Abstract
The enzyme type 5 17β-hydroxysteroid dehydrogenase 5 (17β-HSD5) catalyzes the transformation of androstenedione (4-dione) to testosterone (T) in the prostate. This metabolic pathway remains active in cancer patients receiving androgen deprivation therapy. Since physicians seek to develop advantageous and better new treatments to increase the average survival of these patients, we synthesized several different dehydroepiandrosterone derivatives. These compounds have a pyrazole or imidazole function at C-17 and an ester moiety at C-3 and were studied as inhibitors of 17β-HSD5. The kinetic parameters of this enzyme were determined for use in inhibition assays. Their pharmacological effect was also determined on gonadectomized hamsters treated with Δ4-androstenedione (4-dione) or testosterone (T) and/or the novel compounds. The results indicated that the incorporation of a heterocycle at C-17 induced strong 17β-HSD5 inhibition. These derivatives decreased flank organ diameter and prostate weight in castrated hamsters treated with T or 4-dione. Inhibition of 17β-HSD5 by these compounds could have therapeutic potential for the treatment of prostate cancer and benign prostatic hyperplasia.
Introduction
Prostatic cancer recurrence has been observed in some castrated patients as well as in patients under androgen ablation therapiesCitation1. The growth of these tumors could be determined by the adaptive changes of androgen receptors (AR) such as amplifications or mutations, which have been previously describedCitation2–5. However, most prostate cancers are produced in an androgen-dependent manner, and the presence of intraprostatic dihydrotestosterone (DHT) could stimulate AR signaling, since this steroid is only reduced by close to 60%Citation4,Citation6 with medical castration using GnRH analogues.
Prostatic tissue is capable of synthesizing DHT either de novo or from weak androgens, dehydroepiandrosterone (DHEA) and androstenedione (4-dione) synthesized the by adrenal glands. These androgens are also present in the plasma of cancer patients under androgen ablation therapiesCitation5. Therefore, the synthesis of DHT from DHEA and 4-dione is possible, since the enzymes involved in this pathway are increased in the prostates of these patientsCitation2–5.
The enzyme 17β-HSD type 5 (17β-HSD5) is well characterized in human prostate tissues, and contributes to local androgen formationCitation7,Citation8. The gene that encodes 17β-HSD5 has been identified in bands 10 p15 and p14 of human chromosome 13 from prostate cells and its mRNA is over-expressed in prostate cancer tissue (77%)Citation5–8. Since this enzyme is produced locally in prostatic cancer tumors, it must be considered as a therapeutic target for this illnessCitation9.
During the conversion of 4-dione to T (), conformational changes of 17β-HSD5 have been observed by X-ray analysisCitation8. This fact has inspired researchers to design new drugs intended to inhibit the activity of this enzymeCitation5,Citation7,Citation10,Citation11.
Figure 1. Conversion of androstenedione (4-dione) to testosterone (T)Citation22.
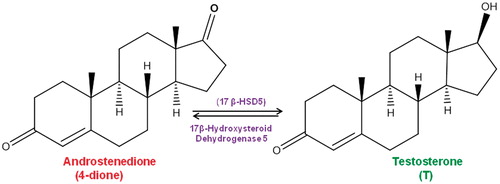
Studies carried out with the prostate cancer cell lines DU-145, PC-3 and LNCaPCitation3–6 showed increased reductive activity for 17β-HSD5 in these cell linesCitation9. 17β-HSD5 activity could therefore be relevant in the growth and development of cancerous tumorsCitation5–10. Thus the inhibition of this enzyme could offer a new alternative for prostate cancer treatment as compared to currently used endocrine therapies.
Currently, 20 crystalline isoforms of 17β-HSD5 are known; X-ray analysis of these structures has revealed the presence of pockets and subpockets inside these proteinsCitation5,Citation6,Citation8,Citation9. On the basis of these findings, a large number of steroidal and non-steroidal 17β-HSD5 inhibitors have been synthesizedCitation5–10. Recently, we prepared several new dehydroepiandrosterone derivatives that have a pyrazole group at C-17; some of these compounds entirely inhibited the proliferation of PC-3 cellsCitation11. Although the PC-3 prostate cancer cell line exhibits some androgen resistanceCitation12, these cells express 17β-HSD2 mRNA and show oxidative activityCitation13,Citation14. On the basis of these facts, we propose that dehydroepiandrosterone derivatives with a pyrazole group at C-17 could inhibit the activity of 17β-HSD5Citation15.
On the basis of these studies, we synthesized several pyrazole–dehydroepiandrosterone derivatives (4a–4f, ): 16-formyl-17-(1H-pyrazole-1-yl)-androsta-5,16-dien-3β-yl-cyclopropanoate (4a), 16-formyl-17-(1H-pyrazole-1-yl)-androsta-5,16-dien-3β-yl-cyclobutanoate (4b), 16-formyl-17-(1H-pyrazole-1-yl)-androsta-5,16-dien-3β-yl-cyclopentanoate (4c), 16-formyl-17-(1H-pyrazole-1-yl)-androsta-5,16-dien-3β-yl-cyclohexanoate (4d), 16-formyl-17-(1H-pyrazole-1-yl)-androsta-5,16-dien-3β-yl-cycloheptanoate (4e) and the previously synthesized steroid 16-formyl-17-(1H-pyrazole-1-yl)androsta-5,16-diene-3β-yl-p-fluorobenzoate (4f)Citation11.
It has been demonstrated that when the nitrogen atom takes different positions in the pyrazole ring (several isomers are possible), variations in biological activity are observed. Either isomer (pyrazole or imidazole rings) can form a stable complex with the active site of some enzymes, such as activin-like kinase 5 (ALK5), thus inhibiting their activity in cell-based luciferase reporter assays, with higher activity of the pyrazole ringCitation13. On the basis of this, we synthesized the imidazole derivative 7 (16-formyl-17-(1H-1,3-imidazole-1-yl)-androsta-5,16-dien-3β-yl-acetate) with the aim of comparing its biological activity with the pyrazole derivatives (4a–4f).
Materials and methods
Chemical and radioactive materials
Solvents were of laboratory grade. Melting points (uncorrected) were determined on a melting point apparatus (Fisher Johns, Mexico City, Mexico); 1H NMR and 13C NMR were taken on Varian Gemini 200 and VRX-300 spectrometers, respectively (MR Resources, Fitchburg, MA). Chemical shifts are given in ppm relative to that of Me4Si (δ = 0) in CDCl3 (the abbreviations of signal patterns are as follows: s, singlet; d, doublet; t, triplet; m, multiplet). Mass spectra were obtained with an HP5985-B spectrometer (Avantes, Apeldoorn, The Netherlands). IR spectra were obtained using a Perkin-Elmer 200s spectrometer (Perkin Elmer Life and Analytical Science, Shelton, CT). The UV lamp (254 nm) was from UVP (Upland, CA). UV spectra were determined on a Perkin-Elmer Lambda 2 apparatus using methanol as a solvent. The purity of the synthesized compounds was corroborated by TLC, melting point and Perkin-Elmer High Resolution Mass Spectrometry (HSMS).
(1,2,6,7-3H) T specific activity: 95 Ci/mmol and (1,2,6,7-3H) androstenedione (4-dione) specific activity 98.2 Ci/mmol were supplied by Perkin Elmer Life and Analytical Science (Boston, MA). Radioinert T and 4-dione were supplied by Steraloids (Wilton, NH). Sigma Chemical Co. (St. Louis, MO) provided NADPH and Lubrol PX. Finasteride was obtained by extraction from Proscar (Merck, Sharp and Dohme). The tablets were crushed and extracted with chloroform, and the solvent was eliminated in vacuum; the crude product was purified by silica gel column chromatography. The melting point of the isolated Finasteride (252–254 °C) was identical to that reported in the literatureCitation16.
Synthesis of the steroidal derivatives: overview
For the synthesis of the new steroidal compounds 4a–4e and 7 (), we used commercially available dehydroepiandrosterone (1) and also its acetate (5). Compounds 2f, 3f and 4f were prepared according to the procedure reported previouslyCitation11. The synthesis of the new compounds is briefly described below.
General preparation of esters (2a–2e)
The corresponding acid (14 mmol) was added to a solution of steroid 1 (1 g, 3 mmol), N,N'-dicyclohexylcarbodiimide (2.147 g, 10.41 mmol), 4-dimethylaminopyridine (0.848 g, 6.94 mmol) in chloroform (20 mL). The resulting solution was stirred at room temperature for 2 h. Hexane (15 mL) was added and the precipitated dicyclohexyl urea was filtered. The organic phase was washed three times with 10% aqueous hydrochloric acid, 5% aqueous sodium bicarbonate and water, and was dried over anhydrous sodium sulfate. The crude ester was recrystallized from methanol.
17-Oxaandrost-5-ene-3β-yl-cyclopropanoate (2a): Yield: 85%. m.p. 197–198 °C. IR (KBr) cm−1: 2947, 1736, 1712. 1H NMR (300 MHz, CDCl3) δ: 0.9 (3H, s, H-18), 1.1 (3H, s, H-19), 4.6 (1H, m, 3α-H), 5.4 (1H, dd, J1 = 4 Hz and J2 = 2 Hz, H-6). 13C NMR (100 MHz, CDCl3) δ: 8.3 (2C-cyclopropyl), 13.2 (C-cyclopropyl), 19.3 (C-18), 20.3 (C-19), 73.6 (C-3), 121.7 (C-6), 139.9 (C-5), 162.2 (C-17), 174.2 (ester carbonyl), 220.9 (C-17). HRMS calcd for C23H32O3 356.2351, found 356.2299.
17-Oxaandrost-5-ene-3β-yl-cyclobutanoate (2b): Yield: 84%. m.p. 180–181 °C. IR (KBr) cm−1: 2942, 1735, 1720. 1H NMR (300 MHz, CDCl3) δ: 0.9 (3H, s, H-18), 1.0 (3H, s, H-19), 4.6 (1H, m, 3α-H), 5.4 (1H, dd, J1 = 4 Hz and J2 = 2 Hz, H-6). 13C NMR (100 MHz, CDCl3) δ: 14.6 (C-cyclobutyl), 18.2 (C-cyclobutyl), 19.3 (C-18), 20.3 (C-19), 25.0 (2C-cyclobutyl), 73.3 (C-3), 121.6 (C-6), 139.9 (C-5), 174.8 (ester carbonyl), 220.9 (C-17). HRMS calcd for C24H34O3 370.2508 found 370.2556.
17-Oxaandrost-5-ene-3β-yl-cyclopentanoate (2c): Yield: 88%. m.p. 175–177 °C. IR (KBr) cm−1: 2945, 1735, 1723. 1H NMR (300 MHz, CDCl3) δ: 0.9 (3H, s, H-18), 1.0 (3H, s, H-19), 4.6 (1H, m, 3α-H), 5.4 (1H, dd, J1 = 4 Hz and J2 = 2 Hz, H-6). 13C NMR (100 MHz, CDCl3) δ: 19.3 (C-18), 20.3 (C-19), 25.7 (2C-cyclopentyl), 30.1 (2C-cyclopentyl), 44.1 (C-cyclopentyl), 73.2 (C-3), 121.7 (C-6), 140.3 (C-5), 176.1 (ester carbonyl), 221.0 (C-17). HRMS calcd for C25H36O3 384.2664 found 384.2695.
17-Oxaandrost-5-ene-3β-yl-cyclohexanoate (2d): Yield: 90%. m.p. 157–158 °C. IR (KBr) cm−1: 2928, 1736, 1725. 1H NMR (300 MHz, CDCl3) δ: 0.9 (3H, s, H-18), 1.0 (3H, s, H-19), 4.6 (1H, m, 3α-H), 5.3 (1H, dd, J1 = 4 Hz and J2 = 2 Hz, H-6). 13C NMR (100 MHz, CDCl3) δ: 19.3 (C-18), 20.3 (C-19), 25.3 (2C-cyclohexyl), 25.8 (C-cyclohexyl), 28.6 (2C-cyclohexyl), 43.1 (C-cyclohexyl), 73.4 (C-3), 121.7 (C-6), 139.91 (C-5), 175.8 (ester carbonyl), 220.8 (C-17). HRMS cal for C26H38O3 398.2821 found 398.2885.
17-Oxaandrost-5-ene-3β-yl-cycloheptanoate (2e): Yield: 88%. m.p. 160–162 °C. IR (KBr) cm−1: 2923, 1737, 1725. 1H NMR (300 MHz, CDCl3) δ: 0.9 (3H, s, H-18), 1.0 (3H, s, H-19), 4.6 (1H, m, 3α-H), 5.4 (1H, dd, J1 = 4 Hz and J2 = 2 Hz, H-6). 13C NMR (100 MHz, CDCl3) δ: 19.3 (C-18), 20.3 (C-19), 26.2 (2C-cycloheptyl), 28.5 (2C-cycloheptyl), 30.4 (2C-cycloheptyl), 45.1 (C-cycloheptyl), 73.2 (C-3), 121.7 (C-6), 139.9 (C-5), 176.6 (ester carbonyl), 221.4 (C-17). HRMS cal for C27H40O3 412.2977 found 412.2945.
17-Oxaandrost-5-ene-3β-yl-p-fluorobenzoate (2f): This compound was prepared from dehydroepiandrosterone (1) as described previouslyCitation11.
Synthesis of 17-chloro-16-formyl-androsta-5,16-dien-3β-yl derivatives (3a–3e and 6)
Dimethylformamide (43 mmol) was added dropwise to a round-bottomed flask fitted with a magnetic stirrer and containing cold phosphorus oxychloride (36 mmol), followed by a solution of the corresponding ester (1 mmol) in chloroform (5 mL). The mixture was refluxed for 5 h under N2 atmosphere. The reaction was quenched with a saturated solution of sodium bicarbonate (100 mL). The compound was extracted with chloroform, and the organic phase was washed with water and dried over anhydrous sodium sulfate. The desired product was purified on silica gel column chromatography, using hexane:ethyl actete (80:20) eluent.
17-Chloro-16-formyl-androsta-5,16-dien-3β-yl-cyclopropanoate (3a): Yield: 68%, m.p. 114–115 °C. UV (nm): 257 (ɛ = 10 200). IR (KBr) cm−1: 2928, 1717, 1674, 1589. 1H NMR (300 MHz, CDCl3) δ: 0.9 (3H, s, H-18), 1.0 (3H, s, H-19), 4.6 (1H, m, H-3), 5.3 (1H, dd, J1 = 4 Hz and J2 = 2 Hz, H-6), 9.9 (1H, s, formyl). 13C NMR (100 MHz, CDCl3) δ: 8.3 (2C-cyclopropyl), 13.1 (C-cyclopropyl), 19.2 (C-18), 20.3 (C-19), 73.5 (C-3), 121.7 (C-6), 136.4 (C-16), 140.1 (C-5), 162.2 (C-17), 174.2 (ester carbonyl), 188.0 (formyl). HRMS calcd for C24H31ClO3 402.1962 found 402.1922.
17-Chloro-16-formyl-androsta-5,16-dien-3β-yl-cyclobutanoate (3b): Yield: 69%, m.p. 154–155 °C. UV (nm): 256 (ɛ = 10 100). IR (KBr) cm−1: 2941, 1719, 1671, 1586. 1H NMR (300 MHz, CDCl3) δ: 0.9 (3H, s, H-18), 1.1 (3H, s, H-19), 4.6 (1H, m, 3α-H), 5.4 (1H, dd, J1 = 4 Hz and J2 = 2 Hz, H-6), 9.9 (1H, s, formyl). 13C NMR (100 MHz, CDCl3) δ: 14.6 (C-cyclobutyl), 18.2 (C-cyclobutyl), 19.1 (C-18), 20.2 (C-19), 25.1 (2C-cyclobutyl), 73.2 (C-3), 121.6 (C-6), 136.3 (C-16), 139.9 (C-5), 162.2 (C-17), 174.8 (ester carbonyl), 187.9 (formyl). HRMS cal for C25H33ClO3 416.2118 found 416.2189.
17-Chloro-16-formyl-androsta-5,16-dien-3β-yl-cyclopentanoate (3c): Yield: 72%, m.p. 168–170 °C. UV (nm): 258 (ɛ = 10 300). IR (KBr) cm−1: 2927, 1728, 1708, 1667, 1590. 1H NMR (300 MHz, CDCl3) δ: 0.9 (3H, s, H-18), 1.1 (3H, s, H-19), 4.6 (1H, m, 3α-H), 5.4 (1H, dd, J1 = 4 Hz and J2 = 2 Hz, H-6), 9.9 (1H, s, formyl). 13C NMR (100 MHz, CDCl3) δ: 19.2 (C-18), 20.3 (C-19), 25.8 (2C-cyclopentyl), 30.0 (2C-cyclopentyl), 44.0 (C-cyclopentyl), 73.2 (C-3), 121.6 (C-6), 136.4 (C-16), 140.1 (C-5), 162.1 (C-17), 176.2 (ester carbonyl), 186.0 (formyl). HRMS cal for C26H35ClO3 430.2275 found 430.2210.
17-Chloro-16-formyl-androsta-5,16-dien-3β-yl-cyclohexanoate (3d): Yield: 71%, m.p. 171–173 °C. UV (nm): 257 (ɛ = 10 100). IR (KBr) cm−1: 2929, 1727, 1668, 1592. 1H NMR (300 MHz, CDCl3) δ: 0.9 (3H, s, H-18), 1.1 (3H, s, H-19), 4.6 (1H, m, 3α-H), 5.4 (1H, dd, J1 = 4 Hz and J2 = 2 Hz, H-6), 9.9 (1H, s, formyl). 13C NMR (100 MHz, CDCl3) δ: 19.2 (C-18), 20.3 (C-19), 25.4 (2C-cyclohexyl), 25.8 (C-cyclohexyl), 28.5 (2C-cyclohexyl), 43.4 (C-cyclohexyl), 72.9 (C-3), 121.6 (C-6), 136.4 (C-16), 140.1 (C-5), 162.1 (C-17), 175.5 (ester carbonyl), 188.0 (formyl). HRMS cal for C27H37ClO3 444.2431 found 444.2487.
17-Chloro-16-formyl-androsta-5,16-dien-3β-yl-cycloheptanoate (3e): Yield: 69%, m.p. 168–170 °C. UV (nm): 257 (ɛ = 10 100). IR (KBr) cm−1: 2946, 1719, 1671, 1585. 1H NMR (300 MHz, CDCl3) δ: 0.9 (3H, s, H-18), 1.0 (3H, s, H-19), 4.5 (1H, m, 3α-H), 5.4 (1H, dd, J1 = 4 Hz and J2 = 2 Hz, H-6), 9.9 (1H, s, formyl). 13C NMR (100 MHz, CDCl3) δ: 19.2 (C-18), 20.3 (C-19), 26.3 (2C-cycloheptyl), 28.4 (2C-cycloheptyl), 30.4 (2C-cycloheptyl), 45.1 (C-cycloheptyl), 73.1 (C-3), 121.6 (C-6), 136.4 (C-16), 140.1 (C-5), 162.2 (C-17), 176.5 (ester carbonyl), 188.0 (formyl). HRMS cal for C28H39ClO3 458.2588 found 458.2514.
17-Chloro-16-formyl-androsta-5,16-diene-3β-yl-p-fluorobenzoate (3f): This compound was prepared from 17-oxaandrost-5-ene-3β-yl-p-fluorobenzoate (2f) as described previouslyCitation11.
17-Chloro-16-formyl-androsta-5,16-dien-3β-yl-acetate (6): Yield: 82%, m.p. 165–167 °C. UV (nm): 257 (ɛ = 10 200). IR (KBr) cm−1: 2928, 1717, 1674, 1589. 1H NMR (300 MHz, CDCl3) δ: 0.9 (3H, s, H-18), 1.0 (3H, s, H-19), 2.1 (3H, s, 3β-OCOCH3), 4.6 (1H, m, 3α-H), 5.3 (1H, dd, J1 = 4 Hz and J2 = 2 Hz, H-6), 9.9 (1H, s, formyl). 13C NMR (100 MHz, CDCl3) δ: 19.2 (C-18), 20.3 (C-19), 21.1 (3β-OCOCH3), 73.5 (C-3), 121.7 (C-6), 136.4 (C-16), 140.1 (C-5), 162.2 (C-17), 174.2 (ester carbonyl), 188.0 (formyl). HRMS cal for C22H31ClO3 379.1962 found 379.1954.
The synthesis of compound 6 as well as its spectroscopy data have been reported previouslyCitation17.
Synthesis of 16-formyl-17-pyrazole-androsta-5,16-diene-3β-yl derivatives (4a–4e)
Pyrazole (0.6 mmol) was added to a mixture of Cs2CO3 (1 mmol) and the corresponding 17-chloro-16-formyl-androsta-5,16-dien-3β-yl derivative (0.5 mmol) in dry DMF (2 mL). The mixture was heated at 60 °C under N2 atmosphere for 3.5 h. Cold water was added and the solid was filtered. The desired pyrazole derivative was purified by column chromatography on Florisil, using hexane:ethyl acetate (70:30) eluent.
16-Formyl-17-(1H-pyrazole-1-yl)-androsta-5,16-dien-3β-yl-cyclopropanoate (4a): Yield: 57%. m.p.: 212–214 °C. UV (nm): 296 (ɛ = 14 600). IR cm−1: 2915, 1725, 1650, 1597. 1H NMR (300 MHz, CDCl3) δ: 1.1 (3H, s, H-18), 1.2 (3H, s, H-19), 4.6 (1H, m, 3α-H), 5.4 (1H, dd, J1 = 4 Hz and J2 = 2 Hz, H-6), 6.5 (1H, m, aromatic), 7.7 (1H, d, J = 1.6 Hz, aromatic), 7.8 (1H, d, J = 1.6 Hz, aromatic), 10.1 (formyl). 13C NMR (100 MHz, CDCl3) δ: 8.3 (2C-cyclopropyl), 13.0 (C-cyclopropyl), 19.2 (C-18), 20.5 (C-19), 73.5 (C-3), 107.9 (aromatic), 121.7 (C-6), 129.5 (C-16), 130.5 (aromatic), 139.5 (C-5), 142.4 (aromatic), 160.9 (C-17), 174.2 (ester carbonyl), 190.4 (formyl). HRMS calcd for C27H34N2O3 435.5705 found 435.5699.
16-Formyl-17-(1H-pyrazole-1-yl)-androsta-5,16-dien-3β-yl-cyclobutanoate (4b): Yield: 57%. m.p.: 199–201 °C. UV (nm): 294 (ɛ = 14 500). IR cm−1: 2930, 1722, 1646, 1597. 1H NMR (300 MHz, CDCl3) δ: 1.1 (3H, s, H-18), 1.2 (3H, s, H-19), 4.6 (1H, m, 3α-H), 5.4 (1H, d, J1 = 5.1 Hz, H-6), 6.5 (1H, m, aromatic), 7.7 (1H, d, J = 2.3 Hz, aromatic), 7.8 (1H, d, J = 1.6 Hz, aromatic), 10.1 (formyl). 13C NMR (100 MHz, CDCl3) δ: 14.6 (C-cyclobutyl), 18.2 (C-cyclobutyl), 19.2 (C-18), 20.3 (C-19), 25.1 (2C-cyclobutyl), 73.3 (C-3), 107.9 (aromatic), 121.9, 129.5 (C-16), 130.5 (aromatic), 140.0 (C-5), 142.5 (aromatic), 161.0 (C-17), 174.9 (ester carbonyl), 190.4 (formyl). HRMS calcd for C28H36N2O3 449.5970 found 449.5868.
16-Formyl-17-(1H-pyrazole-1-yl)-androsta-5,16-dien-3β-yl-cyclopentanoate (4c): Yield: 63%. m.p.: 258–260 °C. UV (nm): 297 (ɛ = 14 700). IR cm−1: 2914, 1723, 1653, 1597. 1H NMR (300 MHz, CDCl3) δ: 1.1 (3H, s, H-18), 1.2 (3H, s, H-19), 4.6 (1H, m, 3α-H), 5.4 (1H, d, J = 4.7 Hz), 6.5 (1H, m, aromatic), 7.7 (1H, d, J = 2.3 Hz, aromatic), 7.8 (1H, d, J = 1.6 Hz, aromatic), 10.1 (formyl). 13C NMR (100 MHz, CDCl3) δ: 19.2 (C-18), 20.3 (C-19), 25.8 (2C-cyclopentyl), 30.1 (2C-cyclopentyl), 44.1 (C-cyclopentyl), 73.3 (C-3), 107.9 (aromatic), 121.9 (C-6), 129.5 (C-16), 130.5 (aromatic), 140.0 (C-5), 142.5 (aromatic), 161.0 (C-17), 174.9 (ester carbonyl), 190.4 (formyl). HRMS calcd for C29H38N2O3 463.6236 found 463.6235.
16-Formyl-17-(1H-pyrazole-1-yl)-androsta-5,16-dien-3β-yl-cyclohexanoate (4d): Yield: 64%. m.p.: 257–259 °C. UV (nm): 297 (ɛ = 14 600). IR cm−1: 2937, 1723, 1650, 1596. 1H NMR (300 MHz, CDCl3) δ: 1.1 (3H, s, H-18), 1.2 (3H, s, H-19), 4.6 (1H, m, 3α-H), 5.4 (1H, dd, J = 5.1 Hz, H-6),), 6.5 (1H, m, aromatic), 7.7 (1H, d, J = 2.3 Hz, aromatic), 7.8 (1H, d, J = 1.6 Hz, aromatic), 10.1 (formyl). 13C NMR (100 MHz, CDCl3) δ: 19.2 (C-18), 20.5 (C-19), 25.3 (2C-cyclohexyl), 25.6 (C-cyclohexyl), 28.5 (2C-cyclohexyl), 43.4 (C-cyclohexyl), 73.1 (C-3), 107.9 (aromatic), 121.8 (C-6), 129.5 (C-16), 130.6 (aromatic), 140.0 (C-5), 142.5 (aromatic), 161.0 (C-17), 175.5 (ester carbonyl), 190.4 (formyl). HRMS calcd for C30H40N2O3 477.6502 found 477.6501.
16-Formyl-17-(1H-pyrazole-1-yl)-androsta-5,16-dien-3β-yl-cycloheptanoate (4e): Yield: 59%. m.p.: 225–228 °C. UV (nm): 296 (ɛ = 14500). IR cm−1: 2934, 1726, 1650, 1596. 1H NMR (300 MHz, CDCl3) δ: 1.1 (3H, s, H-18), 1.2 (3H, s, H-19), 4.6 (1H, m, 3α-H), 5.4 (1H, dd, J = 5.1 Hz, H-6), 6.5 (1H, m, aromatic), 7.7 (1H, d, J = 2.3 Hz, aromatic), 7.8 (1H, d, J = 1.6 Hz, aromatic), 10.1 (formyl). 13C NMR (100 MHz, CDCl3) δ: 19.2 (C-18), 20.5 (C-19), 26.2 (2C-cycloheptyl), 28.3 (2C-cycloheptyl), 30.4 (2C-cycloheptyl), 45.1 (C-cycloheptyl), 73.1 (C-3), 107.9 (aromatic), 121.8 (C-6), 129.5 (C-16), 130.6 (aromatic), 140.0 (C-5), 142.5 (aromatic), 161.0 (C-17), 175.5 (ester carbonyl), 190.4 (formyl). HRMS calcd for C31H42N2O3 491.6359 found 491.6350.
16-Formyl-17-(1H-pyrazole-1-yl)androsta-5,16-diene-3β-yl-p-fluorobenzoate (4f): This compound was prepared from 17-chloro-16-formyl-androsta-5,16-diene-3β-yl-p-fluorobenzoate (3f) as described previouslyCitation11.
Synthesis of 16-formyl-17-(1H-1,3-imidazole-1-yl)androsta-5,16-dien-3β-yl-acetate (7)
A mixture of imidazole (3 mmol), K2CO3 (6 mmol) and the steroidal derivative 6 (1 mmol) in dry DMF (5 mL) was heated at 50 °C under N2 atmosphere for 3 h. Cold water was added and the precipitate was filtered. The compound was purified by column chromatography on Florisil, using hexane:ethyl acetate (70:30) eluent. Yield: 93%. m.p.: 225–227 °C. UV (nm): 257 (ɛ = 14 400). IR cm−1: 2947, 1731, 1659, 1616, 1234. 1H NMR (300 MHz, CDCl3) δ: 1.0 (3H, s, H-18), 1.1 (3H, s, H-19), 2.1 (3H, s, 3β-OCOCH3), 4.6 (1H, m, 3α-H), 5.4 (1H, dd, J1 = 4 Hz and J2 = 2 Hz, H-6), 7.1 (1H, s, aromatic), 7.2 (1H, s, aromatic), 7.6 (1H, s, aromatic) 9.7 (formyl). 13C NMR (100 MHz, CDCl3) δ: 15.8 (C-18), 19.0 (C-19), 21.0 (3β-OCOCH3), 73.4 (C-3), 119.7 (aromatic), 121.5 (C-6), 130.6 (C-16), 133.7 (aromatic), 137.6 (aromatic), 139.8 (C-5), 160.4 (C-17), 170.2 (ester carbonyl), 187.2 (formyl). HRMS cal for C25H32N2O3 409.2413 found 409.2476.
Biological activity
Human, animal and tissue procedures
The prostate of a 53-year-old man who died from renal insufficiency was extirpated 4 h after death in the Pathology Department of the General Hospital of Mexico City. This protocol was approved by the Ethical Committee of the Mexico City General Hospital.
The tissue was chilled in ice-cold 150 mM NaCl and stored at −20 °C. The frozen human prostate was thawed on ice, rinsed and minced with an IKA A11 basic tissue mill (Wilmington, NC). Unless otherwise specified, the following procedures were carried out at 4 °C. The human prostate tissue was homogenized in two volumes of buffer A (20 mM sodium phosphate), pH 6.5 containing 0.32 M sucrose, 0.1 mM dithiothreitol (Sigma-Aldrich, Inc.) with an Ultra-Turrax IKA, T18 basic tissue homogenizer (Wilmington, NC). The homogenates were centrifuged at 1500g for 60 min at 0 °CCitation18 in a SW 60 Ti rotor (Beckman Instruments, Palo Alto, CA).
The pellets were separated, suspended in buffer A and kept at −70 °C. The suspension, 1.35 mg of protein/mL for human prostates, determined by the Bradford methodCitation19, was used as a source of 17β-HSD5.
Determination of 17β-HSD5 activity
The activity of 17β-HSD5 using labeled plus unlabeled Δ4-androstenedione (4-dione) as the substrate was determined as reported previouslyCitation18.
In order to determine the optimum conditions (kinetic parameters) for the measurement of human prostate membrane fraction activity as a source of 17β-HSD5, different pHs (6.5, 7, 7.5 and 8) and concentrations (43, 87, 174 and 313 μM) of unlabeled 4-dione plus 4 nM of labeled 4-dione were used. Different temperatures (36, 36.5, 37, 37.5 °C) and human prostate membrane protein (60, 180, 600 and 800 μg), determined by the Bradford MethodCitation19, were used. Different NADPH concentrations (0.5, 2.5, 5.0 and 10 mM) were also evaluated. Each experiment was repeated twice in duplicate. The reaction in each sample began when human prostate membrane was added to the incubating medium. As a control, this procedure was also carried out without the addition of the enzyme fraction.
The incubating samples of each of these assays and experiments were extracted with ethyl acetate and the solvent from the extractions of each sample were combined in a tube and dried. The extract was dissolved in 50 μL of chloroform:methanol (1:1) and spotted on a thin-layer chromatography plate, HPTLC Keiselgel 60 F254 (Merck, Mexico City). Standards of 4-dione, T, were applied on two lanes, one on each side of the plate. These plates were eluted twice in a mixture of chloroform:acetone (9:1)Citation16 and air dried; the radioactivity on them was scanned using an AR2000 Bioscanner (Bioscan, Washington DC). Standards were developed by fluorescence (UV lamp; λ = 254 nm from UVP, Upland, CA) and the data obtained were plotted using SigmaPlot 12 software (Systat Software, Inc., San Jose, CA).
The radioactive zones that showed identical chromatographic behavior to that of the T standard (retention factor, Rf) on the plate were separated in tubes and soaked in 0.2 mL of acetone for 30 min. Each of these fractions was then removed from the tubes and 10 mg of the T standard was added and solubilized in this solvent. A recrystallization of T was carried out; the crystals formed were separated from the acetone (mother liquor). Crystals and mother liquors of each sample were dried under nitrogen steam and the radioactivity contained in 1 mg of each one was determined in a Packard Scintillation Counter (Model 2100 TR) from Perkin Elmer (Boston, MA). This recrystallization procedure was repeated three more times in order to obtain a constant specific activity between the crystals and mother liquors.
Determination of kinetic parameters of 17β-HSD5
In order to determine the kinetic parameters, optimum conditions of pH, temperature, protein and NADPH were used. The following parameters were used: pH 7.5, temperature 37.5 °C, 600 μg human prostate membrane protein and 2.5 mM of NADPH. The incubating time was 30 min.
The concentrations of non-labeled 4-dione used for this experiment were the following: 187, 303, 417, 512, 735 μM plus labeled 4-dione, 4 nM. The incubation procedure was the same as reported previouslyCitation18. The reaction in each sample began when human prostate membrane was added to the incubating medium. As a control, this procedure was also carried out without the addition of the enzyme fraction.
Extraction, purification, radioactivity determination and recrystallization procedures were the same as described in Section “Determination of 17β-HSD5 activity”. The T transformation yields were calculated from the lanes, taking into account the entire radioactivity in the HPTLC, and were plotted using SigmaPlot 12 software. For the control extraction, chromatographic separations and identifications were carried out in the same manner.
Biological activity of steroidal derivatives on 17β-HSD5 activity
To calculate the IC50 values of steroids 4a–4f and 7 required to inhibit 50% of 17β-HSD5 enzyme activity, six series of tubes containing increasing concentrations of these steroids (10−11–10−3 M) were incubated in duplicate, in the presence of 1 mM of dithiothreitol, 40 mM sodium phosphate buffer of pH 7.5; 2.5 mM NADPH, 715.2 µM of unlabeled 4-dione plus [3H] 4-dione, 4 nM and 600 µg of protein from the enzymatic fraction obtained from human prostate as described in Section “Human, animal and tissue procedures”. The reaction was incubated at 37.5 °C for 30 min, using a final buffer volume of 0.1 mL and the procedures for extraction, purification and identification were carried out as described in Section “Determination of 17β-HSD5 activity”. The results were plotted and the IC50 values were calculated from these plots using SigmaPlot 12 Software.
In vivo activity of 4a–4f
Gonadectomized male hamsters
All procedures involving animals were approved by the Institutional Care and Use Committee of the Metropolitan University of Mexico (UAM; Xochimilco, Mexico). All animals used in this study were obtained from the Animal Care Facility at UAM.
For the experiments in vivo, 152 adult male golden hamsters (2.5 months old; 150–200 g) were gonadectomized using isoflurane as anesthetic. The castrated hamsters were allowed to recover for 30 days prior to experimentation. Hamsters were housed in a room with controlled temperature (22 °C) and light–dark periods of 12 h. From surgery until sacrifice, the hamsters were fed with food and water ad libitum.
After 30 days following the gonadectomy in these conditions, the hamsters were separated into 19 groups of four animals per group. The hamsters were treated for six days as detailed below and then sacrificed with CO2. This experiment was carried out twice under the same conditionsCitation20.
Pharmacological activity of steroidal derivatives
For six consecutive days, each of the steroid derivatives 4a–4f and 7 (2 mg/kg body weight (BW)) dissolved in 200 µL sesame oil, together with 1 mg T or 4-dione 1 mg/kg (BW), was administered by subcutaneous (s.c.) injection to a group of gonadectomized hamsters (four animals per derivative). Four groups of gonadectomized animals were used as controls: for six consecutive days, the first group was injected s.c. with 200 µL sesame oil; the second group, with 1 mg T/kg (BW); the third group, with 1 mg 4-dione/kg (BW) and the fourth group with 1 mg T plus 1 mg finasteride/kg (BW). After treatment, the animals were sacrificed with CO2. The diameters of the flank organs were measured, and the prostate and seminal vesicles of each hamster were dissected and weighed. Two separate experiments were performed for each group of steroid-treated hamsters. The results were analyzed using one-way analysis of variance and Dunnett’s method to compare means, using the JMP IN 5.1 program.
Results
Kinetic parameters of 17β-HSD5
The pH-dependent conversion of labeled androstenedione to testosterone
The conversion of [3H] androstendione into T in the presence of 2 mM of NADPH by the membrane fractions (600 μg) of human prostate is shown in panel A, . The optimum mean activity of 17β-HSD5 was obtained at 174 µM of 4-dione and pH of 7.5. , panel B shows the results of the successive recrystallization of T isolated after the chromatographic procedure.
Figure 3. Panel A: Human prostatic 17β-hydroxysteroid dehydrogenase type 5 (17β-HSD5) activity in the presence of 2 mM NADPH, determined at different pH values. These data show the effect of labeled plus unlabeled concentrations of 4-dione (43, 87, 174, 313 μM) on the formation of labeled T. Panel B: Radiochemical purity of T isolated after HPTLC procedure, and determined by repetitive recrystallizations in the presence of the T standard. Cr, crystallization; ML, mother liquors.
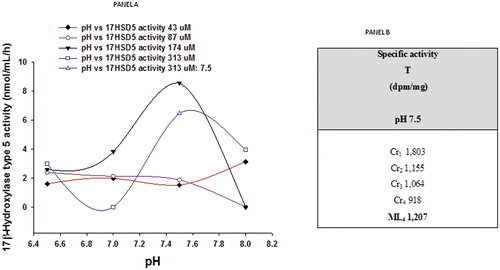
Conversion of 4-dione into T in the presence of NADPH at different temperatures
, panel A shows that the optimum mean concentration of NADPH for the conversion of labeled 4-dione into T was 2.5 mM. Panel B shows that 37.5 °C was the temperature under which the highest conversion of 4-dione to T was obtained.
Figure 4. Panel A shows the effect of different concentrations of NADPH on the conversion of labeled plus unlabeled 4-dione (174 μM) to T. Panel B shows the effect of different temperatures (°C) on this conversion in the presence of 2.5 mM of NADPH.
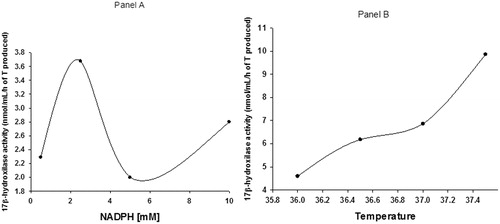
The kinetic parameters were determined from a Lineweaver–Burk plotCitation21 and are shown in . The results from this study exhibited a Km value of 715.2 µM and Vmax of 19.39 nmol/µL ().
Figure 5. Kinetic of 17β-HSD5 enzyme. Lineweaver–Burk plot represents the conversion of labeled plus unlabeled 4-dione (x axis) to T (y axis). Different concentrations of labeled plus unlabeled 4-dione were incubated with 2.5 mM of NADPH at pH 7.5 and 37.5 °C in the presence of 600 μg protein obtained from the membrane fraction of human prostate.
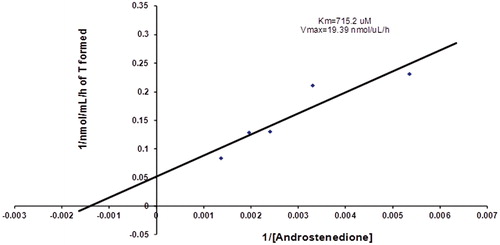
Biological effect of the steroidal derivatives on the inhibition of human 17β-HSD5
The effect of compounds 4a–4f and 7 on the activity of 17-HSD5 is shown in . All synthesized compounds inhibited the 17β-HSD5 enzyme. Compound 4a exhibited the highest potency (IC50 = 0.15 ± 0.02 nM) followed by 4c (IC50 = 0.5 ± 0.09 nM), 7 (IC50 = 0.66 ± 0.32 nM), 4f (IC50 = 0.79 ± 0.003 nM), 4b (IC50 = 3.2 ± 0.9 nM), 4f (0.79 ± 0.003 nM), 4d (IC50 = 90 ± 0.02 nM) and 4e (IC50 17β-HSD5 = 95 ± 3 nM).
Figure 6. Effect of the novel steroids on the activity of 17β-HSD5 enzyme IC50 values show the concentration, for each compound, that inhibited the activity of 17β-HSD5 by 50%.
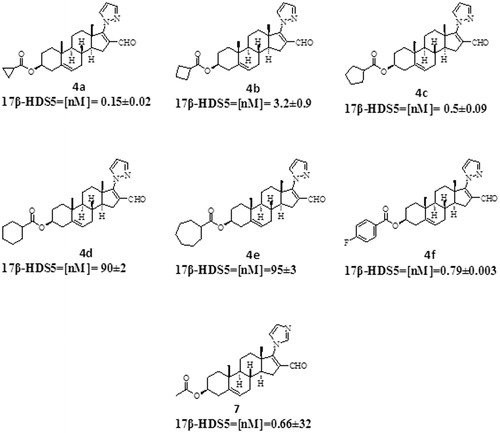
Weight of the prostate gland and seminal vesicles
After gonadectomy, the weight of the hamster prostate and seminal vesicle decreased (p < 0.05) in contrast to the normal weight. Daily injections with vehicle alone (control C) did not have this effect, but when 1 mg/kg of T or 4-dione was administered for 6 days, the weight of these glands in gonadectomized male hamsters was significantly stimulated (p < 0.05) (). When T (1 mg/kg) and finasteride (1 mg/kg) or compounds 4a–4f and 7 (2 mg/kg) were injected together, the weight of the prostate and seminal vesicles diminished significantly (p < 0.05) in contrast to the T-treated hamsters (, Panel A). Surprisingly, compound 4e did not show any significant effect on the weight of prostate and seminal vesicles (, Panel A).
Figure 7. Weight of prostate and seminal vesicles glands ± standard deviation from castrated hamsters receiving different subcutaneous treatments for 6 days. The control hamsters were treated with vehicle (sesame oil) only. The pharmacological experiment was carried out in duplicate. The asterisk shows the statistically significant difference between the group of hamsters treated with T (Panel A) or 4-dione (Panel B) and those treated with finasteride (F) or the synthesized steroids (4a–4f and 7). C – control, T – testosterone, F – finasteride, 4-dione – Δ4-androstenedione.
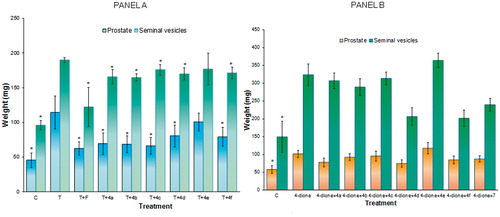
The weight of prostate and seminal vesicles from hamsters treated with 4-dione was greater than those of the gonadectomized hamsters treated with sesame oil (C in , Panel B). With the exception of steroid 4e, derivatives 4a–4f slightly decreased the weight of prostate and seminal vesicles from hamsters treated with 4-dione (, Panel B). However, treatment with 4c did not decrease the weight of seminal vesicles in those hamsters (, Panel B). Further studies are required to explain this phenomenon.
Figure 8. Diameter size ± standard deviations of the pigmented spot from gonadectomized hamster flank organs receiving different treatments with T (Panel A) or 4-dione (Panel B) plus the novel steroids for 6 days. Control gonadectomized hamsters were treated with vehicle (ethanol) only. The asterisk shows the statistically significant difference between the group of hamsters treated with T (Panel A) or 4-dione (Panel B) and those treated with finasteride (F) or the synthesized steroids (4a–4f and 7). C, control, T, testosterone, F, finasteride, 4-dione, Δ4-androstenedione.
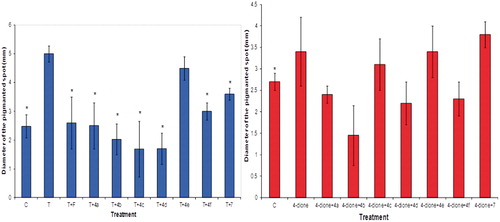
Flank organ test
After castration, the diameter of the male hamster flank organs decreased (p < 0.005) compared to that of the intact animals. Treatment with vehicle alone did not change this, but subcutaneous injections of 1 mg/kg of T for 6 days significantly increased (p < 0.005) the diameter of the pigmented spot in castrated male hamsters’ flank organs (, Panel A). With the exception of compound 4e, steroids 4a–4f and 7 (2 mg/kg) injected together with T (1 mg/kg) significantly decreased (p < 0.005) the diameter of the pigmented spot in contrast to the hamsters treated with T (, Panel A).
The diameter of the pigmented spot of flank organs increased slightly under treatment with 4-dione as compared to the control (, Panel B); T-treatment showed more efficacy than 4-dione by producing this effect (, Panel A). Steroids 4a, 4b, 4d and 4f decreased the size of the flank organs as compared to 4-dione ().
Discussion
The conversion of [3H] 4-dione to T in the presence of NADPH by the membrane fractions of human prostate as well as the radiochemical purity of T demonstrated the presence of 17β-HSD5 enzyme in this fraction. The presence of 17β-HSD5 in prostate has been previously demonstratedCitation22,Citation23. In addition, the expression of 17β-HSD3 activity in normal cells as well as in prostate cancer has also been describedCitation24. This type 3 isozyme is expressed more in seminal vesicles than in prostate; and it is increased in prostate cancer as compared to the normal glandCitation24,Citation25.
Kinetic data indicated that 17β-HSD5 enzyme obtained from the membrane fraction of human prostate clearly followed the Michaelis–Menten kinetic under these experimental conditions (pH 7.5); therefore this fraction can be used as a source of 17β-HSD5 activity to identify novel inhibitors of this enzymeCitation21,Citation26.
Steroids 4a–4f and 7 showed in vitro activity as inhibitors of the 17β-HSD5 enzyme present in the human prostate. With the exception of 4e, these steroids also exhibited pharmacological activity. The overall results suggested compound 4e was not hydrolyzed by the specific esterase present in the membrane fraction of prostate homogenatesCitation27. This esterase has been previously identified as an oxidized protein hydrolase (OPH), which is overexpressed in the tumorigenic LNCaP cell line. OPH is a serine esterase/protease that has a well-characterized esterase activity towards α-naphthyl butyrate and an exopeptidase activity for removing the N-terminally acetylated amino acid residues from peptides/proteinsCitation27.
In addition, pharmacological results suggest that steroid 4e could be metabolized by the hepatic or circulatory esterasesCitation28; as a consequence, an absence of pharmacological effect was observed for this steroid.
Therefore, the incorporation of a pyrazole or imidazole group at C-17 on the dehydroepiandrosterone skeleton having an ester moiety at C-3 (4a–4d and 4f) seems to be the reason for the increase of the ability of these steroids to inhibit the 17β-HSD5 enzyme, since this ester moiety increased the lipophilicity of this molecule and brought on an in vitro inhibitory activity of this enzyme.
The overall results indicated that the incorporation of a pyrazole ring or its isomer imidazole at C-17 of the dehydroepiandrosterone skeleton enhances the inhibition of the 17β-HSD5 enzyme in the in vitro assay using human prostate membrane fraction as a source. Furthermore, compounds 4a–4f and 7 also showed in vivo effect.
The reason for using specific inhibitors of 17β-HSD5 instead of inhibitors of cytochrome P450 in cancer therapy is to decrease side effects. Androgen deprivation therapy using inhibitors of cytochrome P450 as a treatment for prostate cancer could improve existing therapies for this illness. However, these anti-hormonal agents induce a decrease in the production of cortisol by the adrenals and an increase of the synthesis of mineralocorticoids, thus inducing strong metabolic and immunological disturbancesCitation29–31.
Currently, arbiterone is the drug of choice for use in combination with prednisone for the treatment of metastatic castration-resistant prostate cancerCitation29. This drug was approved by the US Food and Drug Administration (FDA) in April 2011 for this condition. Arbiterone is an inhibitor of 17 α-hydroxylase/C17,20 lyase (CYP17A1)Citation29,Citation31.
Nevertheless using inhibitors of 17β-HSD5 might offer some advantage for the therapy of prostatic cancer compared with arbiterone, since 17β-HSD5 does not play a part in the synthesis of mineralocorticoids or glucocoticoids. Therefore therapy using 17β-HSD5 inhibitors does not require the additional use of prednisone.
The advantage of using cell cultures expressing pure enzymes instead of homogenates of different tissues for metabolic studies as a methodology to test inhibitors of 5α-Rs and 17β-HSD5s has been an idea on which several research groups have focused. It is true that a clean preparation produces the expected results. However, it has been demonstrated that artificial conditions tend to induce false results; this is true, for example, when different plates of culture cells (plastic or matricell) are used for the expression of different enzymes by the culture cellsCitation32. Other substances such as growth factor or enriched culture medium are not normally added to prostate homogenatesCitation31. We preferred to use conditions similar to those in the in vivo model. This allowed us to better understand the pharmacokinetic behavior of the novel compounds, which is usually different from that observed in in vitro studies.
Supplemental Material.pdf
Download PDF (1.3 MB)Declaration of interest
This research did not have any real or perceived conflicts of interest or disclosure arising from intellectual, personal or financial circumstances of the researchers.
We would like to thank CONACYT project No 165049 and DGAPA project IN 211312 (UNAM) for their generous financial support.
References
- Chang K-H, Li R, Papari-Zareei M, et al. Dihydrotestosterone synthesis bypasses testosterone to drive castration-resistant prostate cancer. Proc Nat Acad Sci 2011;108:13728–33
- Harris WP, Mostaghel EA, Nelson PS, Montgomery B. Androgen deprivation therapy: progress in understanding mechanisms of resistance and optimizing androgen depletion. Nat Clin Pract Urol 2009;6:76–85
- Zong Y, Goldstein AS. Adaptation or selection—mechanisms of castration-resistant prostate cancer. Nat Rev Urol 2013;10:90–8
- Karantanos T, Corn PG, Thompson TC. Prostate cancer progression after androgen deprivation therapy: mechanisms of castrate resistance and novel therapeutic approaches. Oncogene 2013;32:5501–11
- Kikuchi A, Furutani T, Azami H, et al. In vitro and in vivo characterisation of ASP9521: a novel, selective, orally bioavailable inhibitor of 17β-hydroxysteroid dehydrogenase type 5 (17βHSD5; AKR1C3). Invest New Drugs 2014;32:860–70
- Labrie F. Blockade of testicular and adrenal androgens in prostate cancer treatment. Nat Rev Urol 2011;8:73–85
- Qiu W, Zhou M, Mazumdar M, et al. Structure-based inhibitor design for an enzyme that binds different steroids: a potent inhibitor for human type 5 17β-hydroxysteroid dehydrogenase. J Biol Chem 2007;282:8368–79
- Zhou M, Qiu W, Chang HJ, et al. Purification, crystallization and preliminary X-ray diffraction results of human 17β-hydroxysteroid dehydrogenase type 5. Acta Crystallogr Sect D: Biol Crystallogr 2002;58:1048–50
- Nakamura Y, Suzuki T, Nakabayashi M, et al. In situ androgen producing enzymes in human prostate cancer. Endocr-Relat Cancer 2005;12:101–7
- Marchais-Oberwinkler S, Henn C, Möller G, et al. 17β-Hydroxysteroid dehydrogenases (17β-HSDs) as therapeutic targets: protein structures, functions, and recent progress in inhibitor development. J Steroid Biochem Mol Biol 2011;125:66–82
- Garrido M, Cabeza M, Cortés F, et al. Cytotoxic effect of novel dehydroepiandrosterone derivatives on different cancer cell lines. Eur J Med Chem 2013;68:301–11
- Iype PT, Iype LE, Verma M, Kaighn ME. Establishment and characterization of immortalized human cell lines from prostatic carcinoma and benign prostatic hyperplasia. Int J Oncol 1998;12:257–63
- Castagnetta LAM, Carruba G, Traina A, et al. Expression of different 17β-hydroxysteroid dehydrogenase types and their activities in human prostate cancer cells. Endocrinology 1997;138:4876–82
- Sharifi N, Auchus RJ. Steroid biosynthesis and prostate cancer. Steroids 2012;77:719–26
- Dewang PM, Kim D-K. Synthesis and biological evaluation of 2-pyridyl-substituted pyrazoles and imidazoles as transforming growth factor-β type 1 receptor kinase inhibitors. Bioorganic Med Chem Lett 2010;20:4228–32
- Trapani G, Dazzi L, Pisu MG, et al. A rapid method for obtaining finasteride, a 5α-reductase inhibitor, from commercial tablets. Brain Res Protoc 2002;9:130–4
- Njar VCO, Kato K, Nnane IP, et al. Novel 17-azolyl steroids, potent inhibitors of human cytochrome 17α-hydroxylase-C17,20-lyase (P45017α): potential agents for the yreatment of prostate cancer. J Med Chem 1998;41:902–12
- Cabeza M, Trejo KV, González C, et al. Steroidal 5α-reductase inhibitors using 4-androstenedione as substrate. J Enzyme Inhib Med Chem 2011;26:712–19
- Bradford MM. A rapid and sensitive method for the quantitation of microgram quantities of protein utilizing the principle of protein–dye binding. Anal Biochem 1976;72:248–54
- Cabeza M, Heuze Y, Quintana H, Bratoeff E. Comparison between two different hamster models used for the determination of testosterone and finasteride activity. Asian J Anim Vet Adv 2010;5:202–9
- Lineweaver H, Burk D. The determination of enzyme dissociation constants. J Am Chem Soc 1934;56:658–66
- Luu-The V. Assessment of steroidogenesis and steroidogenic enzyme functions. J Steroid Biochem Mol Biol 2013;137:176–82
- Nahoum V, Gangloff A, Legrand P, et al. Structure of the human 3α-hydroxysteroid dehydrogenase type 3 in complex with testosterone and NADP at 1.25-Å resolution. J Biol Chem 2001;276:42091–8
- Koh E, Noda T, Kanaya J, Namiki M. Differential expression of 17β-hydroxysteroid dehydrogenase isozyme genes in prostate cancer and noncancer tissues. Prostate 2002;53:154–9
- Vicker N, Sharland CM, Heaton WB, et al. The design of novel 17β-hydroxysteroid dehydrogenase type 3 inhibitors. Mol Cell Endocrinol 2009;301:259–65
- Johnson KA, Goody RS. The original Michaelis constant: translation of the 1913 Michaelis–Menten paper. Biochemistry 2011;50:8264–9
- McGoldrick C, Jiang Y-L, Paromov V, et al. Identification of oxidized protein hydrolase as a potential prodrug target in prostate cancer. BMC Cancer 2014;14:77
- Aldridge WN. Serum esterases. I. Two types of esterase (A and B) hydrolysing p-nitrophenyl acetate, propionate and butyrate, and a method for their determination. Biochem J 1953;53:110–17
- DeVore NM, Scott EE. Structures of cytochrome P450 17A1 with prostate cancer drugs abiraterone and TOK-001. Nature 2012;482:116–19
- O'Donnell A, Judson I, Dowsett M, et al. Hormonal impact of the 17[alpha]-hydroxylase//C17,20-lyase inhibitor abiraterone acetate (CB7630) in patients with prostate cancer. Br J Cancer 2004;90:2317–25
- Auchus RJ, Yu MK, Nguyen S, Mundle SD. Use of prednisone with abiraterone acetate in metastatic castration-resistant prostate cancer. Oncologist 2014;19:1231–40
- Délos S, Carsol J-L, Fina F, et al. 5α-Reductase and 17β-hydroxysteroid dehydrogenase expression in epithelial cells from hyperplastic and malignant human prostate. Int J Cancer 1998;75:840–6