Abstract
This study was designed to investigate the mushroom tyrosinase inhibitory activity for the constituents isolated from Neolitsea aciculata. The stems of N. aciculata was extracted with aqueous ethanol and subjected to chromatographic separation, which led to the isolation of 11 compounds: methyl linoleate (1), catechin (2), epicatechin (3), afzelin-7-O-glucopyranoside (4), 2′,3′-di-(p-coumaroyl)afzelin (5), 2′-p-coumaroylafzelin (6), feruloyl tyramine (7), β-sitosterol (8), daucosterol (9), oleic acid (10), and trilaurin (11). Their structures were elucidated on the basis of spectroscopic studies as well as by comparison with the data available in the literature. Among these isolates, compounds 5 and 6 were identified as potent mushroom tyrosinase inhibitors with IC50 values of 0.067 and 0.080 mM, respectively. The inhibition kinetics, analysed by Lineweaver–Burk plots, indicated that compounds 5 and 6 are competitive tyrosinase inhibitors when using l-tyrosine as a substrate. Notably, compounds 1–11 were isolated for the first time from this plant. These results provide evidence that this plant might be a potential source of anti-melanogenesis agents.
Introduction
Tyrosinase (E.C.1.14.18.1) is a copper containing metalloenzyme and is distributed widely in mammals, fungi and plants. This enzyme plays a crucial role in the production of melanin pigment. The first step in the biosynthesis of melanin is the oxygen-mediated hydroxylation of l-tyrosine to generate 3,4-dihydroxyphenylalanine (l-DOPA). The resulting l-DOPA is oxidized again to yield an o-quinone (DOPA quinone)Citation1. These two steps are catalyzed by tyrosinase and are the rate-determining steps in melanin synthesis. As tyrosinase plays a key role in the synthesis of melanin, the inhibition of this enzyme is an effective method of down-regulating the melanin formation.
Melanin pigment is generated in the melanocytes of human skin upon exposure to external ultraviolet (UV) radiation, which is an essential physiological response to protect the inner skin from deleterious UV damage. However, the overproduction and accumulation of dark melanin in the skin result in hyperpigmentation disorders such as melasma, freckles and ageCitation2. Therefore, the regulation of melanin synthesis is of importance from both a medicinal and aesthetic point of view.
Currently, tyrosinase inhibitory chemicals such as arbutin, kojic acid, and hydroquinone derivatives are used clinically for the treatment of hyperpigmentation. However, public concerns have led to questions being raised regarding their low activities and cell toxicitiesCitation3. Accordingly, the development of novel anti-tyrosinase agents exhibiting high efficiency and low toxicity is still necessary for skin-whitening productsCitation4–6. Regarding the use of tyrosinase inhibitors in cosmetics, much of the effort made so far has focused on natural products from the plant kingdom due to their environmentally friendly propertiesCitation7–9.
With the aim of discovering active ingredients for use in cosmetic preparations, we are continuously investigating the extracts from plants growing in Jeju IslandCitation10,Citation11. Located in the southernmost part of Korea, Jeju Island has ecological diversity with over 1,800 plant speciesCitation12. In our previous study, the ethanol extract ofNeolitsea aciculata possessing anti-tyrosinase activities has been evaluated without identification of the active constituentsCitation13. Presently, we sought to isolate the active compounds from N. aciculata ethanol extract. The isolated compounds were tested for the mushroom tyrosinase inhibitory activities, and were kinetically analysed to determine the mechanism of action.
Neolitsea is a genus of 80 species of evergreen shrubs and small trees belonging to the Lauraceae family. They range from topical Asia, Malesia to Australia. N. aciculata (Blume) Koidz is a woody plant distributed widely in Northeast Asia including Korea, Japan and China. Sesquiterpenes, triterpenes and sterols have been identified as chemical constituents from this plant in previous phytochemical studiesCitation14,Citation15. Antibacterial and anti-inflammatory activities of essential oils from the leaves of N. aciculata have been characterizedCitation10.
Materials and methods
Reagents and equipment
A Biochrom Libra S22 UV-visible spectrophotometer was used for the collection of the UV spectra. The 1H (400 MHz) and 13C (100.60 MHz) NMR spectra were recorded on a JEOL (JNM-ECX 400) spectrometer with the chemical shift data reported in ppm relative to the solvent used. Vacuum liquid chromatography (VLC) and column chromatography (CC) were performed using silica gel 60H (15 µm, Merck) and silica gel (0.063–0.2 mm), respectively. The silica gel 60 F254 coated on aluminum plates used for thin layer chromatography was supplied by Merck. Gel filtration chromatography was performed on Sephadex LH-20 (25–100 µm, Fluka).
Plant material
The stems of N. aciculatawere collected from the Jeju Botanical Garden, Jeju Island, Korea in May 2008. A voucher specimen (no. 068) is deposited at the Natural Product Chemistry Laboratory, Department of Chemistry, Jeju National University, Korea.
Extraction and isolation
The air-dried and pulverized branches of N. aciculata(1.0 kg) were extracted three times with 70% ethanol (v/v) (20.0 L) using a mechanical stirrer at room temperature for 24 h. The filtered ethanol solutions were concentrated using a rotary evaporator at a temperature not exceeding 45°C. The obtained gummy mass (50.0 g) was successively partitioned to give n-hexane (5.0 g), EtOAc (8.0 g), n-butanol (9.4 g), and water (14.8 g) soluble fractions.
A portion of the n-butanol-soluble fraction (5.0 g) was subjected to VLC through silica gel using n-hexane-EtOAc-methanol gradients to elute 10 fractions (Frs. V1 to V10). Fraction V2 (350 mg) was further purified by silica gel CC with chloroform-methanol (10:1) as the mobile phase to give compound 1 (7.0 mg). Fraction V5 (500 mg) was also further purified by silica gel CC with chloroform-methanol (3:1) to afford compound 4 (8.0 mg). A portion of the EtOAc-soluble fraction (3.0 g) was similarly subjected to VLC through silica gel using gradient solvents to afford 8 fractions (Fr. V1-1 to V1-8). Compound 2(10.0 mg) was obtained from Fr. V1-3 by silica gel CC with chloroform-methanol (6:1) eluent. Compound 3(15.0 mg) was obtained from Fr. V1-4 by silica gel CC with a hexane-chloroform-methanol (1:10:1) gradient. Another portion of the EtOAc-soluble fraction (5.0 g) was also similarly fractionated by VLC over silica gel using gradient solvents to afford 16 fractions (Frs. V2-1 to V2-15). Fraction V2-2 afforded the compound 5 (8.0 mg). Compounds 6(7.0 mg) and 7 (3.0 mg) were obtained from fraction V2-7 by silica gel CC using chloroform-methanol (6:1) eluent. Another portion of the EtOAc-soluble fraction (4.0 g) was also similarly subjected to VLC over silica gel to afford 10 fractions (Frs. V3-1 to V3-10). Compound 8(8.0 mg) was obtained from Fr. V3-2 by using silica gel CC with chloroform-methanol (4:1) eluent. Compounds 9(15.0 mg), 10 (15.0 mg) and 11 (10.0 mg) were obtained from Fr. V3-5 by silica gel CC using chloroform-methanol (4:1). The isolation scheme for the compounds 1–11 is provided in the supporting information.
Tyrosinase inhibition assay
The mushroom tyrosinase inhibition activities were determined according to the method described in the literature with some modificationsCitation16. Briefly, mushroom tyrosinase (1250 units/mL) and 2 mM l-tyrosine (0.07 mL) were added to a solution of phosphate buffer (0.1 M, pH 6.8, 0.09 mL) containing the test sample. The test mixture (0.2 mL) was incubated for 10 min at 37°C and the absorption due to the formation of dopachrome was monitored at 475 nm. The same mixture except for the plant extract was used as a control. Arbutin (hydroquinone-O-β-glucopyranoside) was used as a positive control. Each treatment was replicated three times. The percent inhibition of the tyrosinase activity was calculated as follows:
Where Abssample, Absblank and Abscontrol are the absorbances of the experimental sample, the blank, and the control, respectively. IC50 indicates the concentration of the sample when the enzyme activity was inhibited by 50%, as determined by linear curve fitting.
Kinetic analysis of tyrosinase inhibition
Four or five different concentrations of l-tyrosine (0.1–0.5 mM) were added as substrates to a 96-well plate containing 5 µL of aqueous solution of mushroom tyrosinase (1250 units) and 100 mM potassium phosphate buffer (pH 6.8) with (10, 20 and 40 µg/mL of the compounds) or without the test sample to a final volume of 200 µL. Using a microplate reader, the initial rate of dopachrome formation in the reaction mixture was determined based on the linear increase in absorbance at 475 nm. The Michaelis constant (Km) and maximal velocity (Vmax) of tyrosinase were determined by Lineweaver–Burk plots with l-tyrosine as the substrate.
Results and discussion
The stem parts of the tree N. aciculata were extracted with 70% aqueous ethanol according to the procedure described in the experimental section. Upon the preliminary screening tests on mushroom tyrosinase, inhibitory activities were observed in this extract when using l-tyrosine as the substrate. In order to identify the active constituents, phytochemical analysis was conducted. The ethanol extract was partitioned into n-hexane, ethyl acetate (EtOAc), n-butanol, and water soluble fractions. Among these, the EtOAc and n-butanol fractions were subjected to repeated CC over silica gel and Sephadex LH-20 leading to the isolation of compounds 1–11 (). The chemical structures of the isolates were identified by spectroscopic methods including 1D and 2D NMR measurements, as well as by comparing the obtained data to the literature values. These isolated compounds were classified into the following subgroups: lipid or fatty acids viz. methyl linoleate (1)Citation17, oleic acid (10)Citation18, and trilaurin (11)Citation19; catechin compounds viz. catechin (2)Citation20 and epicatechin (3)Citation21; flavonoid derivatives viz. afzelin-7-O-glucopyranoside (4)Citation22, 2′,3′-di-(p-coumaroyl)afzelin (5)Citation23, and 2′-p- coumaroylafzelin (6)Citation24; phytosterols viz. β-sitosterol (8)Citation25,Citation26 and daucosterol (9)Citation27; a phenylpropanoid viz. feruloyl tyramine (7)Citation28. To the best of our knowledge, all of the identified compounds (1–11) in were isolated for the first time from the tree N. aciculata.
The isolates (1–11) were examined for their anti-tyrosinase activities using l-tyrosine as the substrate. The test solutions were prepared for each compound with varying concentrations (25–100 µg/mL), and the increment of the absorbance at 475 nm due to the enzyme-mediated formation of dopachrome was monitored using a spectrophotometer (data not shown). Among compounds 1–11, compounds 5and 6 were identified as potent tyrosinase inhibitors (). They suppressed mushroom tyrosinase activities with IC50 values of 0.067 and 0.080, respectively. These results indicate that the activities of these compounds are about five times more potent than that of the positive control arbutin (IC50 = 0.32 ± 0.015 mM). Arbutin, a glycosylated hydroquinone extracted from a bearberry plant, is a commercial anti-tyrosinase skin-lightening agent used in cosmetic formulations. The other isolated compounds, however, displayed poor inhibitory activities, showing IC50 values of over 1.0 mM.
Figure 2. Comparison of 50% tyrosinase inhibition activities (IC50) of the isolated compound 5, 6 and positive control compound.
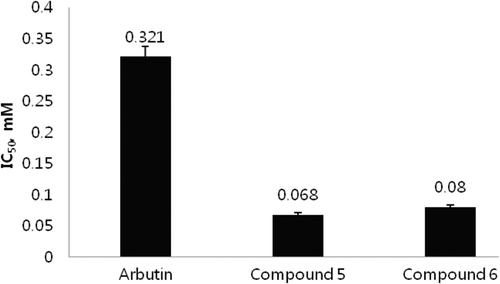
As compounds 5 and 6 displayed potent inhibition against the diphenolase activity of tyrosinase, their inhibition modes were investigated based on Lineweaver–Burk plot analysisCitation29. Compound 5 showed an identical Vmax value of 0.0487 A475/min at concentrations of 0, 0.14, 0.28, and 0.56 mM and its Km values at these concentrations were 0.83, 1.16, 1.58, and 2.63 mM, respectively (). As the concentration of the l-tyrosine substrate varied, the Km value of tyrosinase increased in a dose-dependent manner without any change in Vmax. This indicates that the kaempferol derivative (5) behaves as a competitive tyrosinase inhibitor. In the same manner, 2′-p- coumaroylafzelin (6) was also characterized as a competitive tyrosinase inhibitor using l-tyrosine substrate at concentrations of 0, 0.17, 0.34 and 0.7 mM. It showed the same Vmax value of 0.0467 A475/min and its Km values at these concentrations were 0.652, 1.19, 2.00, and 3.36 mM, respectively. Lineweaver–Burk plot for the compound 6 is provided in the supporting information. Compounds 5 and 6 exhibited inhibition constants (Ki) of 0.23 and 0.15 mM, respectively.
Figure 3. Lineweaver–Burk’s plot of mushroom tyrosinase and l-tyrosine without (♦) and with 0.14 (▪), 0.28 (▴), 0.56 (•) mM of the compound 5.
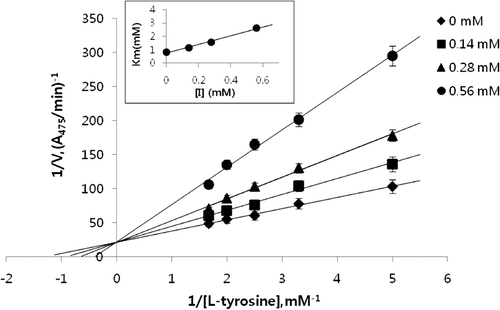
Tyrosinase is a metalloprotein with a dinuclear copper-binding domain in its active site. Its crystal structure confirmed the presence of three different metal forms in its active site, viz. the deoxy-form [Cu(I)-Cu(I)], oxy-from [Cu(II)-O2−2-Cu(II)] and met-form [Cu(II)-OH-Cu(II)]Citation30. As shown in the competitive inhibitor arbutin, monophenol-type inhibitors were assumed to exert their activities by binding to copper metal as a monophenolase substrateCitation31. Considering their competitive inhibition mode, it is suggested that the coordination of phenol functional groups in 5 and 6 to copper in the oxy-form of the enzyme induce the inhibition activities. It is interesting to analyse the structure–activity relationship using compounds 4–6. As the afzelin glycoside 4 did not exhibit any inhibition activities, the phenol in the B ring of the flavonoid is not likely to coordinate to the copper metal in the enzyme. It is reasonable to assume coumaroyl phenols in compounds 5 and 6 are binding to the active site leading to the enzyme inhibition. As the compounds 5 and 6 show the similar activities, the coumaroyls attached to C-2′ are more likely to be responsible to the inhibition activities.
In conclusion, during the investigation of the tyrosinase inhibitory constituents from the branches of N. aciculata, 12 compounds were isolated and characterized. Among the isolates, 2′,3′-di-(p-coumaroyl)afzelin (5) and 2′-p- coumaroylafzelin (6) were identified as the active inhibitory constituents toward mushroom tyrosinase. Further kinetic analysis indicated that compounds 5 and 6 have a competitive inhibitory effect on the L-tyrosine substrate. These results demonstrated that the ethanol extracts of N. aciculata stems might be a potential source of anti-melanogenesis agents.
Declaration of interest
This research was financially supported by the Ministry of Knowledge Economy (MKE) and Korea Institute for Advancement of Technology (KIAT) through the Research and Development for Regional Industry.
References
- Land EJ, Ramsden CA, Riley PA. Tyrosinase autoactivation and the chemistry of ortho-quinone amines. Acc Chem Res 2003;36:300–308.
- Uyen LDP, Nguyen DH, Kim EK. Mechanism of skin pigmentation. Biotechnol Bioprocess Eng 2008;13:383–395.
- Chang TS. An updated review of tyrosinase inhibitors. Int J Mol Sci 2009;10:2440–2475.
- Casañola-Martín GM, Marrero-Ponce Y, Khan MTH, Ather A, Khan KM, Torrens F et al. Dragon method for finding novel tyrosinase inhibitors: biosilico identification and experimental in vitroassays. Eur J Med Chem 2007;42:1370–1381.
- Khan KM, Maharvi GM, Khan MTH, Jabbar Shaikh A, Perveen S, Begum S et al. Tetraketones: a new class of tyrosinase inhibitors. Bioorg Med Chem 2006;14:344–351.
- Khan KM, Maharvi GM, Abbas Khan A, Hayat S, Khan MTH, Makhmoor T, Choudhary MI, Shaheen F, Atta-ur-Rahman. Three tyrosinase inhibitors and antioxidant compounds from Salsola foetida. Helvetica Chemica Acta 2003;86:457–469.
- Khan MT, Choudhary MI, Khan KM, Rani M, Atta-ur-Rahman. Structure-activity relationships of tyrosinase inhibitory combinatorial library of 2,5-disubstituted-1,3,4-oxadiazole analogues. Bioorg Med Chem 2005;13:3385–3395.
- Khan KM, Maharvi GM, Perveen S, Khan MT, Abdel-Jalil RJ, Shah ST et al. Synthesis of methyl ether analogues of sildenafil (Viagra) possessing tyrosinase inhibitory potential. Chem Biodivers 2005;2:470–476.
- Khan KM, Saify ZS, Khan MT, Butt N, Maharvi GM, Perveen S et al. Tyrosinase inhibition: conformational analysis based studies on molecular dynamics calculations of bipiperidine based inhibitors. J Enzyme Inhib Med Chem 2005;20:401–407.
- Kim SS, Kim JE, Hyun CG, Lee NH. Neolitsea aciculata essential oil inhibits drug-resistant skin pathogen growth and Propionibacterium acnes-induced inflammatory effects of human monocyte leukemia. Nat Prod Commun 2011;6:1193–1198.
- Ko RK, Kim GO, Hyun CG, Jung DS, Lee NH. Compounds with tyrosinase inhibition, elastase inhibition and DPPH radical scavenging activities from the branches of Distylium racemosum Sieb. et Zucc. Phytother Res 2011;25:1451–1456.
- Seo JC. A wild flower in Jeju Island. Il Jin Sa 2004;384–394.
- Kim SS, Yoon WJ, Hyun CG, Lee NH. Down-regulation of tyrodinase, TRP-2 and MITF expressions by Neolitsae aciculate extract in Murine B16F10 Melanoma. Int J Pharmacol 2010;6:290–295.
- Takeda K, Horibe I, Teraoka M, Minato H. Components of Neolitsea aciculata Kodiz. Structures and absolute configuration of litsealactone, litseaculane, zeylanine, and zeylanane. Chem commun 1968;940–942.
- Yano K, Akihisa T, Tamura T, Matsumoto T. Four 4α-methylsterols and triterpene alcohols from Neolitsea aciculata. Phytochemistry 1992;31:2073–2078.
- Vanni A, Gastaldi D, Giunata G. Kinetic investigation on the double enzymatic activity of the mushroom tyrosinase. Ann Chim 1991;80:35–60.
- Frankel EN, Neff WE, Weisleder D. Determination of methyl linoleate hydroperoxides by 13C nuclear magnetic resonance spectroscopy. Meth Enzymol 1990;186:380–387.
- Parks JS, Cistola DP, Small DM, Hamilton JA. Interactions of the carboxyl group of oleic acid with bovine serum albumin: a 13C NMR study. J Biol Chem 1983;258:9262–9269.
- Gunstone FD. 13C-NMR spectra of some synthetic glycerol esters alone and as mixtures. Chem Physics Lipids 1990;56:195–199.
- Bang MH, Song JC, Lee SY, Park NK, Beak NI. Isolation and structure determination of antioxidants from the root of Paeonia lactiflora. J Korean Soc Agric Chem Biotechnol 1999;42:170–175.
- Kang IH, Cha JH, Lee SW, Kim HJ, Kwon SH, Ham IH, Hwang BS, Whang KA. Isolation and anti-oxidant from domestic Crataegus pinnatifida Bunge leaves. Kor J Pharmacogn 2005;36:121–128.
- Kucukislamoglu M, Yayli N, Senturk HB, Genc H. Flavonol glycosides from Consolida armeniaca. Turk J Chem 2000;24: 191–197.
- Fathy MS, Shehata AH, Khaleel AE, Ezzat SM. An acylated kaempferol glycoside from flowers of Foeniculum vulgare and F. dulce. Molecules 2002;7:245–251.
- Nowak R. Separation and quantification of tiliroside from plant extracts by SPE/RP-HPLC. Pharmaceut Biol 2003;41:8.
- Kovganko NV, Kashkan ZN, Borisov EV, Batura EV. 13C NMR spectra of β-sitosterol derivatives with oxidixed rings A and B. Chem Nat Comp 1999;35:646–649.
- Hussain RA, Siddiqui HL, Khan KM, Sharif A. Phytochemical studies on Adiantum incisum. J Chem Soc Pakistan 2008;30:605–608.
- Lee DY, Song MC, Yoo JS, Kim SH, Chung IS, Kim DK, Park MH, Kwon BM, Kim SY, Baek NI. Development of biologically active compounds from edible plant sources-XVII. Isolation of sterols from the fruits of Cornus kousa Burg. J Korean Soc Appl Biol Chem 2006;49:82–85.
- Cutillo F, D’Abrosca B, DellaGreca MDi, Marino C, Golino A, Previtera L et al. Cinnamic acid amides from Chenopodium album: effects on seeds germination and plant growth. Phytochemistry 2003;64:1381–1387.
- Chang TS, Ding HY, Tai SSK, Wu CY. Mushroom tyrosinase inhibitory effects of isoflavones isolated from soygerm koji fermented with Aspergillus oryzae BCRC 32288. Food Chem 2007;105:1430–1438.
- Matoba Y, Kumagai T, Yamamoto A, Yoshitsu H, Sugiyama M. Crystallographic evidence that the dinuclear copper center of tyrosinase is flexible during catalysis. J Biol Chem 2006;281:8981–8990.
- Nihei K, Kubo I. Identification of oxidation product of arbutin in mushroom tyrosinase assay system. Bioorg Med Chem Lett 2003;13:2409–2412.