Abstract
Beta-carbonic anhydrases (β-CAs) have been recently reported to be present in many protozoan and metazoan species, whereas it is absent in mammals. In this review, we introduce β-CA from Drosophila melanogaster as a model enzyme for pesticide development. These enzymes can be targeted with various enzyme inhibitors, which can have deleterious effects on pathogenic and other harmful organisms. Therefore, β-CAs represent a new potential target to fight against Dipteran vectors and pests relevant to medicine, veterinary medicine, and agriculture.
Introduction
Drosophila melanogaster, the common fruit fly, has been used as a model organism in scientific research for over a century. Early studies of D. melanogaster by Thomas Hunt Morgan (1866–1945) and his students at Columbia University led to great discoveries, such as sex-linked inheritance and the mutational effects of ionizing radiation on genesCitation1. D. melanogaster was the first major complex organism to have its genome sequencedCitation2. The vast majority of mammalian genes with orthologs in D. melanogaster have been found to be essential for normal mammalian development and function. The observed homologies between the genomes of D. melanogaster and human strengthened the role of D. melanogaster as a model to understand human biology and disease processes. The D. melanogaster genome encodes more than 14 000 genes on four chromosomes, three of which carry the bulk of genetic materialCitation3. It has been estimated that nearly 75% of disease-related genes in humans have functional orthologs in the flyCitation4. Overall identity between fly and mammal homologs, at the nucleotide or protein sequence level, is usually approximately 40%; however, in conserved functional domains, it can be 80 to 90%, or higherCitation5. Multiple features of D. melanogaster make it particularly valuable as a model organism. It has proven to be an excellent model for studying human disease as many medicines effective in D. melanogaster have translated to usage in humansCitation6. The fly has a very rapid life cycle; a single fertile mating pair can produce hundreds of genetically identical offspring within 10 to 12 days. This is in contrast to traditional rodent models, in which only a few offsprings are produced every 3–4 monthsCitation5.
The conservation of human disease genes in Drosophila melanogaster allows for functional analysis of orthologs implicated in human aging and age-related diseases. D. melanogaster models have been developed for a variety of age-related processes and disorders, including stem-cell decline and cardiovascular deteriorationCitation7. In addition to classical and molecular genetics, D. melanogaster is widely used to investigate problems requiring a multidisciplinary approach, such as those involving developmental biology and neurobiologyCitation8. Its complex nervous system, conserved neurological functions, and human disease-related loci allow D. melanogaster to be an ideal model organism in the study of neurodegenerative diseases. Today, it is aiding research in diseases such as Alzheimer's and Parkinson's, which are becoming more prevalent in today's ageing populationCitation1. The D. melanogaster genome encodes many mitochondrial proteins, which have been intensively studied in a number of genetic diseases associated involving mitochondrial dysfunctionsCitation9.
The use of D. melanogaster has not been limited to genetic research. It has been proven useful for pharmacological investigations. Many drug-induced effects, first detected in D. melanogaster, have been validated in mammalsCitation10,Citation11. Drosophila melanogaster is used to dissect host interactions with known insect pathogens and is a tractable model system for human disease. Studies with insect pathogens provide important data on the pathology of infectionCitation6. Drosophila melanogaster has been used as a model system in nutrition and food industries to evaluate potential health benefits of organic foodsCitation12. Recombinant acetylcholinesterase (AChE) originating from D. melanogaster (DmAChE) can be used for detecting organophosphate and carbamate insecticides in food, vegetables, and environmentCitation13. Recombinant D. melanogaster AChE (R-DmAChE), multiwalled carbon nanotubes, and Prussian blue have been combined for the development of a three-electrode biosensor, as a new disposable screen-printed electrode, for rapid detection of organophosphate and carbamate pesticides in vegetable and water samplesCitation14. The mutagenic actions of various chemicals, such as alkylating agents, were tested on D. melanogaster to evaluate unfavorable effects after several generationsCitation15. The toxicity and mutagenicity of 1,2,4,5-tetrachlorobenzene, 1,4,5-trichloro-2,6-nitrobenzene, pentachloronitrobenzene, methyl-l-(butyl-carbamoyl)-2-benzimidazole carbamate fungicides, and dimethyl-2,3,5,6-tetrachloro-terephthalate herbicide have been studied using a Drosophila modelCitation16. Moreover, D. melanogaster has been applied in resistance studies focusing on cyclodiene and phenylpyrazole insecticidesCitation17. Adverse effects of endosulfan manifested both at cellular and organismal levels in D. melanogaster. All these studies have evidenced that D. melanogaster represents a great alternative animal model for screening the risk caused by environmental chemicalsCitation18. It is important to remember that D. melanogaster is not a perfect representative model. However, there are bulks of conserved biology between D. melanogaster and human, and thus, these similarities can be exploited in the drug discovery process. There are potential differences in the pharmacokinetics and pharmacodynamics of small molecules, which may produce significant discrepancies in drug levels and tissue distribution profiles between mammals and D. melanogasterCitation5. Notably, there may be blood-brain permeability differences, which affect the results in the development of drugs targeted to the central nervous systemCitation19. Differences in toxicity represent another very important issue that needs to be taken into account. Because of metabolic differences, some drugs may be toxic in flies, whereas they are not toxic in humans and vice versaCitation20. Because of these factors, it is emphasized that D. melanogaster can be used only as a screening platform for target discovery, primary small-molecule screening, or post-screening validation to narrow down a large pool of potential drug candidates to a much smaller pool of lead compounds that will be validated using traditional mammalian modelsCitation5. Taken together, in spite of certain limitations, D. melanogaster is a great scientific tool for drug, pesticide, and target discovery processes.
Beta carbonic anhydrase
Carbonic anhydrases (CAs) are ubiquitous metalloenzymes. The active site of CAs contain a zinc ion (Zn2+) which has a critical role in the catalytic activity of the enzymeCitation21. CAs catalyze the reversible hydration of carbon dioxide according to the following reactionCitation22: ().
CAs are encoded by five evolutionary divergent gene families and the corresponding enzymes are designated α, β, γ, δ, and ζ-CAs. α-CAs are present in animals, some fungi, bacteria, algae, and cytoplasm of green plants . In total, 13 enzymatically active α-CAs have been reported in mammals: CA I, CA II, CA III, CA VII, and CA XIII are cytosolic enzymes; CA IV, CA IX, CA XII, CA XIV, and CA XV are membrane bound; CA VA and CA VB are mitochondrial; and CA VI is secreted. CA VIII, CA X, and CA XI are acatalytic CA-related proteinsCitation23. β-CAs are expressed in fungi, bacteria, archaea, algae, chloroplasts of plants, some protozoans, and metazoansCitation22–24. γ-CAs are expressed in plants, archaea, and some bacteria. δ- and ζ-CAs are present in several classes of marine phytoplanktonCitation23. ζ- and γ-CAs represent exceptions to the rule of having zinc ion in the catalytic site, because they can use cadmium (ζ), iron (γ), or cobalt (γ) as cofactorsCitation21,Citation25.
The first β-CA was serendipitously discovered by Neish in 1939Citation26, then it was identified as a chloroplastic CA in spinach (Spinacea oleracea) in 1990Citation27. Many putative β-CAs have been discovered since 1990, not only in photosynthetic organisms, but also in eubacteria, yeast, and archaea. The first bacterial β-CA gene was named CynT and recognized in Escherichia coliCitation28. Since then, β-CA has been identified in a number of other pathogenic bacteriaCitation29. Although β-CAs were initially thought to be expressed only in plants, members of this enzyme family are indeed present in a wide variety of species from bacteria and archaea to invertebrate animals, missing from all vertebrates as well as most chordatesCitation22. The unique distribution among various species makes it an attractive target for evolutionary and targeted drug studiesCitation30.
β-CA is an important accessory enzyme for many CO2 or utilizing enzymes (e.g. RuBisCO in chloroplasts, cyanase in E. coliCitation31, urease in Helicobacter pyloriCitation32, and carboxylases in Corynebacterium glutamicumCitation33). In cyanobacteria, β-CA is an essential component of the CO2-concentrating carboxysome organelleCitation34. β-CA activity is required for growth of E. coli bacteria in air; it is also indispensable if the atmospheric partial pressure of CO2 is high, or during anaerobic growth in a closed vessel at low pH, where copious CO2 is generated endogenouslyCitation35. β-CA is also needed for growth of C. glutamicumCitation33 and some yeasts, such as Saccharomyces cerevisiaeCitation36. In higher plants, the Flaveria bidentis genome contains at least three β-CA genes, named CA1, CA2, and CA3Citation37. The functional roles of β-CAs in plants are not yet fully understood, even though a lot of new data have emerged in recent years. C3 and C4 plants have different mechanisms for carbon fixation and photosynthesis and, thus, β-CAs might perform different roles, depending on the location of the enzyme and the type of plantCitation38. In plants, the highest CA activity has been found within the chloroplast stroma, but there is also some CA activity in the cytosol of mesophyll cellsCitation39. Carbon dioxide coming from the external environment must be rapidly hydrated by β-CA and converted into
for the phosphoenolpyruvate carboxylase enzymeCitation38. Additionally, CAs play a role in photosynthesis by facilitating diffusion into and across the chloroplast, and by catalyzing
dehydration to supply CO2 for RuBisCO. Interestingly, both RuBisCO and β-CA expression levels increase together when Pisum sativum is transferred from an environment with high levels of CO2 to one with low levelsCitation40.
Existing crystal structures of β-CAs reveal that a zinc ion (Zn2+) is ligated by two conserved cysteines and one conserved histidineCitation30. Until now, the only X-ray crystallography structure defined for a plant β-CA belongs to P. sativumCitation41. E. coli was the first bacteria for which a β-CA crystal structure was determinedCitation42. β-CA can adopt a variety of oligomeric states with molecular masses ranging from 45 to 200 kDaCitation24.
In protozoan and metazoan, only few studies have been conducted on β-CAs. The majority of studies are related to cloning, characterization, and functional analysis of α-CAs, including Caenorhabditis elegansCitation43, Ostertagia ostertagi (a nematode and causative agent of Ostertagiosis in goat, sheep, cow, and cattle)Citation44, Plasmodium falciparum (causative agent of malaria)Citation45, Riftia pachyptila (giant tube worm)Citation46, and Trypanosoma cruzi (causative agent of Chagas disease)Citation47. In 2010, two independent studies initiated investigations of β-CAs in protozoans and metazoans. Two genes encoding β-CAs (y116a8c.28 and bca-1) were identified in Caenorhabditis elegansCitation48. Our group reported a novel β-CA gene identified in FlyBase (http://flybase.org/), which was named D. melanogaster (fruit fly) β-CA (DmBCA). DmBCA is an active, dimeric mitochondrial enzyme for the physiological reaction catalyzed by CAs, the hydration of CO2 to bicarbonate and protons. It is inhibited by various inorganic anions, boronic/arsonic acids, and sulfonamides. Recently, the inhibitory effects of sulfamates and sulfonamides on DmBCA were evaluatedCitation49. The analyses revealed that ethoxzolamide and sulfonylated benzenesulfonamides are the best inhibitors of DmBCA (inhibition constants: 65.3–138 nM). Moreover, methazolamide and sulthiame were also identified as effective DmBCA inhibitors (inhibition constants: 237 and 249 nM, respectively). The simple aromatic/heterocyclic sulfonamides (inhibition constants: 0.47–6.40 µM) were weaker inhibitors of DmBCA. Some other known CA inhibitors such as topiramate, zonisamide, and saccharine did not show any significant inhibitory effect on DmBCA.
Mammals do not possess β-CAs, but these enzymes are widespread in organisms of lesser complexity, making them exciting new targets for parasitic drug development. In our first article on this topic, we identified 23 β-CA sequences, including many pathogenic organisms and vectors of the animal kingdomCitation22. Recently, we also reported the details of cloning, characterization, and inhibition studies of a β-CA from Leishmania donovani chagasi (causative agent of Leishmaniasis)Citation50. This enzyme possessed significant catalytic activity for the CO2 hydration reaction with kcat of 9.35 × 105 s−1 and kcat/KM of 5.9 × 107 M−1 s−1. Moreover, a large number of aromatic/heterocyclic sulfonamides and 5-mercapto-1,3,4-thiadiazoles were investigated as inhibitors of β-CA from Leishmania donovani chagasi. Interestingly, some of the tested thiols inhibited the growth of the parasites in vivo. In the last updated analysis, 75 β-CAs were identified from protozoans and metazoans with 52 novel sequences that were not previously annotated as β-CAsCitation24. Many of these CAs are relevant as they are present in infectious disease vectors and pests important in medicine, veterinary medicine, and agriculture. The results of subcellular localization prediction suggested that 31 of the β-CAs are targeted to mitochondria and a single β-CA from Anopheles darlingi was predicted as the first secretory β-CA in the Dipteran species.
Diptera
Diptera (true flies or two-winged) are among the largest radiations of terrestrial eukaryotic organisms. As for other holometabolous insect orders, major diversification of fly lineages occurred in mesozoic environmentsCitation51. Dipteran flies are probably one of the most important insect orders in terms of their impacts on medicine, veterinary medicine, and agriculture (). Moreover, D. melanogaster is an important Dipteran model organism for studies in genetics and developmental biology. Until recently, our knowledge about the phylogenetic relationships of most major fly lineages was very limitedCitation52. Diptera comprises a large order, containing an estimated 240 000 species of mosquitoes, gnats, midges, and others, although under half of these have been described, in more than 100 familiesCitation51. The brachyceran flies represent an enormous mesozoic insect radiation. This group includes many well-known members, such as fruit flies, horse flies, flower flies, blow flies, house flies, and numerous less famous relativesCitation52. Many Dipteran species cause economic damages to plants, animals, or humans through direct feeding by their larvae. Also, many species are known to transmit deadly viral, bacterial, parasitic, and helminthic diseases to human and animals via their blood-feeding adult stageCitation51. The complete list of flies and mosquitoes from Diptera order, important as animal and human disease vectors and agricultural pests, are shown in .
Table 1. The most important flies and mosquitoes (Dipteran) which are relevant to medicine, veterinary medicine, and agriculture.
β-CA from Dipteran species
Taxonomically, Dipteran species belong to the Metazoa kingdom and D. melanogaster is located in the subclass of metazoans that express β-CA enzymesCitation22. In addition to D. melanogaster, we recently identified many β-CAs from other Drosophila species: D. ananassae, D. erecta, D. grimshawi, D. mojavensis, D. persimilis, D. pseudoobscura, D. sechellia, D. simulans, D. virilis, D. willistoni, and D. yakuba; and in some Dipteran mosquitoes such as Aedes aegypti, Anopheles darlingi, Anopheles gambiae, and Culex quinquefasciatusCitation22,Citation24. Most recently, we succeeded in identification of β-CA protein sequences in other Dipteran species, such as Anopheles aquasalis, Ceratitis capitata, and Musca domestica, by UniProt BLAST search (http://www.uniprot.org/) using DmBCA as a query.
A multiple sequence alignment (MSA) analysis of β-CA protein sequences from defined Dipteran species was made utilizing the Clustal Omega algorithmCitation53 within Jalview program (version 2.8.ob1) (http://www.jalview.org/)Citation54. This analysis revealed that there are highly identical motifs in all defined Dipteran species, especially in their two highly conserved active sites, CXDXR (C = cysteine, D = aspartic acid, R = arginine, X = any residue) and HXXC (H = histidine, C = cysteine, X = any residue), which are shown in .
Figure 1. Multiple sequence alignment of β-CA protein sequences from defined Dipteran species. First highly conserved sequence (CXDXR) and second highly conserved sequence (HXXC) for β-CAs from Dipteran species are indicated with two arrows at the bottom of the figure.
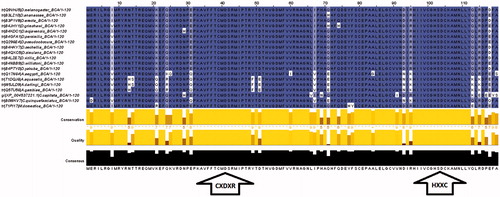
Moreover, previous results on the subcellular localization using the TargetP webserver (http://www.cbs.dtu.dk/services/TargetP/)Citation55 showed that all β-CAs from Dipteran species are predicted as mitochondrial enzymes, except for Anopheles darlingi which may be a secretory enzymeCitation24. Furthermore, prediction of the subcellular localization of β-CAs from Anopheles aquasalis, Ceratitis capitata, and Musca domestica suggested that these enzymes are mitochondrial as well. Prediction results of the subcellular localization of β-CAs from defined Dipteran species are shown in .
Table 2. Prediction of the subcellular localization of β-CAs from defined Dipteran species.
There are more than 240 000 Dipteran species and information of their β-CAs is still unknown in all but a handful of cases. However, our results have indicated that all Dipteran species studied to date have at least one β-CA encoding gene in their genomes. Many Dipteran species are potential invasive vectors or pests, which cause enormous economic losses in agriculture, horticulture, and beekeeping as well as severe viral, bacterial, parasitic, and helminthic diseases in humans and other wild and domestic animal species (Zolfaghari Emameh et al., 2014, submitted), such as Chrysomya bezziana which is one of the causative agents of oralCitation56 and cutaneous myiasisCitation57 ( and ). Meanwhile, β-CAs can be targeted and inhibited, even though the exact sequence data are not yet available for some Dipteran species. So far, our results indicate that Dipteran β-CAs are highly homologous and thus, fairly similar inhibition profiles can be predicted (, ). Thus, the results from D. melanogaster studies can be generalized to other Dipterans with high probability.
Figure 2. Oral myiasis by Chrysomya bezziana. (A) Appearance of the patient before maxillary surgery, (B) maggots in the maxillary anterior region, and (C) removal of maggots with tweezers. Figure adopted with publisher’s permission from Ref. Citation56.
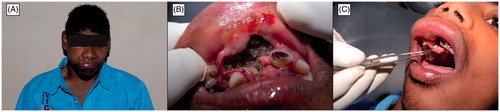
Figure 3. Cutaneous myasis caused by Chrysomya bezziana. (A) Cutaneous myiasis ulcer on right leg of patient, (B) Isolated Chrysomya bezziana larvae from ulcer of a patient. Figure adopted with publisher’s permission from Ref. Citation57.
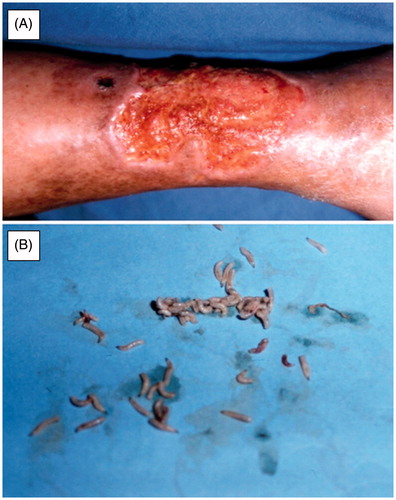
describes eight categories of known β-CA inhibitors, which are able to inhibit catalytic activity of this enzyme familyCitation58,Citation59. As the result, some biochemical pathways in Dipteran species such as gluconeogenesis, nucleotide biosynthesis, fatty acid synthesis, gastrointestinal function, neuronal signaling, respiration, and reproduction can be targeted by inhibition of the β-CA activity. It is known that β-CAs are required for CO2 sequestration within chloroplasts in plants and algae, and therefore CA inhibition may affect the rate of photosynthesis as a potential adverse effectCitation60. Importantly, β-CA inhibition in fungi and D. melanogaster revealed completely different inhibition profilesCitation22, suggesting that β-CAs of parasites and insects can be inhibited with higher affinity than plant CAs by applying the right inhibitors and concentrationsCitation24.
Figure 4. Role of β-CA in cellular biochemical pathways of Dipteran species and effects of β-CA inhibitors on them. Cellular biochemical pathways in Dipteran species are shown in yellow colored rectangles, except photosynthesis in plant and algae which is shown in green colored rectangle. Known β-CA inhibitors are shown in red colored shapes.
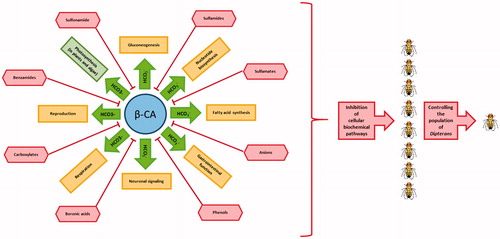
Conclusion
As an overall conclusion, the identity between β-CAs from D. melanogaster and other Dipteran species supports β-CA from D. melanogaster as a model enzyme for molecular and inhibition studies. The absence of β-CA enzymes in humans and other animal species suggests that the possible off-target effects on human and other vertebrate animals would be minimal.
Declaration of interest
The authors declare that they have no competing interests.
To perform these studies, RZE received a scholarship support from the Ministry of Science, Research and Technology, and National Institute of Genetic Engineering and Biotechnology of Islamic Republic of Iran. This study was also funded by Finnish Cultural Foundation (HB), Sigrid Juselius Foundation (SP), Jane and Aatos Erkko Foundation (SP), and Competitive Research Funding of the Tampere University Hospital (SP).
Authors' contributions
All authors participated in the design of the study. RZE carried out the bioinformatics searches including blast searches, MSA and prediction of subcellular localization. RZE prepared the first version of the manuscript. All authors participated in writing further versions and read and approved the final manuscript.
References
- Stephenson R, Metcalfe NH. Drosophila melanogaster: a fly through its history and current use. J Roy Coll Phys Edinburgh 2013;43:70–5
- Adams MD, Celniker SE, Holt RA, et al. The genome sequence of Drosophila melanogaster. Science 2000;287:2185–95
- Reiter LT, Potocki L, Chien S, et al. A systematic analysis of human disease-associated gene sequences in Drosophila melanogaster. Genome Res 2001;11:1114–25
- Lloyd TE, Taylor JP. Flightless flies: Drosophila models of neuromuscular disease. Ann N Y Acad Sci 2010;1184:e1–20
- Pandey UB, Nichols CD. Human disease models in Drosophila melanogaster and the role of the fly in therapeutic drug discovery. Pharmacol Rev 2011;63:411–36
- Dionne MS, Schneider DS. Models of infectious diseases in the fruit fly Drosophila melanogaster. Dis Models Mech 2008;1:43–9
- Brandt A, Vilcinskas A. The fruit fly Drosophila melanogaster as a model for aging research. Adv Biochem Eng Biotechnol 2013;135:63–77
- Rubin GM. Drosophila melanogaster as an experimental organism. Science 1988;240:1453–9
- Sardiello M, Licciulli F, Catalano D, et al. MitoDrome: a database of Drosophila melanogaster nuclear genes encoding proteins targeted to the mitochondrion. Nucleic Acids Res 2003;31:322–4
- Kaun KR, Devineni AV, Heberlein U. Drosophila melanogaster as a model to study drug addiction. Hum Genet 2012;131:959–75
- Heberlein U, Tsai LT, Kapfhamer D, Lasek AW. Drosophila, a genetic model system to study cocaine-related behaviors: a review with focus on LIM-only proteins. Neuropharmacology 2009;56:97–106
- Chhabra R, Kolli S, Bauer JH. Organically grown food provides health benefits to Drosophila melanogaster. PLoS One 2013;8:e52988
- Li J, Qian B, Yin J, et al. Surface display of recombinant Drosophila melanogaster acetylcholinesterase for detection of organic phosphorus and carbamate pesticides. PLoS One 2013;8:e72986
- Tang Z, Chen H, Song S, et al. Disposable screen-printed electrode coupled with recombinant Drosophila melanogaster acetylcholinesterase and multiwalled carbon nanotubes for rapid detection of pesticides. J AOAC Int 2011;94:307–12
- Obe G, Sperling K, Belitz HJ. Some aspects of chemical mutagenesis in man and in Drosophila. Ang Chem 1971;10:302–14
- Paradi E, Lovenyak M. Studies on genetical effect of pesticides in Drosophila melanogaster. Acta Biol Acad Sci Hungar 1981;32:119–21
- Remnant EJ, Good RT, Schmidt JM, et al. Gene duplication in the major insecticide target site, Rdl, in Drosophila melanogaster. Proc Natl Acad Sci USA 2013;110:14705–10
- Sharma A, Mishra M, Shukla AK, et al. Organochlorine pesticide, endosulfan induced cellular and organismal response in Drosophila melanogaster. J Hazard Mater 2012;221-222:275–87
- Mayer F, Mayer N, Chinn L, et al. Evolutionary conservation of vertebrate blood-brain barrier chemoprotective mechanisms in Drosophila. J Neurosci 2009;29:3538–50
- Rand MD. Drosophotoxicology: the growing potential for Drosophila in neurotoxicology. Neurotoxicol Teratol 2010;32:74–83
- Ferry JG. The gamma class of carbonic anhydrases. Biochim Biophys Acta 2010;1804:374–81
- Syrjanen L, Tolvanen M, Hilvo M, et al. Characterization of the first beta-class carbonic anhydrase from an arthropod (Drosophila melanogaster) and phylogenetic analysis of beta-class carbonic anhydrases in invertebrates. BMC Biochem 2010;11:28
- Supuran CT. Carbonic anhydrases: novel therapeutic applications for inhibitors and activators. Nat Rev Drug Discov 2008;7:168–81
- Zolfaghari Emameh R, Barker H, Tolvanen ME, et al. Bioinformatic analysis of beta carbonic anhydrase sequences from protozoans and metazoans. Parasit Vectors 2014;7:38
- Lane TW, Saito MA, George GN, et al. Biochemistry: a cadmium enzyme from a marine diatom. Nature 2005;435:42
- Neish AC. Studies on chloroplasts: their chemical composition and the distribution of certain metabolites between the chloroplasts and the remainder of the leaf. Biochem J 1939;33:300–8
- Burnell JN, Gibbs MJ, Mason JG. Spinach chloroplastic carbonic anhydrase: nucleotide sequence analysis of cDNA. Plant Physiol 1990;92:37–40
- Guilloton MB, Korte JJ, Lamblin AF, et al. Carbonic anhydrase in Escherichia coli. A product of the cyn operon. J Biol Chem 1992;267:3731–4
- Supuran CT. Bacterial carbonic anhydrases as drug targets: toward novel antibiotics? Front Pharmacol 2011;2:34
- Tripp BC, Smith K, Ferry JG. Carbonic anhydrase: new insights for an ancient enzyme. J Biol Chem 2001;276:48615–18
- Guilloton MB, Lamblin AF, Kozliak EI, et al. A physiological role for cyanate-induced carbonic anhydrase in Escherichia coli. J Bacteriol 1993;175:1443–51
- Nishimori I, Onishi S, Takeuchi H, Supuran CT. The alpha and beta classes carbonic anhydrases from Helicobacter pylori as novel drug targets. Curr Pharm Des 2008;14:622–30
- Mitsuhashi S, Ohnishi J, Hayashi M, Ikeda M. A gene homologous to beta-type carbonic anhydrase is essential for the growth of Corynebacterium glutamicum under atmospheric conditions. Appl Microbiol Biotechnol 2004;63:592–601
- Fukuzawa H, Suzuki E, Komukai Y, Miyachi S. A gene homologous to chloroplast carbonic anhydrase (icfA) is essential to photosynthetic carbon dioxide fixation by Synechococcus PCC7942. Proc Natl Acad Sci USA 1992;89:4437–41
- Merlin C, Masters M, McAteer S, Coulson A. Why is carbonic anhydrase essential to Escherichia coli? J Bacteriol 2003;185:6415–24
- Mitsuhashi S, Mizushima T, Yamashita E, et al. X-ray structure of beta-carbonic anhydrase from the red alga, Porphyridium purpureum, reveals a novel catalytic site for CO(2) hydration. J Biol Chem 2000;275:5521–6
- Tetu SG, Tanz SK, Vella N, et al. The Flaveria bidentis beta-carbonic anhydrase gene family encodes cytosolic and chloroplastic isoforms demonstrating distinct organ-specific expression patterns. Plant Physiol 2007;144:1316–27
- Ludwig M. The molecular evolution of beta-carbonic anhydrase in Flaveria. J Exp Bot 2011;62:3071–81
- Zabaleta E, Martin MV, Braun HP. A basal carbon concentrating mechanism in plants? Plant Sci 2012;187:97–104
- Majeau N, Coleman JR. Effect of CO2 concentration on carbonic anhydrase and ribulose-1,5-bisphosphate carboxylase/oxygenase expression in pea. Plant Physiol 1996;112:569–74
- Kimber MS, Pai EF. The active site architecture of Pisum sativum beta-carbonic anhydrase is a mirror image of that of alpha-carbonic anhydrases. EMBO J 2000;19:1407–18
- Cronk JD, Endrizzi JA, Cronk MR, et al. Crystal structure of E. coli beta-carbonic anhydrase, an enzyme with an unusual pH-dependent activity. Protein Sci 2001;10:911–22
- Fasseas MK, Tsikou D, Flemetakis E, Katinakis P. Molecular and biochemical analysis of the alpha class carbonic anhydrases in Caenorhabditis elegans. Mol Biol Rep 2011;38:1777–85
- DeRosa AA, Chirgwin SR, Williams JC, Klei TR. Isolation and characterization of a gene encoding carbonic anhydrase from Ostertagia ostertagi and quantitative measurement of expression during in vivo exsheathment. Vet Parasitol 2008;154:58–66
- Krungkrai SR, Suraveratum N, Rochanakij S, Krungkrai J. Characterisation of carbonic anhydrase in Plasmodium falciparum. Intern J Parasitol 2001;31:661–8
- De Cian MC, Andersen AC, Bailly X, Lallier FH. Expression and localization of carbonic anhydrase and ATPases in the symbiotic tubeworm Riftia pachyptila. J Exp Biol 2003;206:399–409
- Pan P, Vermelho AB, Capaci Rodrigues G, et al. Cloning, characterization, and sulfonamide and thiol inhibition studies of an alpha-carbonic anhydrase from Trypanosoma cruzi, the causative agent of Chagas disease. J Med Chem 2013;56:1761–71
- Fasseas MK, Tsikou D, Flemetakis E, Katinakis P. Molecular and biochemical analysis of the beta class carbonic anhydrases in Caenorhabditis elegans. Mol Biol Rep 2010;37:2941–50
- Syrjanen L, Parkkila S, Scozzafava A, Supuran CT. Sulfonamide inhibition studies of the beta carbonic anhydrase from Drosophila melanogaster. Bioorg Med Chem Lett 2014;24:2797–801
- Syrjanen L, Vermelho AB, Rodrigues Ide A, et al. Cloning, characterization, and inhibition studies of a beta-carbonic anhydrase from Leishmania donovani chagasi, the protozoan parasite responsible for leishmaniasis. J Med Chem 2013;56:7372–81
- Yeates DK, Wiegmann BM. Congruence and controversy: toward a higher-level phylogeny of Diptera. Annu Rev Entomol 1999;44:397–428
- Wiegmann BM, Yeates DK, Thorne JL, Kishino H. Time flies, a new molecular time-scale for brachyceran fly evolution without a clock. Syst Biol 2003;52:745–56
- Sievers F, Wilm A, Dineen D, et al. Fast, scalable generation of high-quality protein multiple sequence alignments using Clustal Omega. Mol Syst Biol 2011;7:539
- Waterhouse AM, Procter JB, Martin DM, et al. Jalview Version 2 – a multiple sequence alignment editor and analysis workbench. Bioinformatics 2009;25:1189–91
- Emanuelsson O, Nielsen H, Brunak S, von Heijne G. Predicting subcellular localization of proteins based on their N-terminal amino acid sequence. J Mol Biol 2000;300:1005–16
- Sankari LS, Ramakrishnan K. Oral myiasis caused by Chrysomya bezziana. JOMFP 2010;14:16–8
- Romero-Cabello R, Calderon-Romero L, Sanchez-Vega JT, et al. Cutaneous myiasis caused by Chrysomya bezziana larvae, Mexico. Emerg Infect Dis 2010;16:2014–15
- Supuran CT. Carbonic anhydrase inhibition/activation: trip of a scientist around the world in the search of novel chemotypes and drug targets. Curr Pharmaceut Design 2010;16:3233–45
- McKenna R, Supuran CT. Carbonic anhydrase inhibitors drug design. Sub-cellular Biochem 2014;75:291–323
- Badger M. The roles of carbonic anhydrases in photosynthetic CO(2) concentrating mechanisms. Photosynth Res 2003;77:83–94
- Gubler DJ. The changing epidemiology of yellow fever and dengue, 1900 to 2003: full circle? Compar Immunol Microbiol Infect Dis 2004;27:319–30
- Tiawsirisup S, Kinley JR, Tucker BJ, et al. Vector competence of Aedes vexans (Diptera: Culicidae) for West Nile virus and potential as an enzootic vector. J Med Entomol 2008;45:452–7
- Hay SI, Sinka ME, Okara RM, et al. Developing global maps of the dominant anopheles vectors of human malaria. PLoS Med 2010;7:e1000209
- Krinsky WL. Animal disease agents transmitted by horse flies and deer flies (Diptera: Tabanidae). J Med Entomol 1976;13:225–75
- Lindsay SW, Lindsay TC, Duprez J, et al. Chrysomya putoria, a putative vector of diarrheal diseases. PLoS Negl Trop Dis 2012;6:e1895
- Vale DS, Cavalieri I, Araujo MM, et al. Myiasis in palate by Cochliomyia hominivorax. J Craniofac Surg 2011;22:e57–9
- Andreadis TG. The contribution of Culex pipiens complex mosquitoes to transmission and persistence of West Nile virus in North America. J Am Mosq Control Assoc 2012;28:137–51
- Linthicum KJ. Introduction to the symposium Global Perspective on the Culex pipiens Complex in the 21st century: The Interrelationship of Culex pipiens, quinquefasciatus, molestus and others. J Am Mosq Control Assoc 2012;28:4–9
- Johnson N, Voller K, Phipps LP, et al. Rapid molecular detection methods for arboviruses of livestock of importance to northern Europe. J Biomed Biotechnol 2012;2012:719402
- Yu CY, Wang JS. Culicoides arakawae (Diptera: Ceratopogonidae) efficiently blood-fed and infected with Leucocytozoon caulleryi through a natural membrane. Vet Parasitol. 2001;99:297–303
- Lowrie RC, Jr Raccurt CP. Assessment of Culicoides barbosai as a vector of Mansonella ozzardi in Haiti. Am J Trop Med Hyg 1984;33:1275–7
- Veronesi E, Henstock M, Gubbins S, et al. Implicating Culicoides biting midges as vectors of Schmallenberg virus using semi-quantitative RT-PCR. PLoS One 2013;8:e57747
- Aybar CA, Juri MJ, Santana M, et al. The spatio-temporal distribution patterns of biting midges of the genus Culicoides in Salta province, Argentina. J Insect Sci 2012;12:145
- Meyer CL, Bennett GF. Observations on the sporogony of Plasmodium circumflexum Kikuth and Plasmodium polare Manwell in New Brunswick. Can J Zoo 1976;54:133–41
- Glaizot O, Fumagalli L, Iritano K, et al. High prevalence and lineage diversity of avian malaria in wild populations of great tits (Parus major) and mosquitoes (Culex pipiens). PLoS One 2012;7:e34964
- Hu JM, Wang CC, Chao LL, et al. First report of furuncular myiasis caused by the larva of botfly, Dermatobia hominis, in a Taiwanese traveler. Asian Pac J Trop Biomed 2013;3:229–31
- Francesconi F, Lupi O. Myiasis. Clin Microbiol Rev 2012;25:79–105
- Ravel S, de Meeus T, Dujardin JP, et al. The tsetse fly Glossina palpalis palpalis is composed of several genetically differentiated small populations in the sleeping sickness focus of Bonon, Cote d'Ivoire. Infect Genet Evol 2007;7:116–25
- Cardoso Jda C, de Almeida MA, dos Santos E, et al. Yellow fever virus in Haemagogus leucocelaenus and Aedes serratus mosquitoes, southern Brazil, 2008. Emerg Infect Dis 2010;16:1918–24
- Desquesnes M, Holzmuller P, Lai DH, et al. Trypanosoma evansi and surra: a review and perspectives on origin, history, distribution, taxonomy, morphology, hosts, and pathogenic effects. BioMed Res Intern 2013;2013:194176
- Langley PA. Pathogen transmission in relation to feeding and digestion by haematophagous Arthropods. Acta Trop 1975;32:116–24
- Dusbabek F, Soukupova A, Gregor F, Krejci J. The role of Hydrotaea armipes Fall. (Diptera, Muscidae) in the transmission of infectious bovine keratoconjunctivitis. Folia Parasitol 1982;29:79–83
- Hillerton JE, Bramley AJ, Thomas G. The role of Hydrotaea irritans in the transmission of summer mastitis. Br Veterin J 1990;146:147–56
- Hassan MU, Khan MN, Abubakar M, et al. Bovine hypodermosis – a global aspect. Trop Anim Health Prod 2010;42:1615–25
- Brenner RJ, Wargo MJ. Observations on adult bionomics and larval ecology of Leptoconops torrens (Diptera: Ceratopogonidae) during an outbreak in the Coachella Valley of southern California, USA. J Med Entomol 1984;21:460–9
- Calzada JE, Saldana A, Rigg C, et al. Changes in phlebotomine sand fly species composition following insecticide thermal fogging in a rural setting of Western panama. PLoS One 2013;8:e53289
- Bech-Nielsen S, Sjogren U, Lundquist H. Parafilaria bovicola (Tubangui 1934) in cattle: epizootiology-disease occurrence. Am J Veterin Res 1982;43:945–7
- Graczyk TK, Knight R, Tamang L. Mechanical transmission of human protozoan parasites by insects. Clin Microbiol Rev 2005;18:128–32
- Vriesekoop F, Shaw R. The Australian bush fly (Musca vetustissima) as a potential vector in the transmission of foodborne pathogens at outdoor eateries. Foodborne Pathogens Dis 2010;7:275–9
- Penner LR, Melnick JL. Methods for following the fate of infectious agents fed to single flies. J Experim Med 1952;96:273–80
- Zapata S, Mejia L, Le Pont F, et al. A study of a population of Nyssomyia trapidoi (Diptera: Psychodidae) caught on the Pacific coast of Ecuador. Parasit Vectors 2012;5:144
- Andreadis TG, Anderson JF, Vossbrinck CR, Main AJ. Epidemiology of West Nile virus in Connecticut: a five-year analysis of mosquito data 1999-2003. Vector Borne Zoonotic Dis 2004;4:360–78
- Barker CM, Paulson SL, Cantrell S, Davis BS. Habitat preferences and phenology of Ochlerotatus triseriatus and Aedes albopictus (Diptera: Culicidae) in southwestern Virginia. J Med Entomol 2003;40:403–10
- Azeredo-Espin AM, Madeira NG. Primary myiasis in dog caused by Phaenicia eximia (Diptera:Calliphoridae) and preliminary mitochondrial DNA analysis of the species in Brazil. J Med Entomol 1996;33:839–43
- Mavale MS, Fulmali PV, Ghodke YS, et al. Experimental transmission of Chandipura virus by Phlebotomus argentipes (diptera: psychodidae). Am J Trop Med Hyg 2007;76:307–9
- Jacob BG, Novak RJ, Toe LD, et al. Validation of a remote sensing model to identify Simulium damnosum s.l. breeding sites in Sub-Saharan Africa. PLoS Negl Trop Dis 2013;7:e2342
- Chansang U, Mulla MS, Chantaroj S, Sawanpanyalert P. The eye fly Siphunculina funicola (Diptera: Chloropidae) as a carrier of pathogenic bacteria in Thailand. Southeast Asian J Trop Med Public Health 2010;41:61–71
- Hornok S, Foldvari G, Elek V, et al. Molecular identification of Anaplasma marginale and rickettsial endosymbionts in blood-sucking flies (Diptera: Tabanidae, Muscidae) and hard ticks (Acari: Ixodidae). Vet Parasitol 2008;154:354–9
- Aluja M, Ordano M, Guillen L, Rull J. Understanding long-term fruit fly (Diptera: Tephritidae) population dynamics: implications for areawide management. J Econ Entomol 2012;105:823–36
- Core A, Runckel C, Ivers J, et al. A new threat to honey bees, the parasitic phorid fly Apocephalus borealis. PLoS One 2012;7:e29639
- Jiang F, Li ZH, Deng YL, et al. Rapid diagnosis of the economically important fruit fly, Bactrocera correcta (Diptera: Tephritidae) based on a species-specific barcoding cytochrome oxidase I marker. Bull Entomol Res 2013;103:363–71
- Karsten M, van Vuuren BJ, Barnaud A, Terblanche JS. Population genetics of Ceratitis capitata in South Africa: implications for dispersal and pest management. PLoS One 2013;8:e54281
- Wharton RA, Trostle MK, Messing RH, et al. Parasitoids of medfly, Ceratitis capitata, and related tephritids in Kenyan coffee: a predominantly koinobiont assemblage. Bull Entomol Res 2000;90:517–26
- Drosopoulou E, Nestel D, Nakou I, et al. Cytogenetic analysis of the Ethiopian fruit fly Dacus ciliatus (Diptera: Tephritidae). Genetica 2011;139:723–32
- Lee JC, Bruck DJ, Curry H, et al. The susceptibility of small fruits and cherries to the spotted-wing drosophila, Drosophila suzukii. Pest Manag Sci 2011;67:1358–67
- Wharton R, Ward L, Miko I. New neotropical species of Opiinae (Hymenoptera, Braconidae) reared from fruit-infesting and leaf-mining Tephritidae (Diptera) with comments on the Diachasmimorpha mexicana species group and the genera Lorenzopius and Tubiformopius. ZooKeys 2012:27–82
- Strikis PC, Marsaro Junior AL, Adaime R, Lima CR. First report of infestation of cassava fruit, Manihot esculenta, by Neosilba perezi (Romero & Ruppell) (Lonchaeidae) in Brazil. Braz J Biol 2012;72:631–2
- Thibout E, Pierre D, Mondy N, et al. Host-plant finding by the asparagus fly, Plioreocepta poeciloptera (Diptera: Tephritidae), a monophagous, monovoltine tephritid. Bull Entomol Res 2005;95:393–9
- Cossentine J, Thistlewood H, Goettel M, Jaronski S. Susceptibility of preimaginal western cherry fruit fly, Rhagoletis indifferens (Diptera: Tephritidae) to Beauveria bassiana (Balsamo) Vuillemin clavicipitaceae (Hypocreales). J Invertebr Pathol 2010;104:105–9
- Castrejon-Gomez VR, Aluja M, Arzuffi R, Villa P. Two low-cost food attractants for capturing Toxotrypana curvicauda (Diptera: Tephritidae) in the field. J Econ Entomol 2004;97:310–15
- Boucher TJ, Ashley R, Durgy R, et al. Managing the pepper maggot (Diptera: Tephritidae) using perimeter trap cropping. J Econ Entomol 2003;96:420–32