Abstract
In an effort to develop safe and potent anti-inflammatory agents, a series of novel 4′-fluoro-2′-hydroxychalcones 5a–d and their dihydropyrazole derivatives 6a–d was prepared. It was synthesized via aldol condensation of 4′-fluoro-2′-hydroxyacetophenone with appropriately substituted aldehydes followed by cyclization with hydrazine hydrate. All the synthesized compounds were evaluated for their antioxidant, anti-inflammatory, cyclooxygenase inhibition selectivity and analgesic activities. The dimethoxychalcone 5a and its dihydropyrazole derivative 6a showed the highest antioxidant activity, while the monomethoxychalcone 5d and its dihydropyrazole derivative 6d showed the highest analgesic and anti-inflammatory activities. It was also found that there is a close correlation between 4′-fluoro-2′-hydroxychalcones 5a–d and their dihydropyrazole derivatives 6a–d in the screened biological activities. To explain the correlation between the synthesized chalcones and their dihydropyrazole derivatives, especially for the anti-inflammatory activity, docking studies were performed.
Introduction
Chalcones are precursors of flavonoid compounds, which are present in many edible plants and display interesting biological activities including antioxidantCitation1, antibacterialCitation2, anti-inflammatoryCitation3–5 and cytotoxicCitation4–6 activities. Chemically, chalcones consist of two aromatic rings joined by a three carbon α, β-unsaturated carbonyl system (structure, ). It was reported that 2-hydroxyl substitution on A ring is critical for both antioxidantCitation7 and anti-inflammatoryCitation8 activities of chalcones. In addition, Padhye et al.Citation9 observed that fluorination of chalcones enhances the biological activities as shown in the reported fluorinated 2′-hydroxychalcones (1). In addition, promising anti-inflammatory and antioxidant activities of 2,4-dimethoxy and 3,4,5-trimethoxy chalcones (2) were obtainedCitation10. Cyclization of the chalcones with hydrazine hydrate will yield the corresponding dihydropyrazole derivatives, which retain the geometry of the two aromatic rings separated with three bonds like chalcones. It is expected that these dihydropyrazole derivatives will maintain the same biological activities of the corresponding open chalcones. Based on these observations, we now describe the synthesis and antioxidant, analgesic and anti-inflammatory activities for a new group of 4′-fluoro-2′-hydroxychalcones 5a–d and their cyclized 3,5-diaryl-4,5-dihydro-1H-pyrazoles 6a–d in which (i) hydroxyl group at 2-position of A ring, (ii) fluoro substituent at 4-position of the same ring, (iii) methoxy, thiometoxy or two methoxy groups at different positions of B ring.
Results and discussion
Chemistry
A group of 3-(4-fluoro-2-hydroxyphenyl)-5-(substitutedphenyl)-4,5-dihydro-1H-pyrazoles (6a–d) was synthesized using the reaction sequence illustrated in Scheme 1. Accordingly, reaction of 4′-flouro-2′-hydroxyacetophenone (3) with different substituted benzaldehydes 4a–d under reflux in ethanol provided the corresponding 4′-flouro-2′-hydroxychalcones (5a–d) in higher yields (74–91%). Cyclization of the chalcones 5a–d with hydrazine hydrate in ethanol gave the respective 3,5-diaryl-4,5-dihydropyrazoles (6a–d) in good yields (65–78%). All the prepared compounds have been characterized by infrared (IR), 1HNMR, 13CNMR, mass spectra and elemental analyses.
Scheme 1. Reagents and conditions: (a) EtOH, NaOH, RT and 2–6 h; (b) EtOH, hydrazine hydrate, reflux and 2–4 h.
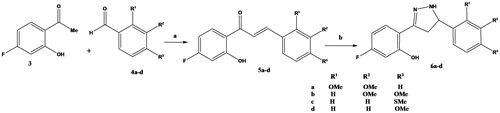
The IR spectra of chalcone compounds 5a–d showed sharp peak at 1632–1641 cm–1 corresponding to C = O, while the 1H NMR spectra of 5a–d displayed two doublets, each of one proton intensity, one at δ 7.43–7.68 and the second at δ 7.90–8.21 with high J value (15.6 Hz) indicating the two olefinic protons. The 1H NMR spectra of dihydropyrazole compounds 6a–d showed three signals as doublet of doublet (dd), each of one proton intensity, one at δ 3.09–3.30, second at δ 3.56–3.67 and the third at δ 4.97–5.23 with three different J values (16.5, 10.2 and 8.7 Hz, respectively) corresponding to three protons of the dihydropyrazole ring. The highest J value was due to geminal coupling of two protons at position four, while the other two J values due to coupling of two geminal protons with proton at position five. In addition, 13C NMR spectra of 5a–d confirmed the presence of α,β-unsaturated carbonyl system of chalcones by presence of three peaks at δ 121.3–125.0, 140.7–145.9 and 192.4–192.5 corresponding to propenone C-2, propenone C-1 and C of C = O, respectively. Furthermore, 13C NMR spectra of 6a–d confirmed the presence of the dihydropyrazole ring due to the presence of three peaks at δ 40.3–40.9, 60.3–60.9 and 155.7–159.6 corresponding to dihydropyrazole C-4, C-5 and C-3, respectively.
Biological evaluation
Antioxidant activity
The antioxidant activities of the all prepared chalcones 5a–d and their cyclized dihydropyrazoles 6a–d were evaluated using 2,2-diphenyl-1-picrylhydrazyl (DPPH) radical scavenging method and yeast-based antioxidant screening assay. The dimethoxychalcone 5a was found to be the most potent antioxidant among all the chalcones 5a–d with the least value of IC50 (190.56 µg/mL) () and the least active among the series being compound 5c with the largest value of IC50 (283.13 µg/mL). Similarly, for dihydropyrazole series 6a–d, the dimethoxy derivative 6a was found to be the most potent antioxidant among all the series with the least value of IC50 (79.8 µg/mL) and the least active among the series being compound 6c with the largest value of IC50 (199.2 µg/mL). Furthermore, the antioxidant activity of test compounds 5a–d and 6a–d, based on their ability to inhibit the H2O2-induced yeast oxidative stress, showed the same order of potency (5a and 6a are the most active derivatives for 5a–d and 6a–d, respectively, while 5c and 6c are the least active derivatives) as shown in .
Figure 2. Antioxidant activity screening in a 96-well microplate high throughput assay using Saccharomyces cerevisiae. Yeast oxidative stress was measured on the basis of survival of yeast cells (yeast growth) after treatment with H2O2.
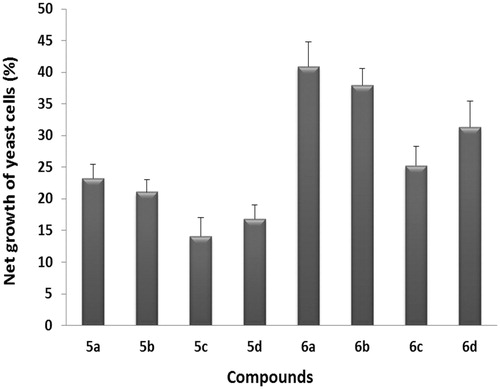
Table 1. Free radical scavenging capacities of the test compounds 5a–d, 6a–d measured by DPPH assay.
Anti-inflammatory activity
In vitro cyclooxygenase (COX) assay revealed the structure–activity relationships of chalcones 5a–d and the corresponding dihydropyrazoles 6a–d and its broad range (moderately potent-to-inactive; ) of inhibitory activity for COX-1 (IC50 = 14.68 to > 30 µM) and COX-2 (IC50 = 0.93–12.35 µM). Within the chalcone series 5a–d, compound 5a was more potent inhibitor of COX-1 than 5d, while 5b and 5c were inactive. On the other hand, all compounds inhibited COX-2 in the following order 5d > 5b > 5c > 5a. For dihydropyrazoles 6a–d, the inhibition of COX-1 was in the following order, 6a > 6b > 6d, while 6c was inactive. Similarly, all compounds 6a–d inhibited COX-2. These results indicated that compounds having R1 = R2 = H and R3 = OMe (5d and 6d) exhibited the highest COX-2 inhibitory activity and selectivity index. For example, 5d had COX-2 IC50 = 1.53 μM and COX-2 S.I. = 12.58), while the most potent one (6d) showed COX-2 IC50 = 0.93 μM and COX-2 S.I. = 18.79 in comparison with the reference drug celecoxib (COX-2 IC50 = 0.43 μM, S.I. = 35.51).
Table 2. In vitro COX-1 and COX-2 inhibition and anti-inflammatory activity of 4′-flouro-2′-hydroxychalcones 5a–d, dihydropyrazoles 6a–d and celecoxib.
In addition, the anti-inflammatory activity of all the prepared chalcones 5a–d and their cyclized dihydropyrazoles 6a–d were evaluated using carrageenan-induced rat paw edema assay. Each compound was administered orally (10 mg/kg) immediately prior to induction of inflammation by carrageenan subcutaneous injection. The anti-inflammatory activity was then calculated based on paw-volume changes at 1, 3 and 6 h after carrageenan injection as listed in .
A comparable study of the anti-inflammatory activity of the test compounds relative to celecoxib as a reference drug at different time intervals indicated that after 1 h, the chalcone series 5a–d showed percentage activity 24–62% and compound 5d > 5b = 5c > 5a in potency, while the dihydropyrazole series 6a–d showed percentage activity 6–64% and compound 6d > 6b > 6c > 6a in potency. After 3 h, the chalcone series 5a–d showed percentage activity 32–62% and compound 5d > 5a > 5c > 5b in potency, while the dihydropyrazole series 6a–d showed percentage activity 10–73% and compound 6d = 6b > 6a > 6c in potency. After 6 h, the chalcone series 5a–d showed percentage activity 19–54% and compound 5d > 5c > 5b > 5a in potency, while the dihydropyrazole series 6a–d showed percentage activity 6–74% and compound 6d > 6b > 6c > 6a in potency. 1-(4-Fluoro-2-hydroxyphenyl)-3-(4-methoxyphenyl)prop-2-en-1-one (5d) displayed good anti-inflammatory activity (54–62%) comparable to that of celecoxib (32–78%) and was the most potent among the four compounds of chalcone series 5a–d at all-time intervals. Furthermore, the corresponding cyclized dihydropyrazole derivative 6d showed excellent anti-inflammatory activity (64–74%) and was the most potent among all compounds of dihydropyrazole series 6a–d at all-time intervals. Similarly, dimethoxychalcone 5b showed considerable activity (30–38%) at the three different time intervals, and its cyclized derivative 6b displayed excellent activity (62–73%) at the same time intervals. These data indicate that (i) presence of methoxy group at 4-position of B ring of chalcones is important for anti-inflammatory activity and (ii) cyclization of chalcones to the corresponding dihydropyrazole analogues maintain or increase the anti-inflammatory activity.
Analgesic activity
Next, the analgesic activity of test compounds was also investigated. It was assessed by two different models: the hot-plate test and acetic acid-induced writhing test. In the hot-plate assay, the latency time on the hotplate was significantly delayed after the administration of tested compounds 5d, 6b, 6c and 6d, compared with basal values, indicating decreased nociception. The percentage increase in the pain threshold for these compounds were 35.2 and 3.52% for compound 5d at 1 and 2 h post-treatment, 30.3 and 20.0% for compound 6b at 1 and 2 h post-drug, 33.5% and 33.7% for compound 6c at 1 and 2 h post-treatment and 68.5% and 50.4% for compound 6d at 1 and 2 h post-treatment. All other compounds showed weak analgesic activity that did not reach statistical significance ().
Table 3. Analgesic effect of test compounds 5a–d, 6a–d compared to piroxicam on thermal pain induced by hot-plate in mice.
Similarly, acetic acid-induced writhing was significantly reduced in mice receiving the compounds 5d, 6b and 6d. The degree of inhibition of the writhing response by these compounds was 27.6%, 31.0% and 54.6%, compared to the vehicle-treated control group. The analgesic activity of the compound 6d was comparable to that of the control drug, piroxicam (62.9%). Other compounds did not exhibit analgesic activity in this test either (). From these results, the analgesic activities of these compounds are clearly parallel to their anti-inflammatory activities.
Figure 3. Analgesic effect of test compounds compared to piroxicam on acetic acid-induced writhing in mice. Test compounds were tested for their analgesic activity using the acetic acid-induced writhing test in mice. Piroxicam was used as a reference standard analgesic drug. Data represent the mean value ± SD of four mice per group. Statistical comparisons between basal and post-drug values were analyzed for statistical significance using one-way ANOVA followed by Dunnett’s test and denoted by *p < 0.05, **p < 0.01.
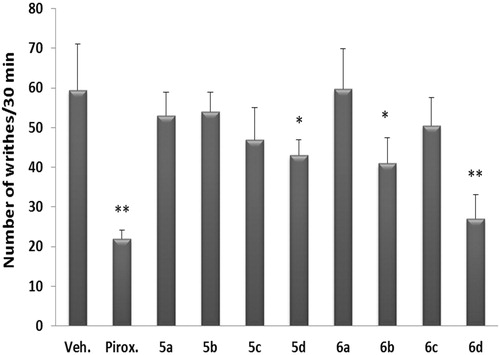
Molecular modeling
Since the anti-inflammatory activity is one of the most important biological activities of the prepared compounds 5a–d, 6a–d and taking in consideration that cyclooxygenase-2 (COX-2) is the most relevant enzymatic system for inflammationCitation11–14, a molecular modeling study was performed to understand the protein-inhibitor interactions and the structural features of the COX-2 active site and also, to investigate the possible binding conformation for the newly prepared compounds to this binding site, which may give a suggestion about their proposed mechanism of action as anti-inflammatory agents. Molecular docking study was done using the crystal structure of COX-2 (protein database [PDB] entry: 3NL1), with the prepared compounds and celecoxib as the reference ligand. The MOE version 2008.10 (Montreal, Quebec, Canada) modeling software (Molecular Operating Environment) was used for this studyCitation15. First of all, the celecoxib ligand was flexibly docked to the binding site, and the docking conformation corresponding to the lowest energy score value was selected as the most probable binding conformation, then we docked the prepared compounds 5a–d and 6a–d. Docking studies showed that these compounds occupied the COX-2 binding site as compared with celecoxib ().
Figure 4. Binding of the most active chalcone analogs 5b, 5d and the most active dihydro-pyrazoles 6b, 6d into the active site of COX-2 as assessed by computer modeling studies.
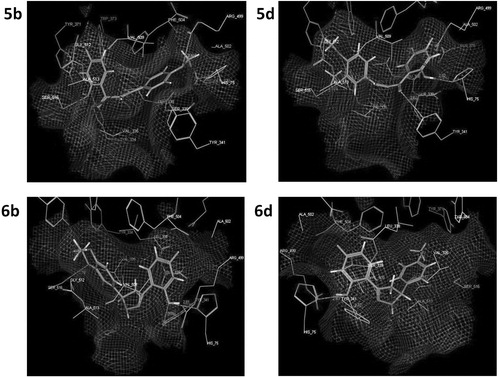
Within the chalcone series 5a–d, the binding energies for the most active derivatives 5d, 5b were found to be –18.71, –10.42 kcal/mol compared to –18.27 kcal/mol observed for celecoxib. Furthermore, compounds 5d and 5b exhibited two and three hydrogen bonding interactions, respectively, in comparison to three hydrogen bonding interactions exhibited by celecoxib. Similarly, within the dihydropyrazole series 6a–d, the most active compounds 6d, 6b showed the highest binding energy scores (–11.59, –13.68 kcal/mol, respectively) and the highest hydrogen bonding interactions (two for 6d and three for 6b) in comparison to celecoxib. The docking scores, amino acid interactions and the hydrogen bond lengths were summarized in .
Table 4. Docking results and consensus scores of 4′-flouro-2′-hydroxychalcones 5a–d, dihydropyrazoles 6a–d and celecoxib.
Conclusion
In summary, two series of new compounds, 4′-flouro-2′-hydroxychalcones 5a–d and the corresponding dihydropyrazoles 6a–d were synthesized for biological evaluation as antioxidant, anti-inflammatory and analgesic activities. The antioxidant activity studies showed that the dimethoxychalcone 5a and the corresponding dihydropyrazole 6a exhibited the highest activities which support data obtained from literature.Citation10 While, the anti-inflammatory activity studies showed that monomethoxychalcone 5d displayed good anti-inflammatory activity (54–62%), and the corresponding dihydropyrazoles 6d showed excellent anti-inflammatory activity (64–74%) comparable to that of celecoxib (32–78%). In addition, the docking experiments showed that compounds 5d and its cyclized derivative 6d better fit into the COX-2 active site, which was in parallel correlation with the in vitro COX inhibition evaluation. Furthermore, the analgesic activities of these compounds are clearly parallel to their anti-inflammatory activities. The relatively potent anti-inflammatory and analgesic activities exhibited by chalcone 5d and the corresponding dihydropyrazole derivative 6d support the drug design concept that cyclization of chalcones to the corresponding dihydropyrazole analogues maintain or increase the anti-inflammatory and analgesic activities.
Experimental
Chemistry
Melting points were determined on a Griffin apparatus and are uncorrected. IR spectra were recorded on a Shimadzu 435 spectrometer using KBr discs. 1H NMR and 13C NMR spectra were measured on a Bruker 400 MHz spectrometer (Faculty of Pharmacy, Beni-Suef University, Beni-Suef, Egypt) in D2O, DMSO-d6 with tetramethylsilane as the internal standard, where J (coupling constant) values were estimated in hertz (Hz). Mass spectra were run on Hewlett Packard 5988 spectrometer (Palo Alto, CA). Microanalysis was performed for C, H, N at the (Micro Analytical Center, Cairo University, Egypt) and was within ± 0.4% of theoretical values. All other reagents, purchased from the Aldrich Chemical Company (Milwaukee, WI), were used without further purification.
General method for preparation of 1,3-diarylprop-2-en-1-ones (5a–d)
4′-Flouro-2′-hydroxyacetophenone (3, 2.0 mmol, 0.38 g) and the appropriate substituted benzaldehyde (4a, 4b, 4c or 4d, 2.0 mmol) were dissolved in a minimum volume of ethanol (5–10 mL). A 10% sodium hydroxide solution (4 mL) was added slowly and the reaction mixture was stirred at room temperature for 2–6 h. The obtained solid was filtered, washed with water (5 mL) and then ethanol (5 mL) followed by crystallization from absolute ethanol to give analytically pure compounds 5a–d. Physical and spectral data are listed below.
3-(2,3-Dimethoxyphenyl)-1-(4-fluoro-2-hydroxyphenyl)prop-2-en-1-one (5a). Yield (91%); yellow crystals; m.p. 114–116 °C; IR (KBr disk) 3429 (OH), 3013 (C–H aromatic), 2945 (C–H aliphatic), 1641 (CO); 1H NMR (CDCl3) δ 3.92 (s, 3H, OCH3), 3.93 (s, 3H, OCH3), 6.63–6.74 (m, 2H, fluorophenyl H-3, H-5), 7.08 (d, J = 6.9 Hz, 1H, dimethoxyphenyl H-4), 7.15 (dd, J = 6.9, 7.8 Hz, 1H, dimethoxyphenyl H-5), 7.28 (d, J = 7.8 Hz, 1H, dimethoxyphenyl H-6), 7.68 (d, J = 15.6 Hz, 1H, COCH = CH), 7.93 (d, J = 8.7 Hz, 1H, fluorophenyl H-6), 8.21 (d, J = 15.6 Hz, 1H, COCH = CH), 13.24 (s, 1H, OH, D2O exchangeable); 13C NMR (CDCl3) δ 55.9, 56.0, 105.2, 107.2, 114.7, 117.2, 119.9, 121.3, 124.3, 128.6, 132.1, 140.7, 149.2, 153.3, 166.1, 168.7, 192.9; MS (m/z): 302 (M+, 0.7%) 80 (100%); Anal Calcd for C17H15FO4: C, 67.54; H, 5.00; Found: C, 67.71; H, 4.87.
3-(3,4-Dimethoxyphenyl)-1-(4-fluoro-2-hydroxyphenyl)prop-2-en-1-one (5b). Yield (78%); yellow crystals; m.p. 147–149 °C; IR (KBr disk) 3425 (OH), 3014 (C–H aromatic), 2938 (C–H aliphatic), 1632 (CO); 1H NMR (CDCl3) δ 3.93 (s, 3H, OCH3), 3.95 (s, 3H, OCH3), 6.63–6.73 (m, 2H, fluorophenyl H-3, H-5), 6.92 (d, J = 8.1 Hz, 1H, dimethoxyphenyl H-5), 7.17 (s, 1H, dimethoxyphenyl H-2), 7.28 (d, J = 8.1 Hz, 1H, dimethoxyphenyl H-6), 7.43 (d, J = 15.6 Hz, 1H, COCH = CH), 7.90 (d, J = 15.6 Hz, 1H, COCH = CH), 7.95 (d, J = 8.7 Hz, 1H, fluorophenyl H-6), 13.30 (s, 1H, OH, D2O exchangeable); 13C NMR (CDCl3) δ 56.0, 56.1, 105.2, 107.1, 110.3, 111.2, 117.0, 117.1, 124.1, 127.5, 131.9, 145.9, 149.2, 151.9, 166.2, 168.6, 192.5; MS (m/z): 302 (M+, 14%) 80 (100%); Anal Calcd for C17H15FO4: C, 67.54; H, 5.00; found: C, 67.63; H, 4.89.
1-(4-Fluoro-2-hydroxyphenyl)-3-(4-methylsulfanylphenyl)prop-2-en-1-one (5c). Yield (74%); yellow crystals; m.p. 166–168 °C; IR (KBr disk) 3423 (OH), 3067 (C–H aromatic), 2921 (C–H aliphatic), 1632 (CO); 1H NMR (CDCl3) δ 2.54 (s, 3H, SCH3), 6.63–6.74 (m, 2H, fluorophenyl H-3, H-5), 7.28 (d, J = 8.7 Hz, 2H, methylsulfanylphenyl H-3, H-5), 7.53 (d, J = 15.6 Hz, 1H, COCH = CH), 7.58 (d, J = 8.7 Hz, 2H, methylsulfanylphenyl H-2, H-6), 7.91 (d, J = 15.6 Hz, 1H, COCH = CH), 7.95 (d, J = 8.7 Hz, 1H, fluorophenyl H-6), 13.25 (s, 1H, OH, D2O exchangeable); 13C NMR (CDCl3) δ 15.1, 105.3, 107.2, 117.1, 118.6, 125.9, 129.1, 130.8, 131.9, 143.3, 145.2, 166.1, 168.7, 192.4; MS (m/z): 288 (M+, 97%) 137 (100%); Anal Calcd for C16H13FO2S: C, 66.65; H, 4.54; found: C, 66.84; H, 4.92.
1-(4-Fluoro-2-hydroxyphenyl)-3-(4-methoxylphenyl)prop-2-en-1-one (5d). Yield (82%); yellow crystals; mp 122–124 °C; IR (KBr disk) 3430 (OH), 3073 (C–H aromatic), 2966 (C–H aliphatic), 1641 (CO); 1H NMR (CDCl3) δ 3.88 (s, 3H, OCH3), 6.67–6.74 (m, 2H, fluorophenyl H-3, H-5), 6.99 (d, J = 8.7 Hz, 2H, methoxyphenyl H-3, H-5), 7.37 (d, J = 8.7 Hz, 2H, methoxyphenyl H-2, H-6), 7.56 (d, J = 15.6 Hz, 1H, COCH = CH), 7.91 (d, J = 15.6 Hz, 1H, COCH = CH), 7.94 (d, J = 8.7 Hz, 1H, fluorophenyl H-6), 13.18 (s, 1H, OH, D2O exchangeable); 13C NMR (CDCl3) δ 55.4, 105.3, 107.3, 113.8, 116.7, 120.2, 121.3, 130.1, 135.8, 145.7, 160.0, 166.2, 168.8, 192.5; MS (m/z): 272 (M+, 5%) 80 (100%); Anal. Calcd for C16H13FO3: C, 70.58; H, 4.81; found: C, 70.89; H, 4.49.
General method for preparation of 3,5-diaryl-4,5-dihydro-1H-pyrazoles(6a–d)
To a solution of the appropriate chalcone 5a, 5b, 5c or 5d (1.0 mmol) in ethanol (10 mL), hydrazine hydrate (1.2 mmol, 0.06 g) was added, and the reaction mixture was refluxed for 2–4 h. The reaction was monitored every 60 min interval on TLC plates using chloroform:methanol (9:1 V/V). The excess solvent was removed in vacuum, and the residue was washed with water, crystallized from absolute ethanol/methanol mixture (1:1) to give the respective dihydropyrazoles 6a–d for which physical and spectral data are listed below.
5-(2,3-Dimethoxyphenyl)-3-(4-fluoro-2-hydroxyphenyl)-4,5-dihydro-1H-pyrazole (6a). Yield (78%); white crystals; m.p. 96–98 °C; IR (KBr disk) 3427–3373 (OH, NH), 3079 (C–H aromatic), 2938 (C–H aliphatic), 1609 (C = N); 1H NMR (CDCl3) δ 3.09 (dd, J = 16.5, 8.7 Hz, 1H, pyrazole H-4), 3.56 (dd, J = 16.5, 10.2 Hz, 1H, pyrazole H'-4), 3.89 (s, 3H, OCH3), 3.90 (s, 3H, OCH3), 5.23 (ddd, J = 10.2, 8.7, 0.9 Hz, 1H, pyrazole H-5), 6.60 (ddd, J = 7.8, 1.8, 0.9 Hz, 1H, dimethoxyphenyl H-6), 6.72 (dd, J = 7.8, 1.8 Hz, 1H, dimethoxyphenyl H-4), 6.91 (dd, J = 7.8, 7.8 Hz, 1H, dimethoxyphenyl H-5), 7.04–7.27 (m, 3H, fluorophenyl H-3, H-5, H-6), 10.55 (s, 1H, NH, D2O exchangeable), 13.27 (s, 1H, OH, D2O exchangeable); 13C NMR (DMSO-d6) δ 40.9, 56.2, 56.7, 60.8, 103.5, 106.8, 112.5, 114.4, 118.0, 124.5, 129.7, 136.2, 146.7, 152.8, 159.0, 161.9, 164.3; MS (m/z): 316 (M+, 0.2%) 271 (100%); Anal Calcd for C17H17FN2O3: C, 64.55; H, 5.42; N, 8.86; found: C, 64.69; H, 5.57; N, 8.86.
5-(3,4-Dimethoxyphenyl)-3-(4-fluoro-2-hydroxyphenyl)-4,5-dihydro-1H-pyrazole (6b). Yield (69%); white crystals; m.p. 144–146 °C; IR (KBr disk) 3393–3331 (OH, NH), 3071 (C–H aromatic), 2934 (C–H aliphatic), 1605 (C = N); 1H NMR (DMSO-d6) δ 3.22 (dd, J = 6.5, 8.7 Hz, 1H, pyrazole H-4), 3.61 (dd, J = 16.5, 10.2 Hz, 1H, pyrazole H'-4), 3.87 (s, 3H, OCH3), 3.88 (s, 3H, OCH3), 4.97 (dd, J = 10.2, 8.7 Hz, 1H, pyrazole H-5), 6.61 (dd, J = 8.4, 2.4 Hz, 1H, dimethoxyphenyl H-2), 6.72 (dd, J = 8.4, 2.4 Hz, 1H, dimethoxyphenyl H-6), 6.78 (d, J = 8.4 Hz, 1H, dimethoxyphenyl H-5), 6.91–6.97 (m, 2H, fluorophenyl H-3, H-5), 7.15–7.27 (m, 1H, fluorophenyl H-6), 10.53 (s, 1H, NH, D2O exchangeable), 13.31 (s, 1H, OH, D2O exchangeable);13C NMR (DMSO-d6) δ41.4, 55.9, 56.0, 62.5, 103.6, 106.8, 111.0, 112.2, 114.4, 119.3, 129.8, 134.8, 149.2, 152.7, 159.1, 161.9, 164.3; MS (m/z): 316 (M+., 35%) 151 (100%); Anal Calcd for C17H17FN2O3: C, 64.55; H, 5.42; N, 8.86; found: C, 64.82; H, 5.61; N, 9.11.
3-(4-Fluoro-2-hydroxyphenyl)-5-(4-methylsulfanylphenyl)-4,5-dihydro-1H-pyrazole (6c). Yield (65%); pale yellow crystals; m.p. 152–154 °C; IR (KBr disk) 3408–3324 (OH, NH), 3066 (C–H aromatic), 2917 (C–H aliphatic), 1602 (C = N); 1H NMR (DMSO-d6) δ 2.47 (s, 3H, SCH3), 3.29 (dd, J = 16.5, 8.7 Hz, 1H, pyrazole H-4), 3.65 (dd, J = 16.5, 10.2 Hz, 1H, pyrazole H'-4), 5.01 (dd, J = 10.2, 8.7 Hz, 1H, pyrazole H-5), 6.64–6.80 (m, 3H, fluorophenyl H-3, H-5, H-6), 7.13–7.33 (m, 4H, methysulfanylphenyl H-2, H-3, H-5, H-6), 10.52 (s, 1H, NH, D2O exchangeable), 13.25 (s, 1H, OH, D2O exchangeable); 13C NMR (DMSO-d6) δ13C NMR (DMSO-d6) δ15.7, 40.4, 60.3, 103.5, 106.9, 113.9, 126.3, 127.2, 132.1, 134.6, 138.1, 155.7, 161.3, 165.1; MS (m/z): 302 (M+, 0.12%) 80 (100%); Anal Calcd for C16H15FN2OS: C, 63.56; H, 5.00; N, 9.26; found: C, 63.19; H, 5.03; N, 9.39.
3-(4-Fluoro-2-hydroxyphenyl)-5-(4-methoxylphenyl)-4,5-dihydro-1H-pyrazole (6d). Yield (70%); yellow crystals; m.p. 115–117 °C; IR (KBr disk) 3409–3321 (OH, NH), 3056 (C–H aromatic), 2932 (C–H aliphatic), 1609 (C = N); 1H NMR (DMSO-d6) δ 3.30 (dd, J = 16.5, 8.7 Hz, 1H, pyrazole H-4), 3.67 (dd, J = 16.5, 10.2 Hz, 1H, pyrazole H'-4), 3.93 (s, 3H, OCH3), 5.13 (dd, J = 10.2, 8.7 Hz, 1H, pyrazole H-5), 6.67–6.86 (m, 3H, fluorophenyl H-3, H-5, H-6), 7.18–7.48 (m, 4H, methysulfanylphenyl H-2, H-3, H-5, H-6), 10.55 (s, 1H, NH, D2O exchangeable), 13.31 (s, 1H, OH, D2O exchangeable); 13C NMR (DMSO-d6) δ40.3, 55.8, 60.9, 103.8, 106.6, 111.5, 113.4, 117.3, 128.2, 135.1, 152.7, 159.6, 162.6, 165.0; MS (m/z): 286 (M+., 0.03%) 80 (100%); Anal Calcd for C16H15FN2O2: C, 67.12; H, 5.28; N, 9.78; found: C, 66.87; H, 5.17; N, 9.95.
Biological evaluation
Animals
Swiss albino mice 20–25 g and Wistar rats (150–175 g) were obtained from the National Research Center, Cairo, Egypt) were used throughout the study and were kept at controlled conditions (temperature 23 ± 2 °C, humidity 60 ± 10%) and a 12/12 h light/dark cycle. All procedures relating to animal care and treatments were conducted in accordance with protocols approved by the Research Ethical Committee of Faculty of Pharmacy Beni-Suef University (2014-Beni-Suef, Egypt).
Material
DPPH radical was obtained from Sigma-Aldrich (St. Louis, MO). Ascorbic was purchased from Merck (Rahway, NJ). All the used chemicals and solvents were of analytical grade.
DPPH radical scavenging activity
Antioxidant activity was evaluated as the scavenging activity of 1,1-diphenyl-2-picrylhydrazyl (DPPH) radical essentially as mentioned beforeCitation16–18. In a brief, 2 mL of DPPH solution (0.2 mmol/L in ethanol) was incubated with different concentrations of the test compounds. The reaction mixtures were shaken and wrapped in aluminum foil and kept at room temperature for 30 min in dark. The spectrophotometric measurements at 517 nm were done under dim light. All data are presented as mean ± standard deviation (SD) (n = 3). The IC50 (the concentration of sample necessary to cause 50% inhibition of DPPH radical scavenging activity) was calculated for each compound. Ascorbate (Vitamin C) was used as a positive antioxidant control.
Yeast-based antioxidant screening assay
The yeast-based biological assay detects antioxidant activities of test compounds against physiologically relevant oxidants. DPPH radical scavenging activity method provides only an indication of the ability of a compound to scavenge oxidantsCitation19. For this reason, we used yeast-based method, which can also measure the ability of a compound to induce cellular resistance to the damaging effects of oxidantsCitation19. Antioxidant activity screening was done in a 96-well microplate high throughput assay using Saccharomyces cerevisiae as described beforeCitation18,Citation20. Briefly, S. cerevisiae (BY4743) were cultured overnight then diluted to an optical density of 0.2 at 600 nm (OD600) in the media. Diluted culture (180 μL) was added per well in a 96-well plate, and then 10 μL of test compound was added to a final concentration of 8 mM. H2O2 (10 μL) was added into each well to a final concentration of 4 mM. The OD600 reading as an indicator of yeast growth was taken using a microplate reader before and after the incubation at 30 °C with shaking for 20 h. Ascorbic acid was used as a positive control. The net growth of yeast after the treatment with H2O2 and test compounds was measured using the following equation:
where PYeast growth = net growth of yeast cells after treatment with test compounds; PSample = observed optical density of yeast cells with the treatment with test compounds; PControl = observed optical density of yeast cells with the treatment of negative control.
COX-1/COX-2 inhibition colorimetric assay
The in vitro inhibition of COX-1/COX-2 was measured using colorimetric COX (ovine) Inhibitor Screening Assay Kit (Cayman Chemical, Ann Arbor, MI) according to the manufacturer’s instructions and as mentioned beforeCitation21. Different concentrations of test compounds or celecoxib were incubated with the enzymes for 5 min at 25 °C. After the incubation and addition of colorimetric substrate and arachidonic acid, the absorbance was measured at 590 nm using plate reader.
Carrageenan-induced rat paw edema assay
Rats were administered vehicle, test compounds (10 mg/kg) or Celecoxib (10 mg/kg) by oral gavage. Immediately thereafter and under light anesthesia, the animals received 100 μl of vehicle or carrageenan (1% in saline) s.c. on the plantar surface of the left hindpaw as reported beforeCitation22,Citation23. The development of paw edema was assessed by measuring paw-volume changes at 1, 3 and 6 h after carrageenan injection using plethysmometer. The right hindpaw served as a reference of non-inflamed paw for comparison. Results are expressed as paw-volume change (ml).
Hot-plate test
The test was performed as mentioned beforeCitation24. Basal, 1 and 2 h values of hot-plate latency (seconds) of saline (control) and compounds treated mice (10 mg/kg). Piroxicam (10 mg/kg) was used as positive control. Compounds were administered by oral gavage 1 h before testing. A cutoff latency of 30 s was used to prevent heat-induced tissue damage.
Acetic acid-induced writhing test
The writhing tests were carried as described beforeCitation25. Briefly, mice were pre-treated orally by vehicle, test compounds (10 mg/kg) or piroxicam (10 mg/kg) 30 min prior to intraperitoneal administration of 0.7% v/v acetic acid solution at 10 ml/kg body weight. The number of writhes (i.e. abdominal constriction followed by dorsiflexion and extension) occurring during a 30-min period beginning 5 min after acetic acid injection was measured. The results are expressed as the number of writhes per 30-min period.
Statistical analysis
Comparisons among groups were analyzed for statistical significance using one-way ANOVA followed by Dunnett’s test. Data were represented as mean ± SD. Differences were considered significant at *p < 0.05, and **p < 0.01, respectively.
Molecular modeling and docking
The crystal structure of celecoxib bound at the Mus musculus COX-2 active site (obtained from protein data bank at research collaboration for structural Bioinformatics PDB entry 3LN1) was used in this studyCitation15. Docking of the co-crystallized ligand should be carried out to study the scoring energy(s), root mean standard deviation and amino acid interactions. The root mean square deviation, which is measure of superposing, was 0.50 A° for the lead compound. Docking was performed using London dG force, and refinement of the results was done using Force field energy.
Preparation of the synthesized compounds for docking was achieved via their 3D structure built by MOE. Certain procedures should be taken before docking, which include: 3D protonation of the structures, running conformational analysis using systemic search, selecting the least energetic conformer and applying the same docking protocol used with ligand.
The previous measures were taken and docking for the synthesized compounds was applied. Amino acid interactions and the hydrogen bond lengths were summarized in .
Declaration of interest
The authors have declared no conflict of interest.
References
- Detsi A, Majdalani M, Kontogiorgis CA, et al. Natural and synthetic 2′-hydroxy-chalcones and aurones: synthesis, characterization and evaluation of the antioxidant and soybean lipoxygenase inhibitory activity. Bioorg Med Chem 2009;17:8073–85
- Avila HP, Smania Ede F, Monache FD, Smania A Jr. Structure-activity relationship of antibacterial chalcones. Bioorg Med Chem 2008;16:9790–4
- Bano S, Javed K, Ahmad S, et al. Synthesis of some novel chalcones, flavanones and flavones and evaluation of their anti-inflammatory activity. Eur J Med Chem 2013;65:51–9
- Vogel S, Barbic M, Jurgenliemk G, Heilmann J. Synthesis, cytotoxicity, anti-oxidative and anti-inflammatory activity of chalcones and influence of A-ring modifications on the pharmacological effect. Eur J Med Chem 2010;45:2206–13
- Piotrowska DG, Cieslak M, Krolewska K, Wroblewski AE. Design, synthesis and cytotoxicity of a new series of isoxazolidines derived from substituted chalcones. Eur J Med Chem 2011;46:1382–9
- Shenvi S, Kumar K, Hatti KS, et al. Synthesis, anticancer and antioxidant activities of 2,4,5-trimethoxy chalcones and analogues from asaronaldehyde: structure-activity relationship. Eur J Med Chem 2013;62:435–42
- Cheng JH, Hung CF, Yang SC, et al. Synthesis and cytotoxic, anti-inflammatory, and anti-oxidant activities of 2′,5′-dialkoxylchalcones as cancer chemopreventive agents. Bioorg Med Chem 2008;16:7270–6
- Tran TD, Park H, Kim HP, et al. Inhibitory activity of prostaglandin E2 production by the synthetic 2′-hydroxychalcone analogues: synthesis and SAR study. Bioorg Med Chem Lett 2009;19:1650–3
- Padhye S, Ahmad A, Oswal N, et al. Fluorinated 2′-hydroxychalcones as garcinol analogs with enhanced antioxidant and anticancer activities. Bioorg Med Chem Lett 2010;20:5818–21
- Bandgar BP, Gawande SS, Bodade RG, et al. Synthesis and biological evaluation of simple methoxylated chalcones as anticancer, anti-inflammatory and antioxidant agents. Bioorg Med Chem 2010;18:1364–70
- Abdellatif KR, Chowdhury MA, Dong Y, et al. Diazen-1-ium-1,2-diolated nitric oxide donor ester prodrugs of 5-(4-hydroxymethylphenyl)-1-(4-aminosulfonylphenyl)-3-trifluoromethyl-1H-pyrazole and its methanesulfonyl analog: synthesis, biological evaluation and nitric oxide release studies. Bioorg Med Chem 2008;16:9694–8
- Abdellatif KR, Chowdhury MA, Dong Y, et al. Diazen-1-ium-1,2-diolated nitric oxide donor ester prodrugs of 5-(4-carboxymethylphenyl)-1-(4-methanesulfonylphenyl)-3-trifluoromethyl-1H-pyrazo le and its aminosulfonyl analog: synthesis, biological evaluation and nitric oxide release studies. Bioorg Med Chem 2009;17:5182–8
- Abdellatif KR, Chowdhury MA, Velazquez CA, et al. Celecoxib prodrugs possessing a diazen-1-ium-1,2-diolate nitric oxide donor moiety: synthesis, biological evaluation and nitric oxide release studies. Bioorg Med Chem Lett 2010;20:4544–9
- Abdellatif KR, Huang Z, Chowdhury MA, et al. A diazen-1-ium-1,2-diolated nitric oxide donor ester prodrug of 3-(4-hydroxymethylphenyl)-4-(4-methanesulfonylphenyl)-5H-furan-2-one: synthesis, biological evaluation and nitric oxide release studies. Bioorg Med Chem Lett 2011;21:3951–6
- Wang JL, Limburg D, Graneto MJ, et al. The novel benzopyran class of selective cyclooxygenase-2 inhibitors. Part 2: the second clinical candidate having a shorter and favorable human half-life. Bioorg Med Chem Lett 2010;20:7159–63
- Schlesier K, Harwat M, Bohm V, Bitsch R. Assessment of antioxidant activity by using different in vitro methods. Free Radic Res 2002;36:177–87
- Liu B, Yang J, Ma Y, et al. Antioxidant and angiotensin converting enzyme (ACE) inhibitory activities of ethanol extract and pure flavonoids from Adinandra nitida leaves. Pharm Biol 2010;48:1432–8
- Abdelgawad MA, Elshemy HAH, Abdellatif KRA, Omar HA. Synthesis of novel substituted 4H-chromenes and their antioxidant screening. J Chem Pharm Res 2013;5:387–94
- Wu MJ, O'Doherty PJ, Fernandez HR, et al. An antioxidant screening assay based on oxidant-induced growth arrest in Saccharomyces cerevisiae. FEMS Yeast Res 2011;11:379–87
- Ravipati AS, Zhang L, Koyyalamudi SR, et al. Antioxidant and anti-inflammatory activities of selected Chinese medicinal plants and their relation with antioxidant content. BMC Complement Altern Med 2012;12:173
- Abdelazeem AH, Abdelatef SA, El-Saadi MT, et al. Novel pyrazolopyrimidine derivatives targeting COXs and iNOS enzymes; design, synthesis and biological evaluation as potential anti-inflammatory agents. Eu J Pharm Sci 2014;62:197–211
- Salvemini D, Wang ZQ, Wyatt PS, et al. Nitric oxide: a key mediator in the early and late phase of carrageenan-induced rat paw inflammation. Br J Pharmacol 1996;118:829–38
- El-Nezhawy AO, Biuomy AR, Hassan FS, et al. Design, synthesis and pharmacological evaluation of omeprazole-like agents with anti-inflammatory activity. Bioorg Med Chem 2013;21:1661–70
- Fakhr IM, Radwan MA, el-Batran S, et al. Synthesis and pharmacological evaluation of 2-substituted benzo[b]thiophenes as anti-inflammatory and analgesic agents. Eur J Med Chem 2009;44:1718–25
- Arrigoni-Martelli E. Screening and assessment of antiinflammatory drugs. Methods Find Exp Clin Pharmacol 1979;1:157–77