Abstract
A novel series of N,N′-bis-methylenedioxybenzyl-alkylenediamines 5a–5g have been designed, synthesized and evaluated as bivalent anti-Alzheimer’s disease ligands. The enzyme inhibition assay results indicated that compounds 5e–5g inhibit both acetylcholinesterase (AChE) and butyrylcholinesterase (BuChE) in the micromolar range (IC50, 2.76–4.24 µM for AChE and 3.02–5.14 µM for BuChE), which was in the same potential as the reference compound rivastigmine (IC50, 5.50 µM for AChE and 1.60 µM for BuChE). It was found that compounds could bind simultaneously to the peripheral and catalytic sites of AChE. β-Amyloid (Aβ) aggregation inhibition assay results showed that compound 5e exhibited highest self-mediated Aβ fibril aggregation inhibition activity (40.3%) with a similar potential as curcumin (41.6%). It was also found that 5e–5g did not affect neuroblastoma cell viability at the concentration of 50 μM.
Introduction
Alzheimer’s disease (AD) is a progressive neurodegenerative brain disorder that affects millions among the aging population. The etiology of AD is not completely known, although there are diverse hallmarks such as low levels of acetylcholine and β-amyloid (Aβ) deposits that seem to play significant roles in the diseaseCitation1. One of the most useful approaches involved in the design of new agents for the treatment of AD focuses on increasing cholinergic neurotransmission in the brain by inhibiting cholinesterases (ChE) such as donepezil, rivastigmine and galanthamineCitation2. The main function of both acetylcholinesterase (AChE) and butyrylcholinesterase (BuChE) is terminating the impulse transmission at cholinergic synapses in which ChE catalyzes the hydrolysis of the neurotransmitter acetylcholine into choline and acetic acid. But clinical experience shows that ChE inhibition is a palliative treatment, which does not address the etiology of ADCitation3. In recent times, due to the multi-pathogenesis of AD, one of the current strategies to develop novel anti-AD agents focuses on compounds with multiple potenciesCitation4.
On the other hand, the progressive deposition of Aβ in AD’s brain is generally considered to be fundamental to the development of neurodegenerative pathology. And cell toxicity associated with Aβ fibril aggregation provides an explanation for the neuronal cell loss found in ADCitation5. Therefore, preventing of Aβ fibril aggregation in the brain is currently another target as potential therapy for ADCitation6,Citation7. Several inhibitors, such as curcuminCitation8,Citation9 and benzofuran analoguesCitation5,Citation10, were developed.
Schizadrins were main bioactive components in medicinal plants of schisandra that have exhibited many pharmacological effectsCitation11. Recently, it was reported that the schizadrins showed significant inhibition of the activity of AChE, and structure–activity relationship indicated that aromatic methylenedioxy group was necessaryCitation12. Moreover, it is interesting that γ-schizadrin containing methylenedioxybenzyl had inhibition activity on Aβ productionCitation13. In order to develop a novel type of difunctional anti-AD agents that could inhibit both cholinesterase and Aβ aggregation, a series of N,N′-bis-methylenedioxybenzyl-alkylenediamines (NBMA, 5a–5g) were designed, synthesized, and assayed for their AChE, BuChE, and Aβ aggregation inhibitory activities.
Materials and methods
Materials
1H and 13C NMR spectra were recorded using TMS (Tetramethyl silicane) as the internal standard in CDCl3 with a Bruker BioSpin GmbH spectrometer at 400 and 100 MHz, respectively; MS spectra were recorded on a Shimadzu LCMS-2010A instrument with an ESI mass selective detector, and high-resolution mass spectra (HRMS) on Shimadzu LCMS-IT-TOF (Liquid chromatography-mass spectrometry-ion trap-time of flight). The purities of compounds were confirmed to be higher than 95% by analytical HPLC performed with a dual pump Shimadzu LC-20AB system equipped with a Ultimate XB-C18 column (4.6 × 250 mm, 5 µm) and eluted with methanol/water (30:70 to 50:50) containing 0.1% TFA (Trifluoroacetic Acid) at a flow rate of 0.5 mL/min. All reagents used in this study were of AR grade and available commercially.
Chemistry
The synthetic routes to target compounds are summarized in . The starting material vanillin 1 was treated with bromine in acetic acid to give 5-bromovanillin 2, which was heated with NaOH and copper powder in water yielded 3,4-dihydroxy-5- methoxybenzaldehyde 3. Methylenation of compound 3 by a convenient procedure (CH2Br2–CuO–K2CO3–DMF) afforded the intermediate 4 in 72% yield, and conversion to the target compounds 5a–5g by reaction with diamine and then reduction with NaBH4 in methanol. All the target compounds were purified by flash chromatography with chloroform/methanol/ammonia (20:1:0.5%) elution.
Scheme 1. Reagent and condition: (i) Br2, AcOH, rt, 1h; 95% (ii) NaOH, Cu, H2O, reflux, 18h; 60% (iii) CH2Br2,CuO, K2CO3, DMF, 140°C, 4h; 70% (iv) MeOH, diamine, rt, 4h (v) NaBH4, rt, 4h, 80–90%.
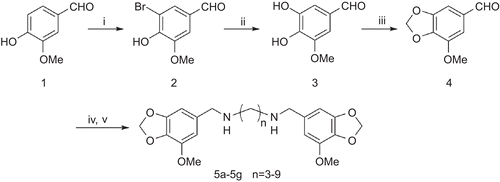
There are some different methods in the preparation of intermediate 4 reported in many articlesCitation14–16. Most of them reacts under nitrogen, and the yield was between 50 and 70%. In our synthesis, CuO was used as catalyst, K2CO3, CH2Br2, and 3 were stirred in DMF (Dimethylformamide) at 140°C for 3 h without nitrogen, could get intermediate 4 in a satisfactory yield.
Synthesis of intermediate 4
CuO (0.13 g), anhydrous K2CO3 (1.75 g) and CH2Br2 (1.25 g) were added to a solution of compound 3 (1.0g) in anhydrous DMF (12.5 mL), and the mixture was heated at 140°C for 4 h. The reaction mixture was poured into water, and the product was extracted with ether. The ethereal extract was washed successively with 2% HCl, 2% NaOH and water. Evaporation of the solvent after drying over anhydrous K2CO3 gave light brown crystalline mass, which on recrystallization from MeOH, afforded colorless pillarsCitation14,Citation17, yield: 72%, m.p. 129–130°C (m.p. 131–132°C)Citation15.
General procedure for the synthesis of compounds 5a–5g
Aromatic aldehyde 4 (0.36 g, 2 mmol) and 1 mmol diamine were stirred in MeOH for 4 h and then were reduced directly by NaBH4 (6 mmol) at room temperature for 4 h. The solvent was evaporated and the residue was poured into water and extracted with ethyl acetate, the solution was dried over anhydrous MgSO4 and then concentrated, the target compounds were purified by flash chromatography with chloroform/methanol/ammonia (20:1:0.5%) elutionCitation18.
N1,N3-bis((7-methoxybenzo[d][1,3]dioxol-5-yl)methyl)propane-1,3-diamine (5a)
Colorless oil. MS (ESI, m/z): [M + H]+ 403.1; 1H NMR (400 MHz, CDCl3) δ 6.50 (s, 4H, Ar–H), 5.94 (s, 4H,−OCH2O–), 3.88 (s, 6H,−OCH3), 3.68 (s, 4H,−CH2–), 2.70 (t, J = 6.7 Hz, 4H,−CH2–), 2.40 (s, 2H,−CH2–), 1.77–1.70 (m, 2H); 13C NMR (100 MHz, CDCl3) δ 148.83, 143.53, 134.55, 134.14, 107.47, 102.20, 101.32, 56.54, 53.89, 47.79, 29.54; purity: 97.3% by HPLC. HRMS (ESI): calcd for (M + H)+ (C21H26N2O6) requires m/z 403.1869, found 403.1856.
N1,N4-bis((7-methoxybenzo[d][1,3]dioxol-5-yl)methyl)butane-1,4-diamine (5b)
Colorless oil. MS (ESI, m/z): [M+H]+ 417.1; 1H NMR (400 MHz, CDCl3) δ 6.50 (s, 4H, Ar–H), 5.93 (s, 4H,−OCH2O–), 3.89 (s, 6H,−OCH3), 3.67 (s, 4H,−CH2–), 2.62 (t, J = 6.5 Hz, 4H,−CH2–), 1.82 (s, 2H,−CH2–), 1.71–1.39 (m, 2H,−CH2–); 13C NMR (100 MHz, CDCl3) δ 148.79, 143.51, 135.02, 134.05, 107.36, 102.18, 101.29, 56.54, 53.96, 49.07, 27.81; purity: 99.9% by HPLC. HRMS (ESI): calcd for (M + H)+ (C22H28N2O6) requires m/z 417.2026, found 417.2017.
N1,N5-bis((7-methoxybenzo[d][1,3]dioxol-5-yl)methyl)pentane-1,5-diamine (5c)
Colorless oil. MS (ESI, m/z): [M + H]+ 431.2; 1H NMR (400 MHz, CDCl3) δ 6.50 (s, 4H, Ar–H), 5.93 (s, 4H,−OCH2O–), 3.89 (s, 6H,−OCH3), 3.67 (s, 4H,−CH2–), 2.60 (t, J = 7.1 Hz, 4H,−CH2–), 1.57–1.46 (m, 4H,−CH2–), 1.45–1.32 (m, 4H,−CH2–); 13C NMR (100 MHz, CDCl3) δ 148.79, 143.51, 135.27, 134.02, 107.34, 102.18, 101.28, 56.55, 54.11, 49.24, 30.00, 25.11; purity: 99.1% by HPLC. HRMS (ESI): calcd for (M + H)+ (C23H30N2O6) requires m/z 431.2182, found 431.2178.
N1,N6-bis((7-methoxybenzo[d][1,3]dioxol-5-yl)methyl)hexane-1,6-diamine(5d)
Colorless oil. MS (ESI, m/z): [M + H]+ 445.1; 1H NMR (400 MHz, CDCl3) δ 6.51 (s, 4H Ar–H), 5.93 (s, 4H,−OCH2O–), 3.89 (s, 6H,−OCH3), 3.67 (s, 4H,−CH2–), 2.60 (t, J = 7.2 Hz, 4H,−CH2–), 1.54–1.45 (m, 6H,−CH2–), 1.37–1.30 (m, 4H,−CH2–); 13C NMR (100 MHz, CDCl3) δ 148.81, 143.53, 135.29, 134.04, 107.39, 102.19, 101.27, 56.57, 54.09, 49.28, 30.05, 27.30; purity: 100% by HPLC. HRMS (ESI): calcd for (M + H)+ (C24H32N2O6) requires m/z 445.2339, found 445.2340.
N1,N7-bis((7-methoxybenzo[d][1,3]dioxol-5-yl)methyl)heptane-1,7-diamine (5e)
Colorless oil. MS (ESI, m/z): [M + H]+ 459.0; 1H NMR (400 MHz, CDCl3) δ 6.51 (s, 4H, Ar–H), 5.94 (s, 4H,−OCH2O–), 3.90 (s, 6H,−OCH3), 3.68 (s, 4H,−CH2–), 2.59 (t, J = 7.2 Hz, 4H,−CH2–), 1.54–1.46 (m, 6H,−CH2–), 1.37–1.30 (m, 6H,−CH2–); 13C NMR (100 MHz, CDCl3) δ 148.79, 143.52, 135.27, 134.03, 107.34, 102.20, 101.28, 56.56, 54.10, 49.33, 30.03, 29.46, 27.30; purity: 99.8% by HPLC. HRMS (ESI): calcd for (M + H)+ (C25H34N2O6) requires m/z 459.2495, found 459.2493.
N1, N8-bis((7-methoxybenzo[d][1,3]dioxol-5-yl)methyl)octane-1,8-diamine (5f)
Colorless oil. MS (ESI, m/z): [M + H]+ 473.1; 1H NMR (400 MHz, CDCl3) δ 6.51 (s, 4H, Ar–H), 5.93 (s, 4H,−OCH2O–), 3.89 (s, 6H,−OCH3), 3.67 (s, 4H,−CH2–), 2.59 (t, J = 7.2 Hz, 4H,−CH2–), 1.61 (s, 2H,−CH2–), 1.54–1.44 (m, 4H,−CH2–), 1.33–1.25 (m, 8H,−CH2–); 13C NMR (100 MHz, CDCl3) δ 148.77, 143.49, 135.24, 133.99, 107.32, 102.18, 101.26, 56.55, 56.48, 54.07, 49.32, 30.04, 29.47, 27.28; purity: 99.9% by HPLC. HRMS (ESI): calcd for (M + H)+ (C26H36N2O6) requires m/z 473.2652, found 473.2652.
N1,N9-bis((7-methoxybenzo[d][1,3]dioxol-5-yl)methyl)nonane-1,9-diamine (5g)
Colorless oil. MS (ESI, m/z): [M + H]+ 487.2; 1H NMR (400 MHz, CDCl3) δ 6.51 (s, 4H, Ar–H), 5.93 (s, 4H,−OCH2O–), 3.89 (s, 6H,−OCH3), 3.68 (s, 4H,−CH2–), 2.59 (t, J = 7.2 Hz, 4H,−CH2–), 1.67 (s, 2H,−CH2–), 1.56–1.40 (m, 4H,−CH2–), 1.32–1.25 (s, 10H,−CH2–); 13C NMR (100 MHz, CDCl3) δ 148.79, 143.51, 135.24, 134.02, 107.36, 102.21, 101.28, 56.55, 54.08, 49.34, 30.05, 29.52, 29.49, 27.34; purity: 99.7% by HPLC. HRMS (ESI): calcd for (M + H)+ (C27H38N2O6) requires m/z 487.2808, found 487.2807.
Pharmacology
All the synthesized compounds were screened for AChE and BuChE inhibition activities. AChE (E.C. 3.1.1.7, from electric eel), BuChE (E.C. 3.1.1.8, from equine serum), 5,5′-dithiobis-(2-nitrobenzoic acid) (Ellman’s reagent, DTNB), butyrylthiocholine chloride and acetylthiocholine chloride (ATC) were purchased from Sigma–Aldrich and rivastigmine hydrochloride standard was purchased from Sunve (Shanghai) Pharmaceutical Co., Ltd. Curcumin used in this work were synthesized and characterized in our laboratoryCitation19.
Enzyme inhibition assays
All the assays were under 0.1 M KH2PO4/K2HPO4 buffer, pH 8.0, using a Shimadzu 2450 Spectrophotometer. Enzyme solutions were prepared to give 2.0 units/mL in 2 mL aliquots. The assay medium contained phosphate buffer, pH 8.0 (1 mL), 50 μL of 0.01 M DTNB, 10 μL of enzyme, and 50 μL of 0.01 M substrate (ATC). The substrate was added to the assay medium containing enzyme, buffer, and DTNB with inhibitor after 15 min of incubation time. The activity was determined by measuring the increase in absorbance at 412 nm at 1 min intervals at 37°C. Calculations were performed according to the method of the equation in Ellman et al.Citation20. In vitro BuChE assay use the similar method described above.
Kinetic characterization of AChE was performed using a reported method. Six different concentrations of substrate were mixed in the 1 mL 0.1 M KH2PO4/K2HPO4 buffer (pH 8.0), containing 50 μL of DTNB, 10 μL AChE, and 50 μL substrate. Test compound was added into the assay solution and pre-incubated with the enzyme at 37°C for 15 min, followed by the addition of substrate. Kinetic characterization of the hydrolysis of ATC catalyzed by AChE was done spectrometrically at 412 nm. A parallel control with no inhibitor in the mixture, allowed adjusting activities to be measured at various times.
Inhibition of self-mediated Aβ aggregation
The thioflavin-T fluorescence method was usedCitation21. Aβ42 peptide sodium (Anaspec Inc.) was dissolved in phosphate buffer (pH 7.40, 0.01 M) to obtain a 20 μM solution. Compounds were first prepared in dimethyl sulfoxide (DMSO) to obtain a 10 mM solution. The final concentration of Aβ42 and inhibitors was 20 μM. After incubated in 37°C for 24 h, thioflavin-T (5 μM in 50 mM glycine–NaOH buffer, pH 8.00) was added. Fluorescence intensity method was carried out (excitation at 450 nm and emission at 485 nm). Each inhibitor was run in triplicate. The fluorescence intensities were recorded, and the percentage of inhibition on aggregation was calculated by the following expression: (1 − IFi/IFc) × 100% in which IFi and IFc were the fluorescence intensities obtained for absorbance in the presence and absence of inhibitors, respectively, after subtracting the fluorescence of respective blanks.
MTT (methyl thiazolyl tetrazolium) assay of SH-SY5Y cell viability
SH-SY5Y cells, at passages between 3 and 16 after thawing, were maintained in Dulbecco’s modified Eagle’s medium containing 15 nonessential amino acids and supplemented with 10% fetal calf serum, 1 mM glutamine, 50 mg/μL penicillin, and 50 mg/μL streptomycin. Cultures were seeded into flasks containing supplemented medium and were maintained at 37°C in 5% CO2 humidified air. For assays, SH-SY5Y cells were sub-cultured in 96-well plates at a seeding density of 10,000 cells per well. After 24 h, they were placed into serum-free medium and treated with compounds. After 48 h, the survival of cells was determined by MTT assay. Briefly, after incubated with 20 μL of MTT (2.5 mg/mL) at 37°C for 4 h, living cells containing MTT formazon crystals were solubilized in 100 μL of DMSO. The absorbance of each well was measured by a microculture plate reader at the wavelength of 570 nm.
Results and discussion
Enzyme inhibition assays
All the newly synthesized compounds (5a–5g) were tested for their inhibition activities toward AChE and BuChE in vitro according to the modified Ellman method with commercially available rivastigmine as the reference standard. The ChE inhibition results were summarized in .
Table 1. Inhibition activity of AChE and BuChE.
The results showed that most of the target compounds possessed ChE inhibition activity, and the AChE inhibitory potency of derivatives was closely related to the length of the alkylene chain. Compared with rivastigmine (IC50 = 5.50), 5e, 5f, and 5g (IC50 = 4.24, 2.79, and 2.76 µM, respectively) showed slightly better AChE inhibitory activity, 5c and 5d (IC50 = 13.25 and 9.78 µM) showed lower inhibition potential, 5b showed a quite weak inhibitory effect with a IC50 value of 119.96 µM, and 5a was not active at 125µM. The results indicated that NBMA derivatives required chains of suitable length to bind at both the catalytic site and the peripheral site of the AChE.
Compared with AChE activity, the BuChE inhibitory potency was less impacted by the chain length. The majority of compounds (5c–5f) showed a similar inhibition activity with rivastigmine (IC50 = 1.60 µM), the highest potency (IC50 = 2.07 µM) was achieved in 5d with six methylene groups between two aromatic units.
Most NBMA derivatives showed greater selectivity for BuChE. The reason seems to be the enzymic conformational difference that relatively small residues line the gorge in BuChE, this allow bulky group to better fit inside the gorge of BuChECitation2. And recently, BuChE was considered a potential target, because it also plays an important role in regulating acetylcholine levelsCitation22. The concurrent inhibition of both AChE and BuChE was shown to provide additional benefits in AD, such as rivastigmineCitation23. In this study 5e–5g had significant inhibition at similar lever with rivastigmine on both enzymes.
The nature of AChE inhibition caused by a representative compound 5g was investigated by the graphical analysis of steady-state inhibition data (). The Lineweaver–Burk plots showed both increasing slopes and increasing intercepts with higher inhibitor concentration. The pattern indicated the mixed-type inhibition that was similar to that of tacrine. The result revealed that compound 5g was able to bind both of the catalytic site and the peripheral site of AChE.
Inhibition of self-mediated Aβ aggregation
All the target compounds were tested for their ability to inhibit self-mediated aggregation of Aβ(1-42) by using a thioflavin T fluorescence methodCitation21. Compared with the reference compound curcumin, the results showed that all the target compounds significantly inhibit Aβ aggregation at 20μM, with percentages of inhibition ranging from 5 to 40% (). The compound 5e was found to be most potent. And the activity of the compounds did not depend on the chain length of the connecting linker.
MTT assay of cell viability
The toxicity of synthesized compounds was determined in human neuroblastoma cell line SH-SY5Y. Results indicated that the most potent three inhibitors, 5e, 5d, and 5g, showed no obvious effect on cell viability at concentrations of 50 μM, as shown in . Compared with curcumin, they had a lower toxicity on cell viability.
Table 2. MTT assay of SH-SY5Y cell viability.
Conclusions
In summary, seven of NBMA compounds 5a–5g were designed, synthesized and evaluated for cholinesterase, self-induced Aβ aggregation inhibition activities and human neuroblastoma cell viability. Results indicated that their AChE and BuChE inhibition activities were comparable with or stronger than that of rivastigmine. The inhibition activity of AChE was greatly influenced by the length of the alkylene chain. Besides, compounds 5e, 5f, and 5g potently significantly inhibited self-induced Aβ aggregation. And compounds 5e–5g did not show significant cell cytotoxicity for neuroblastoma cell at concentrations of 50 μM. Compound 5e may be considered to be novel multipotent anti-Alzheimer lead drugs and further pharmacological evaluations are in progress.
Declaration of interest
The authors thank the Natural Science Foundation of China (Grants U0832005, 90813011), and the Science Foundation of Guangzhou (2009A1-E011-6) for financial support of this study.
References
- Fernández-Bachiller MI, Pérez C, Campillo NE, Páez JA, González-Muñoz GC, Usán P et al. Tacrine-melatonin hybrids as multifunctional agents for Alzheimer’s disease, with cholinergic, antioxidant, and neuroprotective properties. Chemmedchem 2009;4:828–841.
- Rizzo S, Rivière C, Piazzi L, Bisi A, Gobbi S, Bartolini M et al. Benzofuran-based hybrid compounds for the inhibition of cholinesterase activity, beta amyloid aggregation, and abeta neurotoxicity. J Med Chem 2008;51:2883–2886.
- Tumiatti V, Milelli A, Minarini A, Rosini M, Bolognesi ML, Micco M et al. Structure–activity relationships of acetylcholinesterase noncovalent inhibitors based on a polyamine backbone. 4. Further investigation on the inner spacer. J Med Chem 2008;51:7308–7312.
- Cavalli A, Bolognesi ML, Minarini A, Rosini M, Tumiatti V, Recanatini M et al. Multi-target-directed ligands to combat neurodegenerative diseases. J Med Chem 2008;51:347–372.
- Howlett DR, Perry AE, Godfrey F, Swatton JE, Jennings KH, Spitzfaden C et al. Inhibition of fibril formation in beta-amyloid peptide by a novel series of benzofurans. Biochem J 1999;340 (Pt 1):283–289.
- Thorsett ED, Latimer LH. Therapeutic approaches to Alzheimer’s disease. Curr Opin Chem Biol 2000;4:377–382.
- Wolfe MS, Citron M, Diehl TS, Xia W, Donkor IO, Selkoe DJ. A substrate-based difluoro ketone selectively inhibits Alzheimer’s gamma-secretase activity. J Med Chem 1998;41:6–9.
- Yang F, Lim GP, Begum AN, Ubeda OJ, Simmons MR, Ambegaokar SS et al. Curcumin inhibits formation of amyloid beta oligomers and fibrils, binds plaques, and reduces amyloid in vivo. J Biol Chem 2005;280:5892–5901.
- Lim GP, Chu T, Yang F, Beech W, Frautschy SA, Cole GM. The curry spice curcumin reduces oxidative damage and amyloid pathology in an Alzheimer transgenic mouse. J Neurosci 2001;21:8370–8377.
- Ono M, Kung MP, Hou C, Kung HF. Benzofuran derivatives as Abeta-aggregate-specific imaging agents for Alzheimer’s disease. Nucl Med Biol 2002;29:633–642.
- Kuo YH, Wu MD, Hung CC, Huang RL, Yang Kuo LM, Shen YC et al. Syntheses of C(18) dibenzocyclooctadiene lignan derivatives as anti-HBsAg and anti-HBeAg agents. Bioorg Med Chem 2005;13:1555–1561.
- Hung TM, Na M, Min BS, Ngoc TM, Lee I, Zhang X et al. Acetylcholinesterase inhibitory effect of lignans isolated from Schizandra chinensis. Arch Pharm Res 2007;30:685–690.
- Liu W, Yu R, Wu JH, Luo HM. [Gamma-Schisandrin inhibits production of amyloid beta-protein 42 in M146L cells]. Yao Xue Xue Bao 2006;41:1136–1140.
- Tomita M, Aoyagi Y. Cupric oxide as an efficient catalyst for the methylenation of catechols. Chem Pharm Bull 1968;16:523–526.
- Schneiders GE, Stevenson R. Structure and synthesis of (±)-Wuweizisu C. J Org Chem 1981;46:2969–2971.
- Mckittrick B. A., Stevenson R. Synthesis of the yeast antioxidant benzofuran and analogues. J Chem Soc Perkin Trans 1984;4:709–712.
- James EE, Steven RL. A convenient synthesis of 3,4-dimethoxy-5-hydroxy- benzaldehyde. Synth Commun 1998;28:1517–1524.
- Zhou JJ, Huang H, Xie SQ, Wang YX, Zhao J, Wang CJ. The synthesis and molecular recognization of the polyamine transporter of hydrazine-modified diamine conjugates. Chin Chem Lett 2008;19:99–101.
- Qiu X, Liu Z, Shao WY, Liu X, Jing DP, Yu YJ et al. Synthesis and evaluation of curcumin analogues as potential thioredoxin reductase inhibitors. Bioorg Med Chem 2008;16:8035–8041.
- Ellman GL, Courtney KD, Andres V Jr, Feather-Stone RM. A new and rapid colorimetric determination of acetylcholinesterase activity. Biochem Pharmacol 1961;7:88–95.
- Rosini M, Simoni E, Bartolini M, Cavalli A, Ceccarini L, Pascu N et al. Inhibition of acetylcholinesterase, beta-amyloid aggregation, and NMDA receptors in Alzheimer’s disease: a promising direction for the multi-target-directed ligands gold rush. J Med Chem 2008;51:4381–4384.
- Elsinghorst PW, Tanarro CM, Gütschow M. Novel heterobivalent tacrine derivatives as cholinesterase inhibitors with notable selectivity toward butyrylcholinesterase. J Med Chem 2006;49:7540–7544.
- Pan L, Tan JH, Hou JQ, Huang SL, Gu LQ, Huang ZS. Design, synthesis and evaluation of isaindigotone derivatives as acetylcholinesterase and butyrylcholinesterase inhibitors. Bioorg Med Chem Lett 2008;18:3790–3793.