Abstract
In the present study, some acetamide derivatives were synthesized and their potential analgesic activities were investigated. N-(benzothiazol-2-yl)-2-[(1-substituted-1H-tetrazol-5-yl)thio]acetamide derivatives were obtained by the nucleophilic substitution reaction of 2-chloro-N-(benzothiazole-2-yl)acetamides with appropriate tetrazol-5-thioles. The chemical structures of the compounds were elucidated by IR, 1H-NMR, 13C-NMR and FAB+-MS spectral data and elemental analyses. The prepared compounds were investigated for their potential analgesic properties against thermal, mechanical and chemical nociceptive stimuli using hot-plate, tail-clip and acetic acid-induced writhing tests, respectively. The assessment of motor coordination was carried out using Rota-Rod test. Tested compounds applied at 100 mg/kg doses caused significant decrease in acetic acid-induced writhing responses and increase in hot-plate and tail-clip latencies. None of the compounds exhibited destructive effect on motor coordination of the mice in Rota-Rod performance.
Introduction
Pain, which is associated with a number of different conditions, is the common symptom of many diseases. Although there are many drugs currently available for relieving pain, the treatment of pain is still a major problem due to the adverse effects accompanying the long-term use of these drugsCitation1,Citation2.
Analgesics, which are most widely used drugs for the treatment of pain, can be divided into two groups: morphine and related drugs and nonsteroidal anti-inflammatory drugs (NSAIDs). The fear of addiction and tolerance associated with morphine and related drugs has led to the restriction and withdrawal of these drugsCitation1–5.
NSAIDs act primarily by inhibiting cyclooxygenase (COX) enzymes, which catalyze the first step in the prostaglandin biosynthesis. The long-term use of NSAIDs may also lead to severe gastrointestinal side effects, which limit the use of these drugs. The adverse effects accompanying the use of non-selective NSAIDs arise from the reduction of the levels of protective prostaglandins in the gastrointestinal (GI) tract due to the inhibition of COX-1. Although selective COX-2 inhibitors cause less GI adverse effects than nonselective NSAIDs, their use in the treatment is also limited due to their serious cardiovascular effectsCitation1–5.
From the above discussion, it is clear that the search for new effective compounds has gained great importance. Acetamide derivatives have been found to possess analgesic activity. Paracetamol, which is one of the world’s most widely used drugs, is an example of analgesic agents bearing acetamide groupCitation6–9.
Medicinal chemists have carried out considerable research for novel analgesic agents which possess carboxylic acid moiety. The prominent compounds bearing carboxylic acid group are aspirin, ibuprofen and naproxen, all of which are widely used as over-the-counter drugs for the alleviation of pain. Many researchers have also studied tetrazoles extensively due to the fact that new effective compounds can be obtained by the bioisosteric replacement of the carboxylic acid group with tetrazole ring. Numerous studies have confirmed that tetrazole derivatives possess analgesic activityCitation2,Citation10–15.
Compounds bearing benzothiazole moiety have also been reported to exhibit a wide spectrum of biological effects including analgesic activityCitation16–20.
In this present study, we described the synthesis of some acetamide derivatives, which possess two functional moieties, namely benzothiazole and tetrazole and focused on the potential analgesic properties of these novel compounds.
Methods
Chemistry
All reagents were used as purchased from commercial suppliers without further purification. Melting points were determined using Electrothermal 9100 digital melting point apparatus and were uncorrected (Electrothermal, Essex, UK). The compounds were checked for purity by TLC on silica gel 60 F254. Spectroscopic data were recorded on the following instruments: IR, Shimadzu 435 IR spectrophotometer (Shimadzu, Tokyo, Japan); 1H NMR, Bruker 250 MHz and 13C NMR, Bruker 100 MHz NMR spectrometer (Bruker Bioscience, Billerica, MA) in DMSO-d6 using TMS as internal standard; MS-FAB, VG Quattro mass spectrometer (Fisons Instruments Vertriebs GmbH, Mainz, Germany). Elemental analyses were performed on a Perkin-Elmer EAL 240 elemental analyser (Perkin-Elmer, Norwalk, CT).
General procedure for synthesis of the compounds
N-(benzothiazol-2-yl)-2-chloroacetamides (1a–e)
Chloroacetyl chloride (5 mmol) was added dropwise with stirring to a mixture of 2-aminobenzothiazole (5 mmol) and triethylamine (2 mL) in toluene (50 mL) at 0−5°C. The solvent was evaporated under reduced pressure. The residue was washed with water and crystallized from ethanolCitation20.
N-(benzothiazol-2-yl)-2-[(1-substituted-1H-tetrazol-5-yl)thio]acetamide derivatives (2a–j)
A mixture of N-(benzothiazol-2-yl)-2-chloroacetamide (1) (2 mmol) and tetrazol-5-thiole (2 mmol) in acetone was stirred at room temperature for 8 h in the presence of potassium carbonate and filtered. The residue was washed with water and crystallized from ethanolCitation21.
N-(6-chlorobenzothiazol-2-yl)-2-[(1-phenyl-1H-tetrazol-5-yl)thio]acetamide (2b)
IR (KBr) νmax (cm−1): 3262 (amide N-H), 1715 (amide C=O), 1675, 1552, 1385 (C=N, N=N and C=C). 1H NMR (250 MHz, DMSO-d6): 4.54 (2H, s), 7.47 (1H, dd, J = 8.8, 2.2 Hz), 7.65-7.75 (5H, m), 7.77 (1H, d, J = 8.7 Hz), 8.13 (1H, d, J = 2.2 Hz), 12.90 (1H, m). 13C NMR (100 MHz, DMSO-d6): 36.48 (CH2), 121.48 (CH), 121.90 (CH), 124.41 (2CH), 126.56 (CH), 127.79 (C), 130.09 (2CH), 130.72 (CH), 132.92 (C), 133.11 (C), 147.36 (C), 153.69 (C), 158.47 (C), 166.52 (C). For C16H11ClN6OS2 calculated: 47.70% C, 2.75% H, 20.86% N; found: 47.74% C, 2.75% H, 20.87% N. MS (FAB) [M+1]+: m/z 403.
N-(6-chlorobenzothiazol-2-yl)-2-[(1-methyl-1H-tetrazol-5-yl)thio]acetamide (2g)
IR (KBr) νmax (cm−1): 3230 (amide N-H), 1682 (amide C=O), 1627, 1542, 1392 (C=N, N=N and C=C). 1H NMR (250 MHz, DMSO-d6): 3.90 (3H, s), 4.55 (2H, s), 7.20-7.91 (3H, m), 12.59 (1H, m). 13C NMR (100 MHz, DMSO-d6): 33.65 (CH3), 36.24 (CH2), 103.68 (CH), 115.25 (CH), 122.01 (CH), 132.67 (C), 142.22 (C), 152.09 (C), 154.69 (C), 158.17 (C), 166.81 (C). For C11H9ClN6OS2 calculated: 38.77% C, 2.66% H, 24.66% N; found: 38.80% C, 2.67% H, 24.70% N. MS (FAB) [M+1]+: m/z 341.
Pharmacology
Animals
Swiss albino male mice, weighing 35–40 g, were housed at room temperature of 24 ± 1°C with 12/12-h light/dark cycle. Twelve hours before each experiment, animals received only water in order to avoid food interference with substances absorption. The experimental protocols have been approved by the Local Ethical Committee on Animal Experimentation of Eskişehir Anadolu University, Turkey.
Assessment of analgesic activity
Hot-plate test
Antinociceptive activities of the tested compounds (2a–j) against thermal noxious stimuli were measured by hot-plate test in mice as described previouslyCitation22. Mice were placed individually on a hot-plate analgesiameter (Ugo Basile, Italy, No. 7280) which was set at 55 ± 1.0°C and the time of licking the forepaws or eventually jumping were recorded as a parameter of nociception. Maximum cut-off time was chosen as 30 s to avoid tissue damage. Response latencies were measured 30 min after the application of sunflower oil as control; morphine sulphate (10 mg/kg) as reference drug and each test compounds (100 mg/kg). The effects of the compounds on nociception were determined by converting the hot-plate latencies to percentage analgesic activity according to the following equation:
Tail-clip test
Antinociceptive activities of the tested compounds (2a–j) against mechanical noxious stimuli were measured by tail-clip test in mice as described previouslyCitation23. A metal artery clamp was applied to the tail of mouse and the time spent before biting the clamp was recorded by a stopwatchCitation24. A sensitivity test was carried out before the experimental session, and animals that did not respond to the clamp within 10 s were discarded from the experimentsCitation25. Maximum latency time (cut-off time) for the tail-clip tests was chosen as 10 s to avoid possible tissue damageCitation26. Analgesia was expressed as a percentage of the maximum possible effect (MPE %), according to the following equationCitation27:
Writhing test
Antinociceptive activities of the tested compounds (2a–j) against chemical noxious stimuli were measured by acetic acid-induced writhing test in mice as described previouslyCitation22. Mice were pre-treated with sunflower oil, morphine sulphate or test compounds (100 mg/kg, i.p.) 30 min prior to intraperitoneal injection of 0.6% acetic acid (Merck, Brazil) at a dose of 10 mL/kg. Five minutes after the injection of acetic acid, the number of abdominal contractions and stretches during the following 10 min was recorded. The percentage protection of writhing was calculated according to the following formula:
Assessment of motor coordination via Rota-Rod test
For evaluating any non-specific muscle-relaxant or motor coordination impairing effects of the tested compounds (2a–j), mice were submitted to the Rota-Rod task as described previouslyCitation22. Before the experimental session, three trials were given for three consecutive days on the Rota-Rod apparatus (Ugo Basile 7560, Milano, Italy) set at a rate of 16 revolutions per minute. Mice that were able to remain on the rod longer than 180 s were selected for the test. Each mouse was tested in the Rota-Rod and latency to fall from the rotating mill was recorded.
Statistical analysis
The data used in statistical analysis was obtained from six animals for each of the groups. Experimental data from behavioural tests were analysed by one-way analysis of variance followed by Tukey’s test. Statistical evaluation of the data was performed using GraphPad Prism 3.0 (GraphPad Software, San Diego, CA). Experimental results were expressed as mean ± SEM. Differences between given sets of data were considered to be significant when p value was less than 0.05.
Results
Initially, 2-chloro-N-(benzothiazol-2-yl)acetamides (1a–e) were obtained by reacting 2-aminobenzothiazoles with chloroacetyl chloride in the presence of triethylamine, which was responsible for the removal of hydrogen chloride from the reaction mixture.
The desired compounds (2a–j) were prepared by reacting 2-chloro-N-(benzothiazol-2-yl)acetamides with appropriate tetrazol-5-thioles. This nucleophilic substitution reaction was carried out in the presence of potassium carbonate. These reactions are summarized in .
The structures of the compounds (2a–j) were confirmed by IR, 1H-NMR, 13C-NMR and FAB+-MS spectral data and elemental analyses.
In the IR spectra, all derivatives have a strong characteristic band in the region 1715–1650 cm−1 due to the amide C=O stretching vibration. In the 1H-NMR spectra of all compounds (2a–j), the signal due to the amide proton appears at 12–13 ppm. In their 13C-NMR spectra, the signal due to the amide carbon is observed at 166–167 ppm. In the mass spectra of all compounds, the M+1 peak is observed. All compounds gave satisfactory elemental analysis.
All of the test compounds at 100 mg/kg doses caused significant increases in the reaction times of mice against thermal noxious stimulus in hot-plate tests. Compounds 2b, 2e, 2g, and 2j exhibited the highest antinociceptive actions ().
Figure 1. Antinociceptive effects of morphine and test compounds. (A) Hot-plate test. (B) Tail-clip test. (C) Acetic acid induced writhing test. Values are given as mean ± SEM. Significancy against control values *p< 0.05, **p< 0.01, ***p< 0.001. One-way ANOVA, post-hoc Tukey’s test, n = 6.
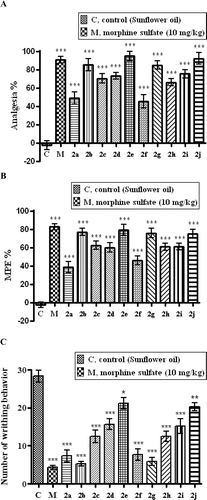
The test compounds also increased the reaction times of mice in tail-clip tests against mechanical noxious stimuli. Compounds 2b, 2e, 2g, and 2j were again the most effective in the tail-clip tests ().
In writhing tests, all test compounds prevent the animals from acetic acid-induced abdominal contractions and stretches. Chloride derivative compounds 2b and 2g and non-substituted derivatives 2a and 2f were the most active compounds among the test compounds (). Percentage protection values induced by each of the compounds were shown in .
Table 1. Protection percentage values of morphine and test compounds (2a–j) in acetic acid-induced writhing test.
No significant change was observed in the falling latencies of animals in Rota-Rod tests (data not shown).
Discussion
The results of this present study exhibited the significant antinociceptive activities for all of the test compounds (2a–j). Antinociceptive activities observed in all hot-plate, tail-clip and acetic acid-induced writhing tests clearly showed the pharmacological effects of these compounds on thermal, mechanical and chemical nociceptive pathways.
Hot-plate and tail-clip tests have been reported as a measure of centrally mediated transient pain. As reported previously, the hot-plate test predominantly measures responses organized supraspinally, whereas the tail-clip test mainly measures spinal reflexesCitation27,Citation28. As test compounds showed significant analgesic activities in both hot-plate and tail-clip tests, it may be suggested that analgesic activities observed in the present study are related to both supraspinal and spinal mechanisms.
Compounds 2e and 2j carrying ethoxy group as well as 2b and 2g carrying chloride group on their benzothiazole rings exhibited the highest antinociceptive actions in both hot-plate and tail-clip tests. On the other hand, unsubstituted compounds 2a and 2f exhibited relatively lower antinociceptive actions in both tests. Observed results can be depended on the lipophilic character of the compounds since the ethoxy and chloro substitutions increase the lipophilicity of the structure when compared with the other substituents. Depending on this respect, it may be declared that ethoxy and chloro substituents distinguish an optimum lipophilicity, which is a key property that influences the ability of a drug to reach the target by transmembrane diffusion and to have a major effect on the biological activity.
The acetic acid-induced abdominal construction is a visceral pain model employed as a test tool for the assessment of antinociceptive activities of new analgesic compoundsCitation29. It has been suggested that acetic acid injection into peritoneal cavity leads to an increased level of COX and lipooxygenase products in peritoneal fluids and the release of various inflammatory mediators such as bradykinin, substance P, TNF-α, IL-1β, IL-8, which eventually excites the primary afferent nociceptors entering dorsal horn of the central nervous systemCitation30. Therefore, inhibition of acetic acid-induced writhing behaviour after the administration of test compounds suggests that the mechanisms of the compounds may at least partly be related to the inhibition of COX and/or LOX and other inflammatory mediators in peripheral tissues, thereby interfering with the mechanism of signal transduction in primary afferent nociceptors. Chloride derivative compounds 2b and 2g and non-substituted derivatives 2a and 2f were the most active compounds among the test compounds. On the other hand, compounds 2e and 2j carrying ethoxy group, which were quite active in hot-plate and tail-clip tests, exhibited relatively lower antinociceptive actions in the writhing test. Greater analgesic activity of the compounds 2b, 2g, 2a and 2f with respect to 2e and 2j may be related with electronic features of the molecules. Compounds 2b and 2g carry an electron withdrawing chloro substituent and 2a and 2f are in non-substituted forms. However, less active compounds 2c, 2h, 2d, 2i, 2e and 2j bear electron donating substituents such as methyl, methoxy and ethoxy, which increase the electron density of the compounds. Therefore, it may be suggested that substitution with electron donating groups decreases, whereas electron withdrawing chloro group increases the peripheral analgesic activity of the compounds.
As benzothiazole ring, tetrazole ring was also substituted in the present study. However, the substitution of tetrazole ring with methyl or phenyl group did not cause any significant differences in the pharmacological activity.
Tested compounds did not impair the motor performance in Rota-Rod test, indicating that the observed antinociception unlikely occurred due to motor abnormalities.
Conclusion
The antinociceptive evaluations of some acetamide derivatives were demonstrated in the present study. The compounds (2a–j) exhibited statistically significant antinociceptive activities against thermal, mechanical, and chemical noxious stimuli in hot-plate, tail-clip and acetic acid-induced writhing tests, respectively. Based on these findings, it can be concluded that all the compounds (2a–j) act on all thermal, mechanical, and chemical nociceptive pathways and effect supraspinal, spinal and peripheral nociceptive mechanisms.
The findings of this present investigation prove the hypothesis that acetamide derivatives carrying benzothiazole and tetrazole rings possess antinociceptive activities and support the previous papers reporting the antinociceptive activities of various benzothiazole, tetrazole or acetamide derivativesCitation6–20.
Declaration of interest
The authors report no conflicts of interest. The authors alone are responsible for the content and writing of the paper.
References
- Moore ND. In search of an ideal analgesic for common acute pain. Acute Pain 2009;11:129–137.
- Buschmann H, Christoph T, Friderichs E, Maul C, Sundermann B. Analgesics: From chemistry and pharmacology to clinical application. Weinheim: Wiley-VCH Germany, 2002: pp. 1–264.
- Turunen JH, Mäntyselkä PT, Kumpusalo EA, Ahonen RS. Frequent analgesic use at population level: Prevalence and patterns of use. Pain 2005;115:374–381.
- Simmons DL, Botting RM, Hla T. Cyclooxygenase isozymes: The biology of prostaglandin synthesis and inhibition. Pharmacol Rev 2004;56:387–437.
- Dannhardt G, Laufer S. Structural approaches to explain the selectivity of COX-2 inhibitors: Is there a common pharmacophore? Curr Med Chem 2000;7:1101–1112.
- Moore A, Collins S, Carroll D, McQuay H. Paracetamol with and without codeine in acute pain: A quantitative systematic review. Pain 1997;70:193–201.
- Onkol T, Dogruer DS, Ito S, Sahin MF. Synthesis and antinociceptive activity of (5-chloro-2-benzothiazolinon-3-yl)acetamide derivatives. Arch Pharm (Weinheim) 2000;333:337–340.
- Pilli HG, Ozkanli F, Safak C, Erdogan H, Unlü S, Gümüsel B et al. 2-(6-Acyl-2-benzoxazolinone-3-yl)acetamide and acetonitrile derivatives with analgesic activities. Pharmazie 1994;49:63–64.
- Dogruer DS, Unlü S, Yesilada E, Fethi Sahin M. N-(2-pyridinyl)-2-[2(3H)-benzazolone-3-yl]acetamides: Synthesis, antinociceptive and anti-inflammatory activity. Farmaco 1997;52:745–750.
- Juby PF, Hudyma TW, Brown M. Preparation and antininflammatory properties of some 5-(2-anilinophenyl)tetrazoles. j Med Chem 1968;11:111–117.
- Bachar SC, Lahiri SC. Synthesis of chloro and bromo substituted 5-(indan-1′-yl)tetrazoles and 5-(indan-1′-yl)methyltetrazoles as possible analgesic agents. Pharmazie 2004;59:435–438.
- Ciapetti P, Giethlen B. Molecular variations based on isosteric replacements. In: Wermuth CM (ed.). The Practice of Medicinal Chemistry, Burlington: Academic Press USA, 2008: pp. 290–342.
- Rajasekaran A, Thampi PP. Synthesis and analgesic evaluation of some 5-[β-(10-phenothiazinyl)ethyl]-1-(acyl)-1,2,3,4-tetrazoles. Eur j Med Chem 2004;39:273–279.
- Vicini P, Amoretti L, Barocelli E, Chiavarini M, Impicciatore M. Synthesis and anti-inflammatory, antipyretic and analgesics properties of 5-(1,2-benzisothiazolyl)tetrazoles. Farmaco Sci 1986;41:111–118.
- Shanmugapandiyan P, Ramesh A. Synthesis and biological applications of certain 1-acetamido-(benzothiazol-2′-yl)-5-aryltetrazole and benzothiazol-2′-yl-1-ethylamine-5-aryltetrazoles. J Pharm Chem 2008;2:169–173.
- Paramashivappa R, Phani Kumar P, Subba Rao PV, Srinivasa Rao A. Design, synthesis and biological evaluation of benzimidazole/benzothiazole and benzoxazole derivatives as cyclooxygenase inhibitors. Bioorg Med Chem Lett 2003;13:657–660.
- Yous S, Poupaert JH, Chavatte P, Espiard JG, Caignard DH, Lesieur D. Synthesis and pharmacological evaluation of analgesic 6-substituted 2(3H)-benzothiazolones. Drug Des Discov 2001;17:331–336.
- Gökce M, Cakir B, Erol K, Sahin MF. Synthesis and antinociceptive activity of [(2-oxobenzothiazolin-3-yl)methyl]-4-alkyl/aryl-1,2,4-triazoline-5-thiones. Arch Pharm (Weinheim) 2001;334:279–283.
- Bahekar SS, Shinde DB. Synthesis and anti-inflammatory activity of [2-(benzothiazol-2-ylimino)-4-oxo-3-phenylthiazolidin-5-yl]-acetic acid derivatives. J Korean Chem Soc 2003;47:237–240.
- Bhargava PN, Ram P. Synthesis of local anesthetics. Bull Chem Soc Japan 1965;38:339–341.
- Connell RD, Lease TG, Ladouceur GH, Osterhout MH. α-Alkoxy- and α-thioalkoxyamide neuropeptide Y NPY5 receptor antagonists and therapeutic methods using them. US Patent 1999;5939462A 19990817.
- Kaplancikli ZA, Turan-Zitouni G, Ozdemir A, Can O, Chevallet P. Synthesis and antinociceptive activities of some pyrazoline derivatives. Eur J Med Chem 2009;44:2606–2610.
- Can OD, Ozkay UD, Oztürk N, Oztürk Y. Effects of hawthorn seed and pulp extracts on the central nervous system. Pharm Biol 2010;48:924–931.
- D’Amour FE, Smith DL. A method for determining loss of pain sensation. J Pharmacol Exp Ther 1941;72:74–79.
- Adeyemi OO, Okpo SO, Okpaka O. The analgesic effect of the methanolic extract of Acanthus montanus. J Ethnopharmacol 2004;90:45–48.
- Oztürk N, Baser KH, Aydin S, Oztürk Y, Calis I. Effects of Gentiana lutea ssp. symphyandra on the central nervous system in mice. Phytother Res 2002;16:627–631.
- Gabra BH, Sirois P. Beneficial effect of chronic treatment with the selective bradykinin B1 receptor antagonists, R-715 and R-954, in attenuating streptozotocin-diabetic thermal hyperalgesia in mice. Peptides 2003;24:1131–1139.
- Wong CH, Dey P, Yarmush J, Wu WH, Zbuzek VK. Nifedipine-induced analgesia after epidural injection in rats. Anesth Analg 1994;79:303–306.
- De Souza MM, Pereira MA, Ardenghi JV, Mora TC, Bresciani LF, Yunes RA et al. Filicene obtained from Adiantum cuneatum interacts with the cholinergic, dopaminergic, glutamatergic, GABAergic, and tachykinergic systems to exert antinociceptive effect in mice. Pharmacol Biochem Behav 2009;93:40–46.
- Ikeda Y, Ueno A, Naraba H, Oh-ishi S. Involvement of vanilloid receptor VR1 and prostanoids in the acid-induced writhing responses of mice. Life Sci 2001;69:2911–2919.