A., Hartkorn, A., Koch, E., Fürst, R., Vollmar, A.M., Zahler, S. Ginkgo biloba extract EGb 761 increases endothelial nitric oxide production in vitro and in vivo. Cell. Mol. Life Sci. 2007, 64, 1715–1722.
- Yao, Z.X., Han, Z., Drieu, K., Papadopoulos, V. Ginkgo biloba extract (Egb 761) inhibits beta-amyloid production by lowering free cholesterol levels. J. Nutr. Biochem. 2004, 15, 749–756.
- Maitra, I., Marcocci, L., Droy-Lefaix, M.T., Packer, L. Peroxyl radical scavenging activity of Ginkgo biloba extract EGb 761. Biochem. Pharmacol. 1995, 49, 1649–1655.
- Cho, H.J., Nam, K.S. Inhibitory effect of ginkgolide B on platelet aggregation in a cAMP- and cGMP-dependent manner by activated MMP-9. J. Biochem. Mol. Biol. 2007, 40, 678–683.
- OMEGA (version 2.3.2) 2008. OpenEye Scientific Software Inc., Santa Fe, New Mexico, USA.
- FRED (version 2.2.5) 2009. OpenEye Scientific Software, (www.eyesopen.com), USA.
- Egloff, M.P., Marguet, F., Buono, G., Verger, R., Cambillau, C., van Tilbeurgh, H. The 2.46 A resolution structure of the pancreatic lipase-colipase complex inhibited by a C11 alkyl phosphonate. Biochemistry 1995, 34, 2751–2762.
- Lee, Y.P., Chung, G.H., Rhee, J.S. Purification and characterization of Pseudomonas fluorescens SIK W1 lipase expressed in Escherichia coli. Biochim. Biophys. Acta, Lipids Lipid Metab. 1993, 1169, 156–164.
- Sharma, N., Sharma, V.K., Seo, S.Y. Screening of some medicinal plants for anti-lipase activity. J. Ethnopharmacol. 2005, 97, 453–456.
- Mohammad, M., Aiedeh, K.M., Alkhatib, H.S., Tawaha, K., Alkhalidi, B., Al-Masri, I.M., Hamed, S., Bustanji, Y.A. Comparative enzymatic inhibition assay as a surrogate indicator of pharmaceutical and potency equivalence of two orlistat formulations. Jordan J. Pharm. Sci. 2010, 3, 69–75.
- Ding, Z. Studies on extraction and isolation of flavonoids from ginkgo leaves. J. Food Quality 1999, 22, 693–700.
- Bustanji, Y., Taha, M.O., Almasri, I.M., Al-Ghussein, M.A., Mohammad, M.K., Alkhatib, H.S. Inhibition of glycogen synthase kinase by curcumin: investigation by simulated molecular docking and subsequent in vitro/in vivo evaluation. J. Enzyme Inhib. Med. Chem. 2009, 24, 771–778.