Abstract
The study has demonstrated a dual effect of nitric oxide on phenoloxidase (PO)-mediated DOPA oxidation and melanization process. NO generated at low rates proportionally increased in PO-mediated DOPA oxidation. Competitive PO inhibitor, phenylthiourea, resulted in significant inhibition of NO-mediated DOPA oxidation. Further analysis using fluorescent and EPR methods demonstrated that the effect of NO on DOPA oxidation is explained by oxidation of NO to NO2 at the active site of PO followed by oxidation of DOPA by NO2. On the contrary, the bolus addition of NO gas solution resulted in a significant decrease in observed PO activity. Similar dose-dependent effect of NO was observed for the insect’s haemocytes quantified as percentage of melanized cells after treatment with nitric oxide. In conclusion, the results of the study suggest that NO may have a significant regulatory role on melanization process in invertebrates as well as in human and result in protective or damaging effects.
Introduction
Melanization is the process of formation of natural polymer melanin, which is widespread in living organisms. The browning of fruit and root shears, the dark color of animal skin and hair are caused mostly by melanin formation. The process performs various functions in different organisms, the most important of which is the protective activity. Phenoloxidase (PO) is the key enzyme of the melanization processCitation1,Citation2. In invertebrates, the melanization underlies the immune response. The penetration of pathogen into an organism initiates the melanization cascade which finally results in the formation of a melanin capsule around the pathogenCitation3,Citation4. The particular mechanisms of triggering and regulation of this process were active studyingCitation5, but many aspects are still unclear.
PO (or tyrosinase) refers to oxygenases (monophenol, ortho-diphenol: oxygen oxidoreductase, EC 1.14.18.1). It catalyzes the initial steps of the melanization process, namely the hydroxylation of monophenols and oxidation of ortho-diphenols by molecular oxygen into the corresponding ortho-quinones. Then these quinones undergo a spontaneous intramolecular cyclization to yield indoles that polymerize subsequently into melanin through a cascade of reactions. The active site of PO contains a coupled binuclear copper similar to the active site of haemocyanin, the arthropod’s oxygen carrier protein. Phenoloxidases are widely distributed in living organisms: from bacteria to mammals. Human and mouse phenoloxidases are the transmembrane enzymes consisting of two subunits, whereas those of fungi and plants have only one not membrane-bound subunitCitation1,Citation2,Citation6,Citation7. The phenoloxidases selected from the haemolymph of certain insects are very similar to that of fungiCitation8,Citation9. On substrate oxidation, the active site of PO turns step-by-step into three states: met- with Cu(II)–Cu(II), deoxy- Cu(I)–Cu(I), and oxy- Cu(II)–O2–Cu(II)Citation7,Citation10,Citation11. The native PO is presented mainly by the met- form. Some investigationsCitation11,Citation12 report that NO manifests a high affinity for copper-containing enzymes and proteins, such as hemocyanin, tyrosinase, laccase, and cytochrome c.
In the case of humans, melanin pigmentation is stimulated mainly by ultraviolet radiation (UVR). The chemical NO donors are shown to mimic the melanogenic effects of UVR by increasing the PO activity and, hereby, the melanin synthesis in human melanocytes. In author’s opinionCitation13,Citation14, increasing in melanin formation in human melanocytes by NO is achieved rather through cGMP path than by increasing activity of phenoloxidase due to the interaction of PO with NO.
Another effect of nitric oxide on the mushroom PO-mediated oxidation of dopamine and DOPA is reported by Nappi and VassCitation15. NO was found to promote a significant decrease in the total amount of DOPA and dopamine oxidized under experimental conditions. The reported inhibitory effect of NO on the PO activity was assumed to result from the formation of a copper–nitrosyl complex at the active site of enzyme. These findings allow suggesting that NO may have a dual effect on melanization and thereby may have regulatory role in melanization process in invertebrates as well as in human and result in protective or damaging effects. The aim of our work was to understand how interaction of NO with PO affects the enzyme activity and melanization.
Materials and methods
Chemicals
3-(3,4-Dihydroxyphenyl)-l-alanine (DOPA), mushroom phenoloxidase (PO), and phenylthiourea (PTU) were purchased from Sigma (St. Louis, MO). Nitronylnitroxyl radical (4-methoxy-5,5-dimethyl-2-(pyridine-3-yl)-4,5-dihydroimidazol-1-oxyl), NNR, was kindly provided by Dr. I. Kirilyuk (Institute of Organic Chemistry of RAS SB, Novosibirsk, Russia). Fluorescent probe DAF-FM (4-amino-5-methylamino-2’,7’-difluorofluoresceine) was purchased from Molecular Probes (Eugene, OR).
Synthetic donor, PAPA NONOate (PAPA/NO, (3-(2-hydroxy-2-nitroso-1-propylhydrazino)-1-propanamine), purchased from Cayman (Ann Arbor, MI) was used as a compound to generate NO. PAPA/NO decomposes to release two NO molecules into solution with a half-life of 77 min at neutral pH and room temperatureCitation16. Stock solution was prepared in 10 mM NaOH and stored at −20 °C. The concentration was determined from absorbance values at 250 nm in 10 mM NaOH (ɛ = 8 M−1 cm−1). The rate of NO generation was determined in diluted PAPA/NO solution by EPR method using NNR as NO-scavengerCitation17. An aliquot of PAPA/NO stock solution was diluted with phosphate buffer to 0.5 mM and 0.1 mM NNR was added. NO formation rate was determined from decrease of EPR signal of NNR taking into account that stoichiometry of the reaction between NNR and NO is 1:1. The required rate of NO generation was regulated by appropriate dilution of the PAPA/NO stock solution.
Measurements were performed using the Bruker X-band EPR spectrometer ER 200D-SRC (Bruker Corporation, Billerica, MA). Gaseous nitric oxide was prepared by reduction of NaNO2 with FeSO4 at the acidic conditions and purified from higher oxides by passing through a deaerated NaOH solution. Assays were performed using NO-saturated solution, prepared by bubbling NO gas in deaerated PBS. The concentration of NO in stock solution was assumed as 2 mMCitation18.
Monitoring of DOPA oxidation by phenoloxidase
The experimental mixtures contained 20 U/ml PO and the various concentrations of DOPA. To determine the NO effect, an appropriate concentration of either PAPA/NO or NO gas solution was added to the mixture before the addition of DOPA. In certain experiments, 0.5 μM PTU was added to a solution of PO. The sample was placed into a 1 cm quartz cell and the rate of DOPA-chrome formation was measured at 490 nm (ɛ = 3700 M−1 cm−1)Citation19 using an UV-2401 (PC) CE (“Shimadzu” Corp., Tokyo, Japan). Experiments were carried out at 28 °C.
Detection of DAF-FM triazole formation
To test the interaction between PO and NO, the time course of fluorescent DAF-FM triazole intensity dependent on PO concentration was studied using a DAF-FM probeCitation20. Experimental mixtures contained 5 μM DAF-FM, PAPA/NO (NO generation rate ∼ 2 μM/min), and PO 200, 600, or 1200 U/ml in PBS. Fluorescence kinetics were recorded using a microplate fluorometer. Experimental conditions are described inCitation21.
Detection of half-met form of phenoloxidase by EPR
The mixture of 0.5 mM PO and 10 mM PAPA/NO in PBS was incubated for 30 min at ambient temperature. Then, glycerol was added to the experimental sample up to 50% (v/v) and the EPR spectrum was recorded at 105 °K using an Elexsys E 560-P spectrometer (Bruker Corporation, Billerica, MA).
Detection of melanization in Galleria mellonella haemocytes
Preparation of haemocyte samples from haemolymph of G. mellonella larvae was carried out according toCitation22. Haemocyte suspension (104 cells) was placed on a microscope slide. The slides were kept in a moist chamber at 22 °C for 15 min to allow haemocytes to adhere and spread. To fix the haemocytes on the slides, acetone (100 μl) was added and the slides were kept in a moist chamber in the dark for 10 min. Then the haemocytes on the slides were washed three times in PBS, and 20 mM DOPA was added to all the samples and either PAPA/NO (30 μM/min) or the buffered solution of NO gas (25 μM) was added to the experimental slides. The slides were stored in the dark moist chamber for 40 min and then washed with distilled water and checked for the presence of dark-grey deposits in haemocytes (indicative of melanin formation) using a light microscope LUMAM R3 (Russia). The percentage of melanized haemocytes was calculated.
All experiments were carried out in the phosphate buffer solution (PBS, 50 mM Na–phosphate, 150 mM NaCl, pH 7.4) prepared with bidistilled deionized water.
Statistical analysis
The data were analyzed using the software SigmaPlot for Windows, version 9.0 (Systat Software, Inc., San Jose, CA). When necessary, a statistical analysis was performed and the data were expressed as means ± SE (n ≥ 5). Significant differences of data were analyzed by Student's t-test (p < 0.05).
Results and discussion
The effect of nitric oxide from PAPA/NO on DOPA oxidation catalyzed by PO
To evaluate the NO effect on DOPA oxidation, catalyzed by phenoloxidase, the experiments were carried out at varying concentration of PAPA/NO. Preliminarily, a mixture of PO and PAPA/NO was incubated for 2 min to reach stationary condition of NO generation. Thereafter 1 mM DOPA was added and the kinetics of DOPA-chrome formation, the product of DOPA oxidation, was registered with a spectrophotometer. demonstrates the kinetics of DOPA-chrome formation at different PAPA/NO concentrations. As a result, the nitric oxide activates the DOPA oxidation depending on PAPA/NO concentration. shows the calculated rates of PO-mediated DOPA-chrome formation versus the NO generation rates (close circles). For comparison, the oxidation of DOPA in the presence of PAPA/NO without enzyme was also registered (, open circles). In both cases, the increase of NO generation rate results in a proportional increase of the DOPA oxidation rate. However, in the presence of PO, it was much more effective (slope 1.1 ± 0.6) than for the non-enzymatic pathway (slope 0.0 25 ± 0.0008). It seems that the nitric oxide causes the increase in the apparent PO activity.
Figure 1. The time curves of DOPA-chrome formation during oxidation of DOPA (1 mM) by phenoloxidase (20 units/ml) in the presence of PAPA/NO: (a) 0 mM, (b) 0.05 mM, (c) 0.1 mM, (d) 0.25 mM, (e) 0.5 mM, (f) 1 mM. T = 28 °C, λ = 490 nm.
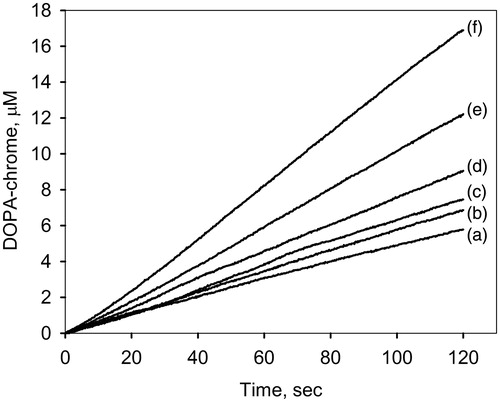
Figure 2. The initial rates of DOPA-chrome formation versus the NO generation rates in the presence of PO (close circles) and without (open circles). The data were obtained by spectrophotometric method under conditions given in Figure 1.
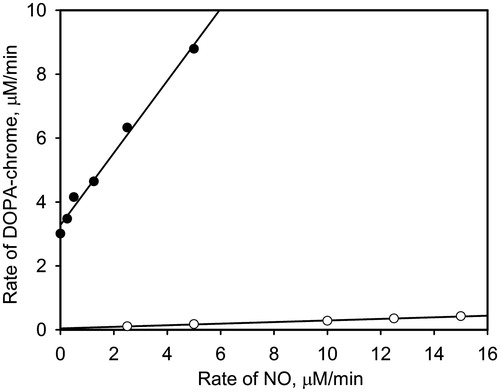
To evaluate the observed effect of NO on PO activity, the dependence of the initial rate of PO-mediated DOPA oxidation on DOPA concentration was investigated. compares this dependence with control samples (without PAPA/NO). The data of fitting in terms of the Michaelis–Menten equation demonstrate that Vmax increases from 18 ± 0.8 μM/min to 23 ± 1.5 μM/min, whereas the KM value remains almost the same (0.30 ± 0.04 mM for control samples and 0.24 ± 0.05 mM with PAPA/NO). These data allow the conclusion that NO generated from PAPA/NO has no essential effect on the PO activity, but leads to the additive PO-mediated pathway of DOPA oxidation.
Figure 3. The initial rates of PO-mediated DOPA-chrome formation versus DOPA concentration in the presence of PAPA/NO (0.4 mM, open circles) and without (close circles). The concentration of PO in mixtures was 20 units/ml.
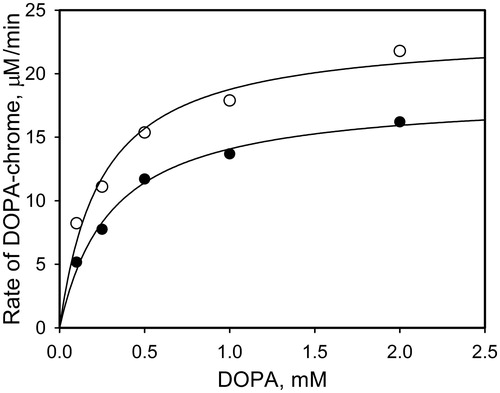
The nitric oxide can react with the met- form of PO (Cu2+ –Cu2+) reducing it to the half-met PO (Cu2+–Cu1+)Citation23–25. It is reasonable to propose that the nitric oxide is oxidized hereby to the highly reactive species, namely nitric dioxide, NO2. Thereafter, it could oxidize DOPA to DOPA-chrome. The next chemical reactions can be involved in this process:
Reaction (1) demonstrates that NO2 formation in aqueous aerobic solutions is of second order in NO. At low NO generation rate (as for PAPA/NO), this reaction could be very slowCitation26. In our experiments, this condition was realized in the system without PO which results in a slight oxidation of DOPA (, open circles). Reactions (2) and (3) describe the processes of PO-mediated oxidation of NO to NO2, followed by the oxidation of DOPA to DOPA-chrome. It is assumed, then, that the observed effect of PAPA/NO on the PO-mediated oxidation of DOPA ( and ) is due to the DOPA oxidation by NO2 in addition to its enzymatic oxidation. NO2 can be formed by NO oxidation at the active site of PO.
Nitric oxide oxidation in the presence of PO
To study PO-mediated oxidation of NO to NO2 proposed above, we apply the fluorescent method using DAF-FM as a probe. This fluorescent probe is widely used to register nitric oxide; however, it does not react directly with nitric oxide but with its oxidized forms, NO2Citation27 or N2O3Citation20, resulting in the formation of a highly fluorescent product, DAF-FM triazole. In our previous studyCitation21, we have observed that the rate of DAF-FM triazole formation is proportional to generation rate of NO2. In the present study, we added PO to the system PAPA/NO and DAF-FM. According to Reactions (1) and (2), the formation of NO2 followed by DAF-FM triazole production should depend on PO concentration. demonstrates the obtained linear dependence of fluorescence rate versus PO concentration. This result indicates that the formation of NO2 from NO depends on the presence of PO.
Figure 4. Dependence of the rate of DAF-FM-triazole formation on PO concentration in the mixture of PAPA/NO (NO generation rate of 2 μM/min) and DAF-FM (5 μM).
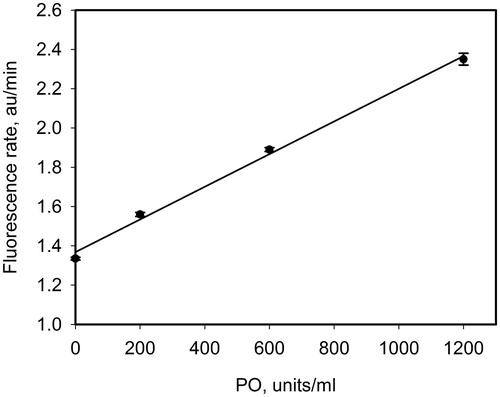
Therefore, the finding support the above statement that the apparent increasing effect of PAPA/NO on the rate of PO-mediated DOPA oxidation could be explained by the additional pathway of oxidation of DOPA by NO2 formed in this system. Scheme 1(A) describes two proposed paths of DOPA-chrome formation: enzymatic and NO-mediated. Oxidation of NO in this system is presented as the enzymatic-like process, where the active center of PO undergoes re-oxidation by oxygen.
The effect of nitric oxide on PO-mediated DOPA oxidation in the presence of inhibitor
As was proposed above, oxidation of nitric oxide to dioxide can occur at the active site of PO. Under this assumption, the addition of a competitive inhibitor of PO, notably PTUCitation28,Citation29, should suppress PO-mediated oxidation of NO. It is based on the reported data that PTU bounds to both the copper ions at the active site of catechol-oxidaseCitation30. The mixtures of PO, PTU, and the different concentrations of PAPA/NO were incubated for 2 min. After that 1 mM DOPA was added and the time-dependent accumulation of DOPA-chrome was measured. demonstrates the dependences of the rate of PO-mediated DOPA-chrome formation versus the rate of NO generation by PAPA/NO in the presence of PTU (open circles) and without (close circles). Increasing effect of NO on the DOPA oxidation rate in the presence of the inhibited PO is negligible, which confirms the supposition that NO is oxidized at the active site of PO.
Interaction between nitric oxide and the active site of PO observed by EPR method
The met- form of PO, which is the main for native enzyme, contains two Cu2+ ions at the active siteCitation7. The EPR spectrum of PO is, however, unobservable due to strong antiferromagnetic interactions between ionsCitation23. Nitric oxide is known to convert the met- into the half-met form of PO due to reduction of one copper ion that can be detected by EPR methodCitation23. It was revealed the final form of enzyme contains the half-met-centre [Cu1+–Cu2+] without NO boundCitation31. We performed the EPR measurements of PO at the temperature of liquid nitrogen (). The incubation of PO with PAPA/NO resulted in the appearance of EPR spectrum. Adding the competitive inhibitor, PTU, to this sample had no effect on the spectrum. Nevertheless, the incubation of PO in reverse order, i.e. first with PTU and then with PAPA/NO, failed to give the EPR spectrum. This observation pointed that PTU blocked NO interaction with PO active site by binding both Cu-ions. No spectra were registered in the control samples with PO alone and in the PO/PTU mixture. EPR results are in fair agreement with those presented in obtained by the spectrophotometric method. These findings together indicate the interaction and redox transformations between NO and binuclear copper active center of PO (Scheme 1A and B).
Figure 6. EPR spectra of (a) native phenoloxidase (0.5 mM), (b) phenoloxidase (0.5 mM) incubated for 30 min with 10 mM PAPA/NO, (c) mixture b with 0.5 mM PTU added after incubation with PAPA/NO, (d) mixture of phenoloxidase (0.5 mM) and PTU (0.5 mM) incubated for 30 min with 10 mM PAPA/NO, (e) native phenoloxidase (0.5 mM) with 0.5 mM PTU. All samples were prepared in PBS buffer (pH 7.4) with 50% glycerol. T = 105 °K.
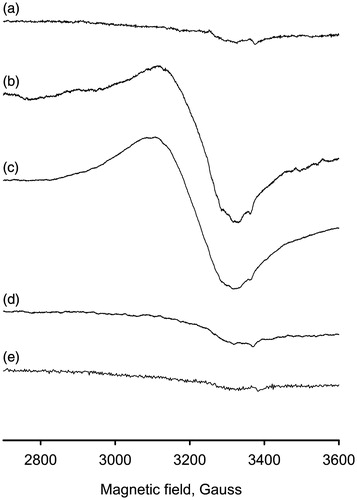
The effect of one-time high-dose treatment of PO by nitric oxide on DOPA oxidation
To test the effect of high local NO concentration on PO activity, we used gaseous nitric oxide solution. The aliquot of the NO-saturated solution was added once up to 25 μM to a PO solution in PBS. After 5 mins’ incubation, DOPA was added to the mixture and the kinetics of DOPA-chrome formation was recorded. shows the effect of bolus addition of NO on the rates of PO-mediated DOPA oxidation at different DOPA concentrations. A significant decrease of apparent PO activity was observed. This effect of NO can be assigned to the inhibition of PO via the oxidative damage of enzyme. The main candidates, which can aggressively attack the PO, are the nitric dioxide and peroxynitrite (ONOO−) at high concentrations. The first one is formed due to the oxidation of NO (Reactions 1 and 2), and ONOO− is the product of the reaction between nitric oxide and superoxide formed during the DOPA oxidationCitation32. An inhibitory effect of NO on DOPA oxidation by DEA NONOate at the high NO generation rate was also reportedCitation15. However, the authors proposed another mechanism of PO inhibition. They suggested that the nitrite derived from NO can interact with the met-form to yield a non-catalytic half-met-form of the enzyme, or NO directly interacts with met-form to produce a met-nitrite form, which is also non-catalytic.
The effect of nitric oxide on melanization process in haemocytes
The PO-mediated DOPA-chrome formation is the first stage of the melanization process that occurs particularly in the insect’s haemolymph in immune response. The PO is found in the immunocompetent cells of insects, haemocytes. PO catalyses the melanization of haemocytes attached to the surface of a parasiteCitation33.
We have tested the effect of nitric oxide on the melanization of the haemocytes of G. mellonella larvae. Experiments were carried out using the haemocytes isolated and fixed on slides. demonstrates the changes in the quantity of melanized cells in the samples treated with PAPA/NO (NO generation rate ∼ 33 μM/min) and with NO-gas solution (25 μM) in comparison with the control samples. In the case of PAPA/NO treatment, the quantity of haemocytes with melanization increased, whereas NO-gas solution inhibited melanization in haemocytes. Thus, the result obtained with insect haemocytes corresponds to our data obtained for the model system of mushroom PO.
Conclusions
The present investigation has demonstrated the dual effect of nitric oxide on the PO-mediated DOPA oxidation process. DOPA-chrome formation increased during continuous low-rate generation of NO. The mechanism of this effect involves oxidation of nitric oxide to dioxide at the active site of PO, which thereafter oxidizes DOPA to DOPA-chrome. On the contrary, a significant decrease of DOPA-chrome formation was observed in the case of bolus addition of NO solution to PO due to the inhibition of PO activity.
A similar, dual dose-dependent effect of NO on the melanization process was demonstrated for isolated haemocytes of G. mellonella larvae. It is reasonable to assume that these effects of NO have place in vivo. We suppose that enhancement of melanization by NO may occur upon encapsulation during immune response in haemolymph of insects. However, it has to be noted that NO-mediated acceleration of melanization may be achieved in the case of NO generation rate comparable with native PO-dependent DOPA oxidation rate. The inhibitory effect of NO requires an enormous local concentration of nitric oxide. This mechanism can be realized in the case of local burst of NO generation or intensive exogenous treatment with nitric oxide. Furthermore we suggest that inhibitory effect of NO on melanization process may protect from harmful action under severe conditions in which the overproduction of melanin results in human diseases.
Acknowledgements
The authors thank Dr. Uvarov M. for assistance in EPR experiment.
Declaration of interest
The authors report that they have no conflicts of interests. The work was supported by the Federal Fundamental Scientific Research Programme for 2013–2020 (VI.51.1.5 and V.44.1.3).
References
- Land EJ, Ramsden CA, Riley PA. Quinone chemistry and melanogenesis. Methods Enzymol 2004;378:88–109
- Ramsden CA, Riley PA. Tyrosinase: the four oxidation states of the active site and their relevance to enzymatic activation, oxidation and inactivation. Bioorg Med Chem 2014;22:2388–95
- Zhao X, Ferdig MT, Li J, Christensen BM. Biochemical pathway of melanotic encapsulation of Brugia malayi in the mosquito, Armigeres subalbatus. Dev Comp Immunol 1995;19:205–15
- Ashida M, Brey PT. Recent advances in research on insect prophenoloxidase cascade. In: Brey PT, Hultmark D, eds. Molecular mechanisms of immune responses in insects. London: Chapman & Hall; 1997:135–72
- Carton Y, Poirie M, Nappi AJ. Insect immune resistance to parasitoids. Insect Sci 2008;15:67–87
- Riley PA. Mechanistic aspects of the control of tyrosinase activity. Pigment Cell Res 1993;6:182–5
- Decker H, Schweikardt T, Nillius D, et al. Similar enzyme activation and catalysis in hemocyanins and tyrosinases. Gene 2007;398:183–91
- Nappi AJ, Christensen BM. Melanogenesis and associated cytotoxic reactions: applications to insect innate immunity. Insect Biochem Mol Biol 2005;35:443–59
- Carton Y, Nappi AJ. Drosophila cellular immunity against parasitoids. Parasitol Today (Regul Ed) 1997;13:218–27
- Jolley RL Jr, Evans LH, Makino N, Mason HS. Oxytyrosinase. J Biol Chem 1974;249:335–45
- Wilson MT, Torres J. Reactions of nitric oxide with copper containing oxidases; cytochrome c oxidase and laccase. IUBMB Life 2004;56:7–11
- Torres J, Wilson MT. The reactions of copper proteins with nitric oxide. Biochim Biophys Acta 1999;1411:310–22
- Romero-Graillet C, Aberdam E, Biagoli N, et al. Ultraviolet B radiation acts through the nitric oxide and cGMP signal transduction pathway to stimulate melanogenesis in human melanocytes. J Biol Chem 1996;271:28052–6
- Romero-Graillet C, Aberdam E, Clement M, et al. Nitric oxide produced by ultraviolet-irradiated keratinocytes stimulates melanogenesis. J Clin Invest 1997;99:635–42
- Nappi AJ, Vass E. The effects of nitric oxide on the oxidations of l-dopa and dopamine mediated by tyrosinase and peroxidase. J Biol Chem 2001;276:11214–22
- Keefer LK, Nims RW, Davies KM, Wink DA. "NONOates" (1-substituted diazen-1-ium-1,2-diolates) as nitric oxide donors: convenient nitric oxide dosage forms. Methods Enzymol 1996;268:281–93
- Woldman Y, Khramtsov VV, Grigor'ev IA, et al. Spin trapping of nitric oxide by nitronylnitroxides: measurement of the activity of no synthase from rat cerebellum. Biochem Biophys Res Commun 1994;202:195–203
- Zacharia IG, Deen WM. Diffusivity and solubility of nitric oxide in water and saline. Ann Biomed Eng 2005;33:214–22
- Mason HS. The chemistry of melanin; mechanism of the oxidation of dihydroxyphenylalanine by tyrosinase. J Biol Chem 1948;172:83–99
- Kojima H, Urano Y, Kikuchi K, et al. Fluorescent indicators for imaging nitric oxide production. Angew Chem Int Ed Engl 1999;38:3209–12
- Semenova AD, Glazachev YI, Slepneva IA, Glupov VV. Quantitative determination of nitric oxide production in haemocytes: nitrite reduction activity as a potential pathway of NO formation in haemolymph of Galleria mellonella larvae. Nitric Oxide 2014;37:46–52
- Kryukova NA, Dubovskiy IM, Chertkova EA, et al. The effect of Habrobracon hebetor venom on the activity of the prophenoloxidase system, the generation of reactive oxygen species and encapsulation in the haemolymph of Galleria mellonella larvae. J Insect Physiol 2011;57:796–800
- Wilcox DE, Porras AG, Hwang YT, et al. Substrate-analog binding to the coupled binuclear copper active-site in tyrosinase. J Am Chem Soc 1985;107:4015–27
- Uiterkamp AJ, Mason HS. Magnetic dipole-dipole coupled Cu(II) pairs in nitric oxide-treated tyrosinase: a structural relationship between the active sites of tyrosinase and hemocyanin. Proc Natl Acad Sci USA 1973;70:993–6
- Winkler ME, Lerch K, Solomon EI. Competitive inhibitor binding to the binuclear copper active-site in tyrosinase. J Am Chem Soc 1981;103:7001–3
- Kharitonov VG, Sundquist AR, Sharma VS. Kinetics of nitric oxide autoxidation in aqueous solution. J Biol Chem 1994;269:5881–3
- Namin SM, Nofallah S, Joshi MS, et al. Kinetic analysis of DAF-FM activation by NO: toward calibration of a NO-sensitive fluorescent dye. Nitric Oxide 2013;28:39–46
- Bernheim F, Bernheim MLC. The action of phenylthiocarbamide on tyrosinase. J Biol Chem 1942;145:213–17
- Ryazanova AD, Alekseev AA, Slepneva IA. The phenylthiourea is a competitive inhibitor of the enzymatic oxidation of DOPA by phenoloxidase. J Enzyme Inhib Med Chem 2012;27:78–83
- Klabunde T, Eicken C, Sacchettini JC, Krebs B. Crystal structure of a plant catechol oxidase containing a dicopper center. Nat Struct Biol 1998;5:1084–90
- Salvato B, Giacometti GM, Beltramini M, et al. The oxidation of Octopus vulgaris hemocyanin by nitrogen oxides. Biochemistry 1989;28:680–4
- Komarov DA, Slepneva IA, Glupov VV, Khramtsov VV. Superoxide and hydrogen peroxide formation during enzymatic oxidation of DOPA by phenoloxidase. Free Radic Res 2005;39:853–8
- Ling E, Yu XQ. Prophenoloxidase binds to the surface of hemocytes and is involved in hemocyte melanization in Manduca sexta. Insect Biochem Mol Biol 2005;35:1356–66