Abstract
A series of 5-benzylidenerhodanine-3-acetamides bearing morpholino-, 4-arylpiperazinyl-, or 4-benzylpiperidinyl- moieties were synthesized and their inhibitory activities against acetylcholinesterase (AChE) were evaluated. Alteration of amide part and substitution on the benzylidene moiety resulted in change of anti-AChE activity. The most active compound was the 1-benzylpiperidinyl derivative containing 4-(dimethylamino)benzylidene scaffold. Notably, the intermediate compounds, namely 5-arylidene-rhodanine-3-acetic acids (3), showed mild inhibitory activity against 15-lipoxygenase (15-LOX), while the final compound 4 showed no activity against 15-LOX.
Introduction
Rhodanines (2-thioxothiazolidin-4-ones) and their analogues have been known as privileged structures in drug discovery. Compounds containing the rhodanine ring have demonstrated a broad range of biological activitiesCitation1. Particularly, 5-arylidene rhodanines have been reported as small molecule inhibitors of diverse enzyme targets such as β-lactamaseCitation2, hepatitis C virus (HCV) NS3 proteaseCitation3, aldose reductaseCitation4, UDP-N-acetylmuramate/l-alanine ligaseCitation5, protein mannosyl transferase, JNK-stimulating phosphatase, cyclooxygenase, 5-lipoxygenaseCitation6 and tyrosinaseCitation7.
Historically, rhodanines have become a therapeutic class of compounds since the introduction of epalrestat (Z,E-[5-(2-methyl-3-phenyl-allylidene)-4-oxo-2-thioxo-thiazolidin-3-yl]-acetic acid) into clinical use for the treatment of diabetic complicationsCitation8. Epalrestat is a rhodanine-3-acetic acid analogue and acts as an aldose reductase inhibitorCitation9. Although, the rhodanine-based compounds are suspected to undergo conjugate addition in vivo, they are frequently employed in drug design, as there is no adverse effect (e.g. mutagenicity) correlated to this scaffoldCitation10. According to a long-term clinical study with epalrestat, the structure can be bioavailable and well-toleratedCitation11. Bulic et al. have been described a series of 5-arylidene-rhodanine-3-acetic acids as tau aggregation inhibitors potentially useful in the management of Alzheimer’s disease and related dementiasCitation12. Also, amide derivatives of 5-arylidene-rhodanine-3-acetic acid have been reported as anti-inflammatory agentsCitation13,Citation14.
In view of these facts, although extensive studies on the diverse bioactivity of 5-arylidene-rhodanines have been reported, the anti-acetylcholinesterase (AChE) activities of these prototypes have never appeared in the literature. Accordingly as part of our ongoing studies in developing new anti-AChE agentsCitation15–18, we have synthesized 5-benzylidenerhodanine-3-acetamides and evaluated their inhibitory activity against AChE. Also, the intermediate compounds 5-arylidene-rhodanine-3-acetic acids were screened for 15-lipoxygenase (15-LOX) inhibitory activity.
Experimental
Carbonyldiimidazole (CDI), dicyclohexylcarbodiimide (DCC), 1-ethyl-3-(3-dimethylaminopropyl)carbodiimide (EDC), hydroxyl benzotriazole (HOBt) and other commercially available reagents were used without further purification. TLC was conducted on silica gel 250 micron, F254 plates. Melting points were measured on a Kofler hot stage apparatus and are uncorrected. The IR spectra were taken using Nicolet FT-IR Magna 550 spectrograph (KBr disks). 1H NMR spectra were recorded on a NMR instrument Bruker 500 MHz. The chemical shifts (δ) and coupling constants (J) are expressed in parts per million and Hertz, respectively. Mass spectra of the products were obtained with an HP (Agilent Technologies, Santa Clara, CA) 5937 Mass Selective Detector. Elemental analyses were carried out by a CHN-Rapid Heraeus elemental analyzer. The results of elemental analyses (C, H, N) were within ±0.4% of the calculated values.
General procedure for the synthesis of compounds 3a-l
Compounds 3a–l were prepared according to the literatureCitation6.
General procedure for the synthesis of compounds 4a–n
A solution of compound 3 (1.0 mmol), EDC (1.1 mmol) and HOBt (1.0 mmol) in acetonitrile (10 ml) was stirred for 1 h at room temperature. Then, the corresponding amine (1.0 mmol) was added and stirred for 24 h at ambient temperature. After completion of the reaction (monitored by TLC), the solvent was evaporated and the residue was crystallized from chloroform-petroleum ether to give compound 4.
(Z)-5-(4-Cholorobenzylidene)-3-(2-morpholino-2-oxoethyl)-2-thioxothiazolidin-4one (4a)
Yield 69%, yellow solid, m.p. 225–227 °C, IR (cm−1, KBr): νmax 3325 (C–H aromatic), 2926 and 2850 (C–H aliphatic), 1716 and 1668 (C=O); 1H NMR (CDCl3, 500 MHz): δ 7.73 (s, 1H, vinylic H), 7.48 and 7.45 (2 d, 4H, H2,3,5,6, J = 8.7 Hz), 4.96 (s, 2H, CH2CO), 3.8 and 3.73 (2t, 4H, CH2O, J = 4.45 Hz), 3.64 and 3.59 (2t, 4H, CH2N, J = 4.45 Hz). EI-MS (m/z, %) 382 (M+, 19.6), 349 (26.7), 297 (34.6), 268 (37.7), 224 (13.3), 168 (100), 143 (14), 133 (23.6), 114 (14), 99 (31.4), 86 (98.4), 72 (53.5), 56 (77.9). Anal. (C16H15ClN2O3S2): C, H, N, Calcd 50.19, 3.95, 7.32. Found. 50.32, 3.71, 7.12.
(Z)-5-(4-(Dimethylamino)benzylidene)-3-(2-morpholino-2-oxoethyl)-2-thioxothiazolidin-4-one (4b)
Yield 52%, orange solid, m.p. <300 °C, IR (cm−1, KBr): νmax 3327 (C–H aromatic), 2926 and 2851 (C–H aliphatic), 1707 and 1644 (C=O); 1H NMR (CDCl3, 500 MHz): δ 7.72 (s, 1H, vinylic H), 7.42 (d, 2H, H2,4, J = 8.4 Hz), 6.75 (d, 2H, H3,5, J = 8.4 Hz), 4.95 (s, 2H, CH2CO), 3.80-3.59 (m, 8H, Morpholine), 3.10 (s, 6H, N(CH3)2). 13C NMR (DMSO-d6, 125 MHz): δ 193.2, 167.1, 163.4, 152.5, 133.7, 132.0, 120.2, 114.0, 112.7, 66.4, 47.9, 45.4, 45.2, 42.4. EI-MS (m/z, %) 391 (M+, 88), 358 (15.7), 278 (13.3), 224 (14), 177 (100), 161 (13.3), 56 (14). Anal. (C18H21N3O3S2): C, H, N, Calcd 55.22, 5.41, 10.73. Found. 55.48, 5.19, 10.53.
(Z)-5-(4-Methoxybenzylidene)-3-(2-morpholino-2-oxoethyl)-2-thioxothiazolidin-4-one (4c)
Yield 41%, yellow solid, m.p. 201–203 °C, IR (cm−1, KBr): νmax 2927 and 2850 (C–H aliphatic), 1702 and 1667 (C=O); 1H NMR (CDCl3, 500 MHz): δ 7.76 (s, 1H, vinylic H), 7.49 (d, 2H, H2,4, J = 8.75 Hz), 7.01 (d, 2H, H3,5, J = 8.85 Hz), 4.95 (s, 2H, CH2CO), 3.89 (s, 3H, OMe), 3.80 and 3.72 (2t, 4H,CH2O, J = 4.6 Hz), 3.65 and 3.59 (2t, 4H, CH2N, J = 4.6 Hz). 13C NMR (DMSO-d6, 125 MHz): δ 193.7, 167.1, 163.2, 162.1, 134.2, 133.5, 125.8, 119.2, 115.6, 66.4, 56.0, 47.9, 45.5, 45.2. EI-MS (m/z, %) 378 (M+, 78.7), 345 (59), 293 (63), 264 (46.4), 164 (100), 149 (99.2), 121 (45.6), 99 (20.4), 86 (72.4), 77 (27.5), 70 (41.7), 56 (55). Anal. (C17H18N2O4S2): C, H, N, Calcd 53.95, 4.79, 7.40. Found. 53.68, 4.54, 7.73.
(E and Z)-3-(2-Morpholino-2-oxoethyl)-2-thioxo-5-(3,4,5-trimethoxybenzylidene)thiazolidin-4-one (4d)
Yield 70%, yellow solid, m.p. 231–233 °C, IR (cm−1, KBr): νmax 3000 (C–H aromatic), 2935–2853 (C–H aliphatic), 1720 and 1671 (C=O); 1H NMR (CDCl3, 500 MHz, E/Z = 27/73): δ 7.86 (E) and 7.72 (Z) (2 s, 1H, vinylic H), 6.76 (E) and 6.74 (Z) (2 s, 2H, H2,6 phenyl), 4.96 (E) and 4.55 (Z) (2 s, 2H, CH2CO), 3.94 (E) and 3.93 (Z) (2 s, 3H, OMe), 3.93 (E) and 3.92 (Z) (2 s, 6H, OMe), 3.80 (E), 3.73 (Z) (2 m, 4H, Morpholine), 3.66 (E) and 3,59 (Z) (2 m, 4H, Morpholine). EI-MS (m/z, %) 438 (M+, 82.7), 405 (22), 353 (20), 325 (40), 265 (11), 224 (100), 209 (89), 181 (19.7), 166 (12.6), 86 (21.2), 70 (14.1), 56 (13.3). Anal. (C19H22N2O6S2): C, H, N, Calcd 52.04, 5.06, 6.39. Found. 51.86, 5.25, 6.14.
(Z)-3-(2-Oxo-2-(4-phenylpiperazine-1-yl)ethyl)-2-thioxo-5-(3,4,5-trimethoxybenzylidene) thiazolidin-4-one (4e)
Yield 67%, yellow solid, m.p. 192–194 °C, IR (cm−1, KBr): νmax 2932 and 2833 (C–H aliphatic), 1651 and 1599 (C=O); 1H NMR (CDCl3, 500 MHz): δ 7.71 (s, 1H, vinylic H), 7.34 (m, 2H, phenyl), 6.97 (m, 3H, phenyl), 6.75 (s, 2H, H2,6), 5.02 (s, 2H,CH2CO), 3.93 (s, 9H, OMe), 3.81 and 3.26 (m, 8H, piperazine). EI-MS (m/z, %) 513 (M+, 42.5), 480 (45.6), 375 (18), 352 (15.7), 324 (14), 265 (11.8), 224 (28.3), 209 (41.7), 181 (16.5), 160 (62.7), 132 (100), 120 (15), 104 (35.4), 91 (11.8), 77 (19.7), 56 (56). Anal. (C25H27N3O5S2): C, H, N, Calcd 58.46, 5.30, 8.18. Found. 58.22, 5.51, 8.01.
(Z)-3-(2-(4-(2-Fluorophenyl)piperazin-1-yl)-2-oxoethyl)-5-(4-methoxybenzylidene)-2-thioxothiazolidin-4-one (4f)
Yield 49%, yellow solid, m.p. 259–260 °C; 1H NMR (CDCl3, 500 MHz): δ 7.76 (s, 1H, vinylic H), 7.49 (d, 2H, H2,6, J = 8.85 Hz), 7.14–6.90 (m, 6H, fluorophenyl and H3,5 methoxyphenyl), 5.01 (s, 2H, benzylic H), 3.89 (s, 3H, OMe), 3.82-3.10 (m, 8H, piperazine). EI-MS (m/z, %) 471 (M+, 34.6), 438 (56.7), 315 (28.3), 292 (18.9), 264 (30.7), 220 (12.6), 178 (100), 164 (69.3), 150 (80.3), 138 (15), 122 (48), 109 (11.8), 95 (11.8), 77 (17.3), 56 (67). Anal. (C23H22FN3O3S2): C, H, N, Calcd 58.58, 4.70, 4.03. Found 58.39, 4.93, 4.36.
(Z)-3-(2-(4-(3-Fluorophenyl)piperazin-1-yl)-2-oxoethyl)-2-thioxo-5-(3,4,5-trimethoxybenzylidene)thiazolidin-4-one (4g)
Yield 52%, yellow solid, m.p. 202–204 °C, IR (cm−1, KBr): νmax 3414 (C–H aromatic), 2928 and 2837 (C–H aliphatic), 1718–1648 (C=O); 1H NMR (CDCl3, 500 MHz): δ 7.70 (s, 1H, vinylic H), 7.32 (d, 2H, H2,6 fluorophenyl, J = 8.95 Hz), 6.99 (d, 1H, H4 fluorophenyl), 6.77 (dd, 1H, H5 fluorophenyl, J = 2.6 Hz), 6.74 (s, 2H, H2,6), 4.99 (s, 2H, CH2CO), 3.93 (s, 9H, OMe), 3.79 and 3.19 (m, 8H, piperazine). EI-MS (m/z, %) 531 (M+, 8.6), 498 (11), 279 (10.2), 241 (16.5), 224 (25.2), 209 (30.7), 167 (26.7), 149 (68.5), 129 (96), 111 (44), 97 (40), 83 (52.7), 71 (58.3), 57 (100). Anal. (C25H26FN3O5S2): C, H, N, Calcd 56.48, 4.93, 7.90. Found. 56.19, 4.67, 7.70.
(Z)-3-(2-(4-(4-Fluorophenyl)piperazin-1-yl)-2-oxoethyl)-2-thioxo-5-(3,4,5-trimethoxybenzylidene)thiazolidin-4-one (4h)
Yield 64%, yellow solid, m.p. 200–202 °C, IR (cm−1, KBr): νmax 3432 (C–H aromatic), 2922 and 2853 (C–H aliphatic), 1706 and 1664 (C=O); 1H NMR (CDCl3, 500 MHz): δ 7.71 (s, 1H, vinylic H), 7.00 (t, 2H, H2,6 fluorophenyl, J = 8 Hz), 6.92 (m, 2H, H3,5 fluorophenyl), 6.74 (s, 2H, H2,6), 5.01 (s, 2H, CH2CO), 3.93 (s, 9H, OMe), 3.76 and 3.17 (m, 8H, piperazine). EI-MS (m/z, %) 531 (M+, 48), 498 (56), 375 (16.5), 352 (19.7), 324 (18), 265 (11.8), 224 (35.4), 209 (50.3), 178 (79.5), 150 (100), 138 (15), 122 (36.2), 109 (10.2), 95 (17.3), 56 (50.4). Anal. (C25H26FN3O5S2): C, H, N, Calcd 56.48, 4.93, 7.90. Found. 56.75, 4.65, 7.61.
(Z)-3-(2-(4-(3,4-Dichlorophenyl)piperazin-1-yl)-2-oxoethyl)-5-(2,3-dimethoxybenzylidene)-2-thioxothiazolidin-4-one (4i)
Yield 25%, yellow solid, m.p. 144–146 °C, IR (cm−1, KBr): νmax 3431(C–H aromatic), 2929–2841 (C–H aliphatic), 1710 and 1670 (C=O); 1H NMR (CDCl3, 500 MHz): δ 8.13 (s, 1H, vinylic H),7.34 (d, 1H, H2 phenyl, J = 8.8 Hz), 7.17 (t, 1H, H3 phenyl, J = 8 Hz), 7.08 and 7.04 (m, 3H, dicholorophenyl), 6.83 (m, 1H, H4, J = 2.5 Hz), 5.01 (s, 2H, CH2CO), 3.90 (s, 6H, OMe), 3.82 and 3.12 (d, 8H, piperazine). EI-MS (m/z, %) 551 (M+, 30.7), 518 (52.7), 345 (30), 322 (66), 294 (81.8), 270 (25.2), 228 (100), 213 (17.3), 200 (93.7), 179 (42.5), 161 (21.2), 149 (26), 136 (21.2), 121 (18), 108 (12.6), 91 (11), 72 (26), 56 (86.6). Anal. (C24H23Cl2N3O4S2): C, H, N, Calcd 52.18, 4.20, 7.61. Found. 52.35, 4.01, 7.34.
(Z)-3-(2-(4-Benzylpiperidin-1-yl)-2-oxoethyl)-5-(4-(dimethylamino)benzylidene)-2-thioxothiazolidin-4-one (4j)
Yield 63%, yellow solid, m.p. 229–230 °C, IR (cm−1, KBr): νmax 2910 and 2853 (C–H aliphatic), 1710 and 1647 (C=O); 1H NMR (CDCl3, 500 MHz): δ 7.71 (s, 1H, vinylic H), 7.45 (d, 2H, H2,6, J = 8.5 Hz), 7.32 (t, 2H, H3,5 benzyl, J = 8.2 Hz), 7.23 (t, 1H, H4 benzyl, J = 8.2 Hz), 7.17 (d, 2H, H2,6 benzyl, J = 8.2 Hz), 6.89 (d, 2H, H3,5, J = 8.5 Hz), 4.97 and 4.93 (2 d, 2H, CH2CO), 4.54 and 3.85 (2 d, 2H, CH2 benzylic, J = 13.8 Hz), 2.60 (m, 4H, CH2N), 1.80–1.20 (m, 5H, CH2 and CH). 13C NMR (DMSO-d6, 125 MHz): δ 193.2, 167.1, 162.6, 152.5, 140.4, 135.3, 133.7, 129.4, 128.6, 126.3, 120.2, 114.1, 112.7, 45.6, 44.8, 42.4, 42.3, 37.6, 31.7. EI-MS (m/z, %) 479 (M+, 47.2), 446 (22), 378 (31.4), 345 (22.8), 293 (21.2), 278 (11), 264 (22), 231 (11), 177 (100), 164 (81), 149 (33.8), 121 (14), 91 (19.6), 72 (10.2), 56 (11). Anal. (C26H29N3O2S2): C, H, N, Calcd 65.11, 6.09, 8.76. Found. 65.34, 6.32, 8.53.
(E and Z)-3-(2-(4-Benzylpiperidin-1-yl)-2-oxoethyl)-5-(3-hydroxy-4-methoxybenzylidene)-2-thioxothiazolidin-4-one (4k)
Yield 34%, yellow solid, m.p. 278–280 °C, IR (cm−1, KBr): νmax 2923 and 2853 (C–H aliphatic), 1706 and 1646 (C=O); 1H NMR (CDCl3, 500 MHz, E/Z = 22/78): δ 7.83 (E) and 7.68 (Z) (2 s, 1H, vinylic H), 7.37 (E) and 7.32 (Z) (t, 2H, H3,5 benzyl, J = 7.3 Hz), 7.26 (E) and 7.22 (Z) (t, 1H, H4 benzyl, J = 7.3 Hz), 7.17 (E) and 7.08 (Z) (m, 2H, phenyl), 6.95 (E) and 6.58 (Z) (dd, 1H, H6 phenyl, J = 8.2 and 1.9 Hz), 4.95 (E) and 4.53 (Z) (m, 2H, CH2CO), 4.53 (E) and 4.16 (Z) (m, 2H, CH2N), 3.98 (Z) and 3.97 (E) (2 s, 3H, OMe), 2.60 (E) and 2.43 (Z) (m, 2H, CH2 benzylic), 1.85-1.30 (E and Z) (m, 5H, CH2 and CH Piperidine). EI-MS (m/z, %) 480 (M+, 7), 466 (26.7), 449 (14.1), 438 (39.3), 413 (15), 384 (45.6), 368 (16.5), 356 (26), 325 (26), 313 (10.2), 298 (15), 280 (15), 265 (19), 251 (14.1), 237 (13.4), 224 (90), 209 (100), 180 (79.5), 165 (48.8), 150 (60.6), 137 (39.3), 122 (33.8), 109 (21.2), 91 (60), 69 (26.7), 56 (43.3). Anal. (C25H26N2O4S2): C, H, N, Calcd 62.22, 5.43, 5.80. Found. 62.54, 5.27, 5.67.
2-((Z)-5-(4-Chlorobenzylidene)-4-oxo-2-thioxothiazolidin-3-yl)-N-(1-benzylpiperidin-4-yl)acetamide (4l)
Yield 43%, yellow solid, m.p. > 250 °C. IR (cm−1, KBr): νmax 3300 (NH), 3088 and 3023 (C–H aromatic), 2930 (C–H aliphatic), 1714 and 1656 (C=O); 1H NMR (DMSO-d6, 500 MHz): δ 8.50-8.60 (br, s, 1H, NH), 7.70 (s, 1H, vinylic H), 8.00–8.30 (m, 4H, Ar-H), 7.40–7.80 (m, 5H, Ar-H of benzyl), 4.80 (s, 2H, COCH2N), 3.35–3.65 (br, s, 3H, CHNH & benzylic CH2), 2.40–2.85 (m, 4H, 2CH2N piperidine), 1.40–2.20 (m, 4H, 2 CH2CH2N piperidine). EI-MS (m/z, %) 485 (M+, 27), 385 (5), 314 (7.7), 297 (6.9), 268 (7.5), 230 (3.8), 203 (12.3), 172 (93.8), 165 (31.5), 146 (6), 133 (16.9), 125 (23), 99 (3), 91 (98), 82 (100), 65 (7.5), 42 (10). Anal. (C24H24ClN3O2S2): C, H, N, Calcd 59.31, 4.98, 8.65. Found. 59.65, 4.76, 8.91.
2-((Z)-5-(4-(Dimethylamino)benzylidene)-4-oxo-2-thioxothiazolidin-3-yl)-N-(1-benzylpiperidin-4-yl)acetamide (4m)
Yield 40%, orange solid, m.p. >250 °C. IR (cm−1, KBr): νmax 3292 (NH), 3082 and 3021 (C–H aromatic), 2930 (C–H aliphatic), 1698 and 1655 (C=O); 1H NMR (DMSO-d6, 500 MHz): δ 8.20 (br, s, 1H, NH), 7.70 (s, 1H, vinylic H), 6.85–7.50 (m, 9H, Ar-H), 4.60 (s, 2H, COCH2N), 3.35–3.50 (br, s, 3H, CHNH and benzylic CH2), 3.05 (s, 6H, 2 CH3), 2.50–2.95 (m, 4H, CH2N piperidine), 1.40–2.30 (m, 4H, 2 CH2CH2N piperidine). 13C NMR (DMSO-d6, 125 MHz): δ 193.3, 167.1, 164.1, 152.4, 135.2, 133.6, 129.2, 128.6, 127.4, 120.2, 114.3, 112.7, 62.4, 52.1, 46.7, 46.5, 31.8. EI-MS (m/z, %) 494 (M+,4.5), 465 (8.3), 439 (25), 421 (5.3), 368 (9), 334 (7.5), 292 (11.3), 248 (12), 215 (13.6), 187 (6.8), 172 (96), 148 (43), 105 (41), 91 (100), 82 (53), 77 (18.9), 65 (13.6), 43 (25.5). Anal. (C26H30N4O2S2): C, H, N, Calcd 63.13, 6.11, 11.33. Found. 63.51, 6.28, 11.03.
2-((Z)-5-(4-Methylbenzylidene)-4-oxo-2-thioxothiazolidin-3-yl)-N-(1-benzylpiperidin-4-yl)acetamide (4n)
Yield 38%, yellow solid, m.p. >250 °C. IR (cm−1, KBr): νmax 3299 (NH), 3082 and 3020 (C–H aromatic), 2924 (C–H aliphatic), 1716 and 1663 (C=O); 1H NMR (DMSO-d6, 500 MHz): δ 8.05–8.27 (br, s, 1H, NH), 7.80 (s, 1H, vinylic H), 7.20–7.70 (m, 9H, Ar-H), 4.62 (s, 2H, COCH2N), 3.30–3.60 (br, s, 3H, CHNH and benzylic CH2), 2.45–2.95 (m, 4H, 2CH2N piperidine), 2.38 (s, 3H, CH3), 1.35–2.30 (m, 4H, 2 CH2CH2N piperidine). 13C NMR (DMSO-d6, 125 MHz): δ 193.9, 167.1, 164.4, 142.1, 134.0, 131.7, 131.2, 130.6, 130.1, 130.0, 129.3, 121.4, 59.5, 51.0, 46.5, 44.7, 29.0, 21.5. EI-MS (m/z, %) 465 (M+, 14.3), 276 (14), 248 (18.9), 172 (100), 148 (35), 132 (11.3), 118 (7.5), 91 (90), 82 (53), 72 (4.5), 56 (2.2), 42 (1.5). Anal. (C25H27N3O2S2): C, H, N, Calcd 64.49, 5.84, 9.02. Found. 64.21, 5.76, 9.28.
Molecular modelling studies
All docking studies were performed using Autodock Vina (ver. 1.1.1, Florida, CA)Citation19. The crystal structures of AChE complexed with E2020 (code ID: 1EVE) and soybean lypoxygenase complexed with 13(S)-hydroproxy-9(Z)-2,11(E)-octadecadienoic acid (code ID: 1IK3) were retrieved from protein data bank. In both cases, the co-crystallized ligand and water molecules were removed. Then the Fe in the structure of soybean lipoxygenase was modified to Fe(III)–OH and both proteins were converted to pdbqt format using Autodock Tools (1.5.4, Florida, CA)Citation20.
The 2D structures of ligands were sketched using MarvinSketch 5.8.3, 2012, ChemAxon (http://www.chemaxon.com) and then converted to 3D and pdbqt format by Openbabel (ver. 2.3.1, Pittsburgh, PA)Citation21.
The docking parameters were as follows:
LOX: size_x = 20; size_y = 20; size_z = 20; center_x = 19.693; center_y = 0.054; center_z = 17.628; exhaustiveness = 100; num_modes = 15.
AChE: center_x = 2.023; center_y = 63.295; center_z = 67.062; size_x = 25; size_y = 25; size_z = 25; exhaustiveness = 80; num_modes = 15. The other parameters were left as default for both of target enzymes. Finally, the conformations with the most favorable free energy of binding were selected for analyzing the interactions between the target enzyme and inhibitor. All the 3D models are generated using the Chimera 1.6 software (San Francisco, CA)Citation22.
AChE inhibition assay
The inhibitory potency of target compounds on AChE was determined using Ellman’s methodCitation23. The test compounds were dissolved in DMSO (1 ml) and phosphate buffer (9 ml, pH = 8) and then diluted using phosphate buffer to achieve five concentrations in the range from 10−3 to 10−7 M. The reaction mixture included 100 µl of DTNB 0.1 M, 50 µl of enzyme (2 U/ml) and 50 µl of inhibitor solution. The changing of the absorbance was measured at 412 nm for 2 min after addition of 10 µl of substrate (acetylthiocholine iodide) 0.15 M to the reaction mixture. The IC50 values were determined graphically from inhibition curves (log inhibitor concentration versus percent of inhibition). All experiments were performed on a UV-2100 Rayleigh Double Beam Spectrophotometer in triplicate.
15-LOX inhibition assay
LOX inhibitory activity of target compounds was studied by a spectrophotometric assayCitation24. The tested compounds were dissolved in DMSO (1 ml) and phosphate buffer (9 ml, 0.1 M, pH = 8). This stock solution was added to test solution containing enzyme (final concentration: 167 U/ml), phosphate buffer (pH = 8) to achieve concentrations in range of 10−3 to 10−6. After incubation of test solution for 4 min, substrate (linoleic acid, final concentration: 134 µM) was added and change in the absorbance was measured for 60 s at 234 nm. The enzyme solution was kept in ice and controls were measured at intervals throughout the experimental periods to ensure that the enzyme activity was constant. All experiments were performed and then analyzed as mentioned above for AChE assay.
Results and discussion
Chemistry
In this work, we describe synthesis of a novel series of 2-((Z)-5-benzylidene-4-oxo-2-thioxothiazolidin-3-yl)acetamide 4a–n. As outlined in Scheme 1, initially, an aldol condensation of rhodanine-3-acetic acid 1 with different aldehydes 2 in the presence of catalytic amount of S-proline yielded intermediate 3. Under these conditions, (Z)-configuration was obtained as major product. In the 1H NMR spectroscopy, the vinylic proton located on the exocyclic double bond was assigned as a singlet ranging from 7.71 to 8.13 ppm. According to the literature reports, vinilic proton in the (Z)-isomers appeared at 7.7–8.5 ppm downfield compared to the (E)-isomersCitation25,Citation26. It would be due to the deshielding effect of the carbonyl group. Finally, the target compounds 4a–n were obtained via condensation of the carboxylic acids 3 with appropriate amines. Several coupling agents including CDI, DCC, EDC and HOBt in different solvents were investigated, but the best result was obtained by using EDC/HOBt in acetonitrile.
In vitro anti-AChE activity
The potential of the target compounds 4a–n to inhibit AChE activity was assessed by the Ellman method. The inhibitory activity (IC50 values, µM) of the test compounds in comparison to tacrine as reference drug was listed in . The most active compound was 1-benzylpiperidinyl derivative 4m with IC50 value of 21.0 µM. The N-(3-fluorophenyl)piperazinyl derivative 4g with IC50 value of 22.4 µM was as potent as compound 4m. Furthermore, compounds 4b, 4f, 4h–l, and 4n showed remarkable inhibitory activity against AChE in concentrations less than 64 µM.
Table 1. Chemical structure and eelAChE inhibition data of target compounds 4a–n.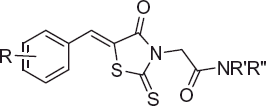
Among the morpholine compounds 4a–d, 4-(N,N-dimethylamino)-benzylidene derivative 4b exhibited higher activity. As evidenced from IC50 values of 4a, 4c and 4d, the introduction of 4-chloro-, 4-methoxy- and 3,4,5-trimethoxy- substituents dramatically diminished the activity. In the N-phenylpiperazine series 4e–i, the unsubstituted analogue 4e showed weak activity (IC50 value = 285 µM), while remaining congeners 4f–i showed good activities in the range of 22.4–61.9 µM. The comparison of IC50 values of unsubstituted compound 4e with 3-fluoro- and 4-fluoro- derivatives (4g and 4h, respectively) revealed that fluoro- group increased the anti-AChE activity. Moreover, by comparing compound 4e with 4d or 4f with 4c demonstrated that N-phenylpiperazine moiety is more favorable than morpholine attached to the acetamide linker. In the case of compounds containing 4-(N,N-dimethylamino)- substituent, morpholine derivative 4b and 4-benzylpiperidine analogue 4j showed nearly same activity, and 1-benzylpiperidin-4-yl derivative 4m was more potent than the latter compounds.
In vitro anti-15-LOX activity
Since the 5-LOX inhibitory activity of 5-benzylidenerhodanine-3-acetic acid has been reported,Citation6 thus we investigated the 15-LOX inhibitory potential of the intermediate compounds 3a–e against soybean 15-LOX enzyme (). For structure–activity relationships study, the 5-benzylidenerhodanine-3-acetic acid series was completed by synthesizing compounds 3f–l and evaluating their 15-LOX inhibitory activity. The results in comparison with quercetin were summarized in . All compounds with the exception of compounds 3b and 3g showed mild inhibitory activity against 15-LOX (IC50 values = 147–421 µM). The 2-hydroxy-4-bromo and 2,4-dihydroxy derivatives (compounds 3f and 3h, respectively) with IC50 values ≤149 µM, were the most active compounds. The comparison of unsubstituted compound 3l with substituted benzylidene compounds 3c, 3g, and 3i revealed that the introduction of 4-(N,N-dimethylamino), 4-methoxy, 3-bromo-4,5-dimethoxy and 2-hydroxy substituents decreased the anti-LOX activity. In contrast, the insertion of 3,4,5-trimethoxy, 2,3-dimethoxy, 2-hydroxy-4-methoxy, 2,4-dihydroxy, 2-methoxy, and 2-bromo substituents on the benzylidene moiety of compound 3l resulted in more active compounds. Interestingly, the inhibitory activity of 3,4,5-trimethoxy analogue (compounds 3d) was superior than that of 4-methoxy derivative 3c. Also, compound 3 h having 2,4-dihydroxy group showed higher inhibition than 2-hydroxy congener 3i. These findings demonstrated that the second hydroxyl substitution on the 4-position of 2-hydroxybenzylidene compound 3i improved the inhibitory activity against 15-LOX. By comparing the IC50 values of compounds 3j and 3i, it is revealed that the O-methylation of the 2-OH is favorable. Notably, formation of amides with various amines that yield final compounds 4a–n, led to inactive compounds toward lipoxygenase enzyme.
Table 2. Chemical structure and soybean 15-LOX inhibition activity data of 5-arylidene-rhodanine-3-acetic acids 3a–l.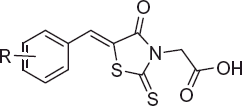
Docking studies
Molecular docking study was performed using the most active compound 4m to investigate the possible interactions with amino acid residues on the active site of the AChE. The interaction of 4m and the residues in the active site of eelAChE was illustrated in . The compound was spanning along the narrow gorge of the enzyme from peripheral anionic binding site (PAS) to catalytic anionic site (CAS). The active site of the enzyme is lined with aromatic residues, which comprise 40% of the residues in the binding pocket. The most active compound 4m takes advantage of these residues by making a variety of interactions. The positively charged piperidine nitrogen makes a cation--π interaction with Phe330 that is essential for ligand recognition. The benzyl ring of the compound shows π–π stacking with the aromatic ring of the Trp84. In such orientation, the pendant fragment including the rhodanine is stabilized by making π–π stacking with Trp279 through the 4-(dimethylamino)phenyl part of the molecule that is positioned at the rim of the gorge.
Figure 1. A representative model for interaction of compound 4m and the AChE. The residues involved in the interaction are shown.
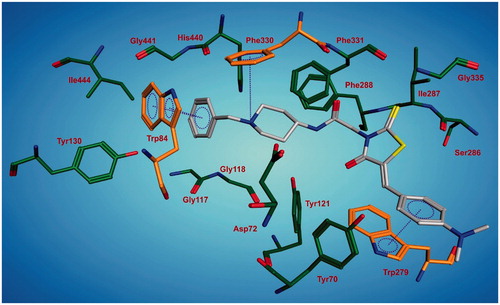
In the case of 15-LOX enzyme, the best LOX inhibitor, 3h, was docked into the LOX active site pocket while the Fe core had been modified to Fe(III)-OH. Analysis of docking results revealed that free carboxylic group of compound 3 h, oriented toward Fe–OH and interfered with it through a hydrogen bonding. It is further stabilized through additional hydrogen bonding formed between 2-hydroxy group of phenyl moiety and Ser510 and His513 (). As mentioned above, all final compounds 4a–k were inactive toward lipoxygenase enzyme. This reconfirmed the importance of free carboxylic group for binding of these compounds to target enzyme and their LOX inhibitory activity.
Figure 2. Representation of the best docked pose of 3h in the active site of 15-LOX enzyme. Hydrogen bonds are shown as red-dotted lines and Fe(III) also is shown as ball model. (For interpretation of the references to colour in this caption, the reader is referred to the online version of this article.)
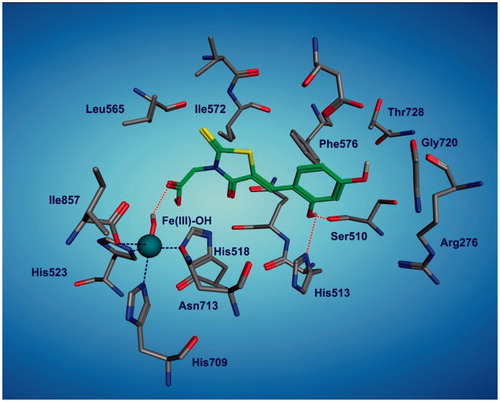
Conclusion
We have synthesized a series of 5-benzylidenerhodanine-3-acetamides bearing morpholino-, 4-arylpiperazinyl-, or 4-benzylpiperidinyl- moieties and evaluated their inhibitory activity against AChE. Alteration of amide part and substitution on the benzylidene moiety resulted in changing of anti-AChE activity. The better result was obtained with 4-(3-fluorophenyl)piperazin-1-yl derivative 4g containing 3,4,5-trimethoxybenzylidene scaffold and 1-benzylpiperidinyl derivative 4m bearing 4-(N,N-dimethylamino)benzylidene moiety.
Besides AChE, most intermediate compounds 3 showed mild inhibitory activity against 15-LOX. The 2-hydroxy-4-bromo and 2,4-dihydroxy derivatives (compounds 3f and 3h, respectively) were the most active compounds.
Supplementary material available online
Supplementary Figures.
Acknowledgements
Chimera is developed by the 25 Resource for Biocomputing, Visualization, and Informatics at the University of California, San Francisco.
Declaration of interest
The authors have declared no conflict of interest.
The work was supported by grant from International Campus, Tehran University of Medical Sciences.
References
- Sortino M, Delgado P, Juárez S, et al. Synthesis and antifungal activity of (Z)-5-arylidenerhodanines. Bioorg Med Chem 2007;15:484–94
- Grant EB, Guiadeen D, Baum EZ, et al. The synthesis and SAR of rhodanines as novel class C beta-lactamase inhibitors. Bioorg Med Chem Lett 2000;10:2179–82
- Sing WT, Lee CL, Yeo SL, et al. Arylalkylidene rhodanine with bulky and hydrophobic functional group as selective HCV NS3 protease inhibitor. Bioorg Med Chem Lett 2001;11:91–4
- Fujishima H, Tsubota K. Improvement of corneal fluorescein staining in post cataract surgery of diabetic patients by an oral aldose reductase inhibitor, ONO-2235. Br J Ophthalmol 2002;86:860–3
- Sim MM, Ng SB, Buss AD, et al. Benzylidene rhodanines as novel inhibitors of UDP-N-acetylmuramate/l-alanine ligase. Bioorg Med Chem Lett 2002;12:697–9
- Irvine MW, Patrick GL, Kewney J, et al. Rhodanine derivatives as novel inhibitors of PDE4. Bioorg Med Chem Lett 2008;18:2032–7
- Liu J, Wu F, Chen L, et al. Evaluation of dihydropyrimidin-(2H)-one analogues and rhodanine derivatives as tyrosinase inhibitors. Bioorg Med Chem Lett 2011;21:2376–9
- Terashima H, Hama K, Yamamoto R, et al. Effects of a new aldose reductase inhibitor on various tissues in vitro. J Pharmacol Exp Ther 1984;229:226–30
- Murata M, Fujitani B, Mizuta H. Synthesis and aldose reductase inhibitory activity of a new series of 5-[[2-(ω-carboxyalkoxy)aryl]methylene]-4-oxo-2-thioxothiazolidine derivatives. Eur J Med Chem 1999;34:1061–70
- Bulic B, Pickhardt M, Schmidt B, et al. Development of tau aggregation inhibitors for Alzheimer's disease. Angew Chem Int Ed 2009;48:1740–52
- Hotta N, Akanuma Y, Kawamori R, et al. Long-term clinical effects of epalrestat, an aldose reductase inhibitor, on diabetic peripheral neuropathy: the 3-year, multicenter, comparative Aldose Reductase Inhibitor-Diabetes Complications Trial. Diabetes Care 2006;29:1538–44
- Bulic B, Pickhardt M, Khlistunova I, et al. Rhodanine-based tau aggregation inhibitors in cell models of tauopathy. Angew Chem Int Ed 2007;46:9215–19
- Roman OM, Nektegayev IO, Lesyk RB. Bioactive compounds among 5-arylidenerhodanine 3-carboxylic acids. Pharm J 2002;5:47–51 (in Ukrainian)
- Roman OM, Nektegayev IO, Mountains VJ, et al. Synthesis and anti-inflammatory activity of 3-aryl-5-arylidene-2-thioxothiazolidin-4-ones. Pharm J 2002;3:56--9 (in Ukrainian)
- Alipour M, Khoobi M, Nadri H, et al. Synthesis of some new 3-coumaranone and coumarin derivatives as dual inhibitors of acetyl- and butyrylcholinesterase. Arch Pharm 2013;346:577–87
- Khoobi M, Alipour M, Sakhteman A, et al. Design, synthesis, biological evaluation and docking study of 5-oxo-4,5-dihydropyrano[3,2-c]chromene derivatives as acetylcholinesterase and butyrylcholinesterase inhibitors. Eur J Med Chem 2013;68:260–9
- Razavi SF, Khoobi M, Nadri H, et al. Synthesis and evaluation of 4-substituted coumarins as novel acetylcholinesterase inhibitors. Eur J Med Chem 2013;64:252–9
- Nadri H, Pirali-Hamedani M, Moradi A, et al. 5,6-Dimethoxybenzofuran-3-one derivatives: a novel series of dual acetylcholinesterase/butyrylcholinesterase inhibitors bearing benzyl pyridinium moiety. Daru 2013;21:15. doi: 10.1186/2008-2231-21-15
- Trott O, Olson AJ. AutoDock Vina: improving the speed and accuracy of docking with a new scoring function, efficient optimization, and multithreading. J Comput Chem 2010;31:455–61
- Sanner MF. Python: a programming language for software integration and development. J Mol Graph Model 1999;17:57–61
- O'Boyle NM, Banck M, James CA, et al. Open babel: an open chemical toolbox. J Cheminform 2011;3:33. doi: 10.1186/1758-2946-3-33
- Pettersen EF, Goddard TD, Huang CC, et al. UCSF Chimera – a visualization system for exploratory research and analysis. J Comput Chem 2004;25:1605–12
- Ellman GL, Courtney KD, Andres V, Featherstone RM. A new and rapid colorimetric determination of acetylcholinesterase activity. Biochem Pharmacol 1961;7:88–95
- Malterud KE, Rydland KM. Inhibitors of 15-lipoxygenase from orange peel. J Agric Food Chem 2000;48:5576–80
- Hardej D, Ashby Jr CR, Khadtare NS, et al. The synthesis of phenylalanine-derived C5-substituted rhodanines and their activity against selected methicillin-resistant Staphylococcus aureus (MRSA) strains. Eur J Med Chem 2010;45:5827–32
- Dolezel J, Hirsova P, Opletalova V, et al. Rhodanineacetic acid derivatives as potential drugs: preparation, hydrophobic properties and antifungal activity of (5-arylalkylidene-4-oxo-2-thioxo-1,3-thiazolidin-3-yl)acetic acids. Molecules 2009;14:4197–212