Abstract
Carbonic anhydrases (CAs, EC 4.2.1.1) belonging to the α-, β-, γ-, δ- and ζ-CAs are ubiquitous metalloenzymes present in prokaryotes and eukaryotes. CAs started to be investigated in detail only recently in pathogenic bacteria, in the search for antibiotics with a novel mechanism of action, since it has been demonstrated that in many such organisms they are essential for the life cycle of the organism. CA inhibition leads to growth impairment or growth defects in several pathogenic bacteria. The microbiota of the human oral mucosa consists of a myriad of bacterial species, Porphyromonas gingivalis being one of them and the major pathogen responsible for the development of chronic periodontitis. The genome of P. gingivalis encodes for a β- and a γ-CAs. Recently, our group purified the recombinant γ-CA (named PgiCA) which was shown to possess a significant catalytic activity for the reaction that converts CO2 to bicarbonate and protons, with a kcat of 4.1 × 105 s−1 and a kcat/Km of 5.4 × 107 M−1 × s−1. We have also investigated its inhibition profile with a range of inorganic anions such as thiocyanate, cyanide, azide, hydrogen sulfide, sulfamate and trithiocarbonate. Here, we describe the cloning, purification and kinetic parameters of the other class of CA identified in the genome of P. gingivalis, the β-CA, named PgiCAb. This enzyme has a good catalytic activity, with a kcat of 2.8 × 105 s−1 and a kcat/Km of 1.5 × 107 M−1 × s−1. PgiCAb was also inhibited by the clinically used sulfonamide acetazolamide, with an inhibition constant of 214 nM. The role of CAs as possible virulence factors of P. gingivalis is poorly understood at the moment but their good catalytic activity and the fact that they might be inhibited by a large number of compounds, which may pave the way for finding inhibitors with antibacterial activity that may elucidate these phenomena and lead to novel antibiotics.
Introduction
The possibility to develop new antibacterial agents raised much interest recently. The main classes of antibiotics clinically used nowadays act towards the inhibition of four classical targets: (a) cell wall biosynthesis; (b) protein biosynthesis; (c) DNA and RNA biosynthesis; (d) folate biosynthesisCitation1,Citation2. Carbonic anhydrases (CAs, EC 4.2.1.1) started to be investigated in detail only recently in pathogenic bacteria, in the search for antibiotics with a novel mechanism of action, since it has been demonstrated that in many bacteria, CAs are essential for the life cycle of the organism and that their inhibition leads to growth impairment or growth defects of the pathogenCitation1–16.
CAs are ubiquitous metalloenzymes, present in prokaryotes and eukaryotes. The five genetically distinct classes known to date, the α-, β-, γ-, δ- and ζ-CAs, use Zn(II), Cd(II), or Fe(II) at their active sitesCitation3–8,Citation17–25. The α-CAs are present in vertebrates, Bacteria, algae and cytoplasm of green plants, the β-CAs are predominantly found in Bacteria, algae and chloroplasts of both mono- as well as dicotyledons, the γ-CAs are present in Archaea, Bacteria and plants, whereas the δ- and ζ CAs, present in marine diatoms and other eukaryotes composing the planktonCitation2,Citation7,Citation11,Citation12,Citation16,Citation21,Citation26–30. These enzymes catalyze a very simple physiological reaction, the interconversion between carbon dioxide and the bicarbonate ion when a proton is also formed, being involved in crucial physiological processes connected with respiration and transport of CO2/bicarbonate between metabolizing tissues and lungs (in vertebrates), pH and CO2 homeostasis, electrolyte secretion in a variety of tissues/organs, biosynthetic reactions (such as gluconeogenesis, lipogenesis and ureagenesis – again in vertebrates), bone resorption, calcification and tumorigenicityCitation7,Citation17. In prokaryotes, the existence of genes encoding for CAs from at least three classes (α-, β- and γ-class) suggests that these enzymes play an important role in the prokaryotic physiology. CAs, in fact, are involved in the transport of CO2 or , in supplying CO2 or
for the biosynthetic reactions (and thus metabolisms); in pH regulation and also in xenobiotic degradation (e.g. cyanate by Escherichia coli), as well as in the survival of intracellular pathogens within their hostCitation11,Citation31,Citation32.
The microbiota of the human oral mucosa consists of a myriad of bacterial species that normally exist in commensal harmony with the host. Among the bacterial species living in the oral cavity, a bacterial complex named “red complex” and composed of Porphyromonas gingivalis, Treponema denticola, and Tannerella forsythia has been strongly associated with advanced periodontal lesionsCitation3,Citation11,Citation32,Citation33. Porphyromonas gingivalis is one of the few major pathogens responsible for the development of chronic periodontitis and a successful colonizer of the oral epithelium. The perturbation of epithelial cells by bacteria is the first stage in the initiation of inflammatory and immune processes causing eventually destruction of the tissues surrounding and supporting the teeth, which ultimately result in tooth loss. The genome of P. gingivalis encodes for β- and γ-CAs. Recently, our group purified the recombinant γ-CA (named PgiCA) identified in the genome of this pathogenic bacteriumCitation3,Citation7,Citation11,Citation32. PgiCA was shown to possess a significant catalytic activity for the reaction that converts the CO2 to bicarbonate and protons, with a kcat of 4.1 × 105 s−1 and a kcat/Km of 5.4 × 107 M−1 × s−1 Citation3,Citation11,Citation32. Like most enzymes belonging to the CA superfamily, PgiCA was also inhibited by acetazolamide with inhibition constants of 324 nM. We have also investigated the inhibition profile of the new enzyme with a range of inorganic anions such as thiocyanate, cyanide, azide, hydrogen sulfide, sulfamate and trithiocarbonateCitation3,Citation11,Citation32. These anions were effective PgiCA inhibitors with inhibition constants in the range of 41–97 μM. Other effective inhibitors were diethyldithiocarbamate, sulfamide, and phenylboronic acid, with KIs of 4.0–9.8 μMCitation3,Citation11,Citation32.
As many other bacteria, this pathogen also encodes for a β-CA, and in this article we describe the cloning, purification and kinetic parameters of the recombinant enzyme named here PgiCAb identified in the genome of P. gingivalis. The role of these enzymes as virulence factors of P. gingivalis is poorly understood at the moment but their good catalytic activity and the fact that they might be inhibited by diverse classes of compounds may be of great importance in considering CAs as antibacterial drug targets and the development of antibiotics with a novel mechanism of action.
Materials and methods
Enzyme purification
The synthetic P. gingivalis gene encoding PgiCAb was designed and produced by Life Technologies (Invitrogen, Carlsbad, CA), a company specialized in gene synthesis. The gene contains NdeI and XhoI restrictions sites at the 5′ and 3′ ends, respectively. The synthetic gene was ligated into the expression vector pET15-b (Novagen, Madison, WI) by T4 DNA ligase to form the expression vector pET15- b/PgiCAb. Arctic Express DE3 competent cells (Agilent Technologies, Santa Clara, CA), were transformed with pET15-b/PgiCAb, grown at 20 °C and induced with 1 mM isopropyl β-d-1-thiogalactopyranoside (IPTG). 0.5 mM ZnSO4 was added after 30 min incubation for uptake in the expressed protein. Arctic Express competent cells have been engineered for improved protein processing at low temperatures, which represents one strategy for increasing the recovery of soluble protein. At 6 h post-induction, cells were harvested and disrupted by sonication at 4 °C. Following centrifugation, the supernatant was loaded onto HIS-Select HF Nickel Affinity Gel (Sigma–Aldrich, St. Louis, MO) and the protein was eluted with 250 mM imidazole. At this stage of purification the enzyme was at least 90% pure.
SDS-PAGE
Sodium dodecyl sulfate (SDS)-polyacrylamide gel electrophoresis (PAGE) was performed asdescribed previously, using 12% gelsCitation34.
Enzyme kinetics
An applied photophysics stopped-flow instrument was used for assaying the CA catalysed CO2 hydration activityCitation35. Phenol red (at a concentration of 0.2 mM) was used as indicator, working at the absorbance maximum of 557 nm, with 20 mM HEPES (pH 7.5, for α-CAs) or 20 mM TRIS (pH 8.3 for the β- and γ-CAs) as buffers, and 20 mM NaClO4 for maintaining a constant ionic strength, following the initial rates of the CA-catalyzed CO2 hydration reaction for a period of 10–100 s. The CO2 concentrations ranged from 1.7 to 17 mM for the determination of the kinetic parameters and inhibition constants. For each inhibitor at least six traces of the initial 5–10% of the reaction have been used for determining the initial velocity. The uncatalyzed rates were determined in the same manner and subtracted from the total observed rates. Stock solutions of inhibitor (10–50 mM) were prepared in distilled-deionized water and dilutions up to 0.01 μM were done thereafter with the assay buffer. Inhibitor and enzyme solutions were pre-incubated for 15 min at room temperature prior to assay, in order to allow the formation of the E-I complex or for the eventual active site mediated hydrolysis of the inhibitor. The kinetic parameters were determined by using the Lineweaver–Burk plots whereas the inhibition constants were obtained by non-linear least squares methods using PRISM 3, as reported earlier, and represent the mean from at least three different determinationsCitation36–38. All CA isoforms were recombinant ones obtained in-house as reported earlier.
Phylogenetic analysis
A phylogenetic tree was constructed using the program PhylML 3.0 searching for the tree with the highest probabilityCitation39.
Results and discussion
IPTG induction of E. coli BL21 (DE3) cells transformed with the plasmid pET15-b/PgiCAb resulted in the production of the recombinant β-CA, named PgiCAb. The β-CA was isolated and purified to homogeneity from the E. coli (DE3) cell extract. Most of the CA activity was recovered in the soluble fraction of the cell extract after sonication and centrifugation. Using an affinity column (His-select HF Nickel affinity gel), PgiCA was purified to homogeneity. Analysis by SDS-PAGE of PgiCAb showed two main bands of about 25 kDA (monomeric form) and 50 kDA (dimeric form) under reducing condition (). The full nucleotide sequence showed an open reading frame encoding a 242 residues polypeptide chain which contained all the typical features of a β-CA, including the three residues that are involved in the catalytic mechanism of the enzyme (two cysteines and one histidine, see ). The predicted molecular mass of the enzyme from its amino acid sequence is of 26.1 kDa.
Figure 1. SDS-PAGE of the recombinant PgiCAb purified from E. coli. Lane STD, molecular markers; Lane 1, purified PgiCA from His-tag affinity column in its monomeric and dimeric forms.
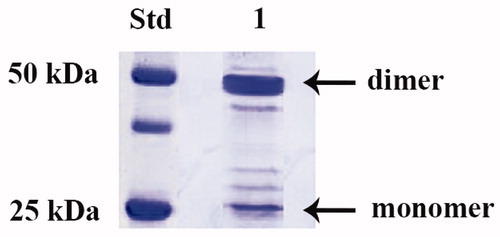
Figure 2. Nucleotide and amino acid sequence of β-CA from P. gingivalis. Amino acid residues participating in the coordination of metal ion are indicated in red, whereas the catalytic dyad involved in the activation of the metal ion coordinated water molecule (Asp97–Arg99) is shown in blue. The asterisk (*) indicates the stop codon.
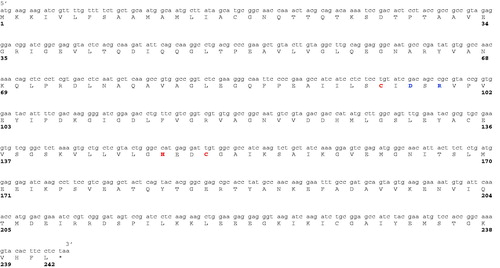
The first bacterial β-CA gene was named CynT and was identified in E. coli. Later, β-CAs were discovered in the genome of some other pathogenic bacteria, such as Helicobacter pylori, Mycobacterium tuberculosis, Salmonella typhimurium, Haemophilus influenzae, Brucella suis, Streptococcus pneumoniae, Salmonella enterica, and Vibrio choleraeCitation40. We identified a β-CA also in the pathogenic bacterium, Porphyromonas gingivalis. β-CAs have also been found in fungi, yeasts, cyanobacteria, carboxysomes of chemoautotrophic bacteria, green algae, red algae, nematodes, and insects. β-CAs, initially thought to be expressed only in plants, are in fact present in a wide variety of species, making it an attractive target for evolutionary studies and drug design campaignsCitation40.
shows the alignment of PgiCAb with those for β-CAs from several other species (bacteria, fungi, algae and plant). The multi-alignment, obtained using the program Clustal XCitation41, reveals a rather relevant homology existing between all these β-CAs. The amino acid residues characteristic of the catalytic site, consisting for the β-CA family of two cysteines and one histidine, are conserved for all these β-CAs. Furthermore, a catalytic dyad formed by one aspartate and one arginine residues (shown in blue in ) involved in the activation of the zinc-coordinated water molecule for the nucleophilic attack, is also conserved in all these enzymes. This multi-alignment is far from being exhaustive, as it does not take into account all the amino acid substitutions that differentiate the sequences of the β-CAs from different organisms. Hence, we have constructed a most parsimonious tree in order to better investigate the relationships of PgiCAb respect to the other β-CAs. From the dendrogram showed in , PgiCAb appears closely related to the β-CA from another pathogenic bacterium, Helicobacter pylori (named HpyCA), as both enzymes clustered in a branch distinct from that of the other β-CAs. Possibly, HpyCA and PgiCAb may be the result of evolution of an ancestral β-CA gene in these two pathogenic bacteria ().
Figure 3. Multi-alignment of the amino acid sequences of the selected β-CAs from plant, yeast, algae and bacterial organisms. Zinc ligands are indicated in red, catalytic dyad in blue. The asterisk (*) indicates identity at a position; the symbol (:) designates conserved substitutions, while (.) indicates semi-conserved substitutions. Multiple alignment was performed with the program Clustal W, version 2.1. Legend: PgiCAb_bacterium, Porhyromonas gingivalis (Accession number: YP_001929649.1); BsuCA_bacterium, Brucella suis (Accession number: WP_012243428.1); HpyCA_bacterium, Helicobacter pylori (Accession number: BAF34127.1); CspCA_alga, Coccomyxa sp. (Accession number: AAC33484.1); SceCA_yeast, Saccharomyces cerevisiae (Accession number: GAA26059); DbrCA_yeast, Dekkera bruxellensis AWRI1499 (Accession number: EIF49256); SpoCA_yeast, Schizosaccharomyces pombe (Accession number: CAA21790); FbiCA_plant, Flaveria bidentis, isoform I (Accession number: AAA86939.2); ZmaCA_plant; Zea mays (Accession number: NP_001147846.1).
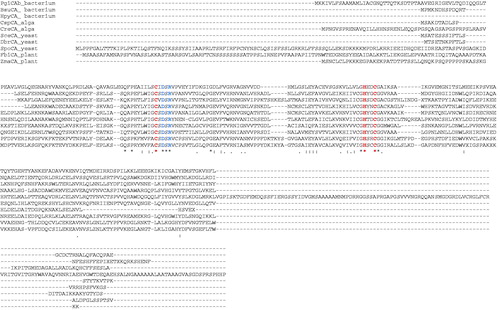
Figure 4. Phylogenetic tree of the β-CAs used in the multi-alignment showed in . The tree was constructed using the program PhyML 3.0. Branch support values are reported at branch points. For the legend see .
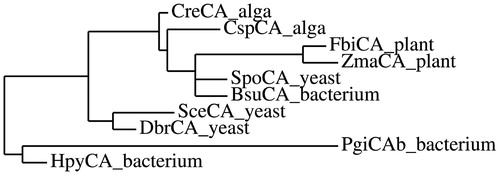
Using a stopped-flow techniques, the kinetic parameters were determined for the purified recombinant PgiCAb using CO2 as a substrate. PgiCAb showed a significant catalytic activity, with a kcat of 2.8 × 105 s−1 and a kcat/Km of 1.5 × 107 M−1 × s−1. shows a comparison of the kinetic parameters of PgiCAb and those of other CAs belonging to different family and from different organisms. CAs belonging to α-class resulted the faster CAs known to date, i.e. SazCA (from the extremophilic bacterium Sulfurihydrogenibium azorense) showed a kcat of 4.4 × 106 s−1; whereas the β- and γ-CAs showed kinetic constants ranging from 104 to 105 s−1. Thus, effective catalytic properties for the CO2 hydration reaction characterize all bacterial CA classes. Interestingly, in general the catalytic efficiency of the γ-CAs is lower compared to the β-CAs, which in turn are also less efficient catalysts compared to many bacterial α-CAs (). However, PgiCAb is about 2.3 times faster than the β-CA isolated from Flaveria bidentis but 1.5 times slower when compared with the γ-class enzyme PgiCA. It may also be observed that the CO2 hydrase activity of PgiCAb is inhibited by the clinically used sulfonamide acetazolamide, with an inhibition constant of 214 nM (PgiCA is on the other hand less inhibited by this compound, with a KI of 324 nM, as reported earlier by Del Prete et al.Citation11).
Table 1. Kinetic parameters for the CO2 hydration reaction catalyzed by various CAs belonging to the various families.
The fact that P. gingivalis, as many other bacteria encodes CAs from more than one class is still puzzling, although Capasso and Supuran recently presented a detailed phylogenetic analysis of bacterial CAs, according to which from the ancestral Ur-CA, the γ-class has arisen first, followed by the β-class, whereas the α-class CAs was the last originated CA class.
Conclusions
In the last decade many bacterial CAs identified in the genome of Nesseria spp., H. pylori, B. suis, S. pneumoniae and M. tuberculosis were subject to in vitro and in vivo inhibition studies with various classes of inhibitors, such as anions, sulfonamides, sulfamates, phenols and dithiocarbamatesCitation17. These studies evidenced the inhibition of bacterial growth in vivo, providing proof-of-concept data that bacterial CAs represent very promising targets for obtaining antibacterials devoid of the resistance problems of the clinically used agents. Further studies are however needed to validate these and other less investigated enzymes as drug targets. In addition, only a very limited number of bacterial genomes have been thoroughly examined for the presence of CAs. It is thus obvious that PgiCAb inhibition studies are needed in order to detect even stronger inhibitors, and eventually the role that this enzyme plays in the pathogenesis of P. gingivalis infection.
Acknowledgements
We thank The Distinguished Scientist Fellowship Program (DSFP) at KSU for funding this project.
Declaration of interest
This study was supported by the Distinguished Scientist Fellowship Program (DSFP) at KSU. The authors declare no conflict of interest.
References
- Capasso C, Supuran CT. Sulfa and trimethoprim-like drugs – antimetabolites acting as carbonic anhydrase, dihydropteroate synthase and dihydrofolate reductase inhibitors. J Enzyme Inhib Med Chem 2014;29:379-87
- Capasso C, Supuran CT. Anti-infective carbonic anhydrase inhibitors: a patent and literature review. Expert Opin Ther Pat 2013;23:693–704
- Vullo D, Del Prete S, Osman SM, et al. Sulfonamide inhibition studies of the gamma-carbonic anhydrase from the oral pathogen Porphyromonas gingivalis. Bioorg Med Chem Lett 2014;24:240–4
- Nishimori I, Vullo D, Minakuchi T, et al. Anion inhibition studies of two new beta-carbonic anhydrases from the bacterial pathogen Legionella pneumophila. Bioorg Med Chem Lett 2014;24:1127–32
- Nishimori I, Vullo D, Minakuchi T, et al. Sulfonamide inhibition studies of two beta-carbonic anhydrases from the bacterial pathogen Legionella pneumophila. Bioorg Med Chem 2014;22:2939–46
- Del Prete S, De Luca V, Scozzafava A, et al. Biochemical properties of a new alpha-carbonic anhydrase from the human pathogenic bacterium, Vibrio cholerae. J Enzyme Inhib Med Chem 2014;29:23–7
- Capasso C, Supuran CT. An overview of the alpha-, beta- and gamma-carbonic anhydrases from Bacteria: can bacterial carbonic anhydrases shed new light on evolution of bacteria? J Enzyme Inhib Med Chem 2014. [Epub ahead of print]. doi: 10.3109/14756366.2014.910202.
- Alafeefy AM, Abdel-Aziz HA, Vullo D, et al. Inhibition of carbonic anhydrases from the extremophilic bacteria Sulfurihydrogenibium yellostonense (SspCA) and S. azorense (SazCA) with a new series of sulfonamides incorporating aroylhydrazone-, [1,2,4]triazolo[3,4-b][1,3,4]thiadiazinyl- or 2-(cyanophenylmethylene)-1,3,4-thiadiazol-3(2H)-yl moieties. Bioorg Med Chem 2014;22:141–7
- Vullo D, De Luca V, Scozzafava A, et al. The alpha-carbonic anhydrase from the thermophilic bacterium Sulfurihydrogenibium yellowstonense YO3AOP1 is highly susceptible to inhibition by sulfonamides. Bioorg Med Chem 2013;21:1534–8
- De Luca V, Vullo D, Scozzafava A, et al. An alpha-carbonic anhydrase from the thermophilic bacterium Sulphurihydrogenibium azorense is the fastest enzyme known for the CO2 hydration reaction. Bioorg Med Chem 2013;21:1465–9
- Del Prete S, Vullo D, De Luca V, et al. A highly catalytically active gamma-carbonic anhydrase from the pathogenic anaerobe Porphyromonas gingivalis and its inhibition profile with anions and small molecules. Bioorg Med Chem Lett 2013;23:4067–71
- Akdemir A, Vullo D, De Luca V, et al. The extremo-alpha-carbonic anhydrase (CA) from Sulfurihydrogenibium azorense, the fastest CA known, is highly activated by amino acids and amines. Bioorg Med Chem Lett 2013;23:1087–90
- Vullo D, De Luca V, Scozzafava A, et al. The first activation study of a bacterial carbonic anhydrase (CA). The thermostable alpha-CA from Sulfurihydrogenibium yellowstonense YO3AOP1 is highly activated by amino acids and amines. Bioorg Med Chem Lett 2012;22:6324–7
- Vullo D, De Luca V, Scozzafava A, et al. Anion inhibition studies of the fastest carbonic anhydrase (CA) known, the extremo-CA from the bacterium Sulfurihydrogenibium azorense. Bioorg Med Chem Lett 2012;22:7142–5
- De Luca V, Vullo D, Scozzafava A, et al. Anion inhibition studies of an alpha-carbonic anhydrase from the thermophilic bacterium Sulfurihydrogenibium yellowstonense YO3AOP1. Bioorg Med Chem Lett 2012;22:5630–4
- Capasso C, De Luca V, Carginale V, et al. Biochemical properties of a novel and highly thermostable bacterial alpha-carbonic anhydrase from Sulfurihydrogenibium yellowstonense YO3AOP1. J Enzyme Inhib Med Chem 2012;27:892–7
- Supuran CT. Bacterial carbonic anhydrases as drug targets: toward novel antibiotics? Front Pharmacol 2011;2:34. doi: 10.3389/fphar.2011.00034
- Vullo D, Kupriyanova EV, Scozzafava A, et al. Anion inhibition study of the beta-carbonic anhydrase (CahB1) from the cyanobacterium Coleofasciculus chthonoplastes (ex-Microcoleus chthonoplastes). Bioorg Med Chem 2014;22:1667–71
- Vullo D, Flemetakis E, Scozzafava A, et al. Anion inhibition studies of two alpha-carbonic anhydrases from Lotus japonicus, LjCAA1 and LjCAA2. J Inorg Biochem 2014;136C:67–72
- Vullo D, Del Prete S, Osman SM, et al. Sulfonamide inhibition studies of the delta-carbonic anhydrase from the diatom Thalassiosira weissflogii. Bioorg Med Chem Lett 2014;24:275–9
- Rodrigues GC, Feijo DF, Bozza MT, et al. Design, synthesis, and evaluation of hydroxamic acid derivatives as promising agents for the management of Chagas disease. J Med Chem 2014;57:298–308
- Migliardini F, De Luca V, Carginale V, et al. Biomimetic CO2 capture using a highly thermostable bacterial alpha-carbonic anhydrase immobilized on a polyurethane foam. J Enzyme Inhib Med Chem 2014;29:146–50
- Del Prete S, Vullo D, Scozzafava A, et al. Cloning, characterization and anion inhibition study of the delta-class carbonic anhydrase (TweCA) from the marine diatom Thalassiosira weissflogii. Bioorg Med Chem 2014;22:531–7
- Del Prete S, Vullo D, De Luca V, et al. Biochemical characterization of the delta-carbonic anhydrase from the marine diatom Thalassiosira weissflogii, TweCA. J Enzyme Inhib Med Chem 2014. [Epub ahead of print]. doi: 10.3109/14756366.2013.868599
- Alafeefy AM, Abdel-Aziz HA, Vullo D, et al. Inhibition of human carbonic anhydrase isozymes I, II, IX and XII with a new series of sulfonamides incorporating aroylhydrazone-, [1,2,4]triazolo[3,4-b][1,3,4]thiadiazinyl- or 2-(cyanophenylmethylene)-1,3,4-thiadiazol-3(2H)-yl moieties. J Enzyme Inhib Med Chem 2014. [Epub ahead of print]. doi: 10.3109/14756366.2013.877897
- Vullo D, Leewattanapasuk W, Muhlschlegel FA, et al. Carbonic anhydrase inhibitors: inhibition of the beta-class enzyme from the pathogenic yeast Candida glabrata with sulfonamides, sulfamates and sulfamides. Bioorg Med Chem Lett 2013;23:2647–52
- Vullo D, De Luca V, Scozzafava A, et al. The extremo-alpha-carbonic anhydrase from the thermophilic bacterium Sulfurihydrogenibium azorense is highly inhibited by sulfonamides. Bioorg Med Chem 2013;21:4521–5
- Russo ME, Olivieri G, Capasso C, et al. Kinetic study of a novel thermo-stable alpha-carbonic anhydrase for biomimetic CO2 capture. Enzyme Microb Technol 2013;53:271–7
- Nishimori I, Vullo D, Minakuchi T, et al. Restoring catalytic activity to the human carbonic anhydrase (CA) related proteins VIII, X and XI affords isoforms with high catalytic efficiency and susceptibility to anion inhibition. Bioorg Med Chem Lett 2013;23:256–60
- Di Fiore A, Capasso C, De Luca V, et al. X-ray structure of the first `extremo-alpha-carbonic anhydrase', a dimeric enzyme from the thermophilic bacterium Sulfurihydrogenibium yellowstonense YO3AOP1. Acta Crystallogr D Biol Crystallogr 2013;69:1150–9
- Vullo D, Isik S, Del Prete S, et al. Anion inhibition studies of the alpha-carbonic anhydrase from the pathogenic bacterium Vibrio cholerae. Bioorg Med Chem Lett 2013;23:1636–8
- Del Prete S, De Luca V, Vullo D, et al. Biochemical characterization of the gamma-carbonic anhydrase from the oral pathogen Porphyromonas gingivalis, PgiCA. J Enzyme Inhib Med Chem 2013. [Epub ahead of print]. doi: 10.3109/14756366.2013.822371
- Mysak J, Podzimek S, Sommerova P, et al. Porphyromonas gingivalis: major periodontopathic pathogen overview. J Immunol Res 2014;2014:1–8
- Laemmli UK. Cleavage of structural proteins during the assembly of the head of bacteriophage T4. Nature 1970;227:680–5
- Khalifah RG. The carbon dioxide hydration activity of carbonic anhydrase. I. Stop-flow kinetic studies on the native human isoenzymes B and C. J Biol Chem 1971;246:2561–73
- Innocenti A, Supuran CT. Paraoxon, 4-nitrophenyl phosphate and acetate are substrates of alpha- but not of beta-, gamma- and zeta-carbonic anhydrases. Bioorg Med Chem Lett 2010;20:6208–12
- Innocenti A, Scozzafava A, Supuran CT. Carbonic anhydrase inhibitors. Inhibition of transmembrane isoforms IX, XII, and XIV with less investigated anions including trithiocarbonate and dithiocarbamate. Bioorg Med Chem Lett 2010;20:1548–50
- Maresca A, Scozzafava A, Kohler S, et al. Inhibition of beta-carbonic anhydrases from the bacterial pathogen Brucella suis with inorganic anions. J Inorg Biochem 2012;110:36–9
- Guindon S, Dufayard JF, Lefort V, et al. New algorithms and methods to estimate maximum-likelihood phylogenies: assessing the performance of PhyML 3.0. Syst Biol 2010;59:307–21
- Zolfaghari Emameh R, Barker H, Tolvanen ME, et al. Bioinformatic analysis of beta carbonic anhydrase sequences from protozoans and metazoans. Parasit Vectors 2014;7:1–12
- Larkin MA, Blackshields G, Brown NP, et al. Clustal W and Clustal X version 2.0. Bioinformatics 2007;23:2947–8