Abstract
Curcumin has multiple pharmacological effects, but it has poor stability. Complexation of curcumin with metals improves its stability. Here, the effects of vanadyl curcumin and vanadyl diacetylcurcumin on the function and structure of horseradish peroxidase enzyme were evaluated by spectroscopic techniques. Cytotoxic effect of the complexes was also assessed on MCF-7 breast cancer, bladder and LNCaP prostate carcinoma cell line. The results showed that the complexes improve catalytic activity of HRP, and also increase its tolerance against the oxidative condition. The result also indicated that the affinity of HRP for hydrogen peroxide substrate decreases, while the affinity increases for phenol substrate. Circular dichroism and fluorescence spectroscopies showed that compactness of the enzyme structure around the catalytic heme group and the distance between the heme group and tryptophan residue decreases after the binding. The antibacterial and cytotoxic results indicated that the complexes have anticancer potential, but they have no considerable antibacterial activity.
Introduction
Recently, many studies have been carried out on curcumin and curcuminoid complexes with metals, because of their potential as therapeutics in a wide variety of diseasesCitation1–3. Curcumin (diferuloylmethane), a polyphenol, is the main active component of the food flavor, turmeric (Curcuma longa)Citation1–3. It has a large variety of biological activities, including neuroprotectiveCitation4,Citation5, antidiabeticCitation6, cytotoxicCitation2,Citation7, antioxidant, antinflammatoryCitation8, antitumourCitation1–3, antimicrobialCitation9, anticancerCitation1–3, wound healingCitation10 and antiarthriticCitation2 properties. Several metallocomplexes of curcuminCitation11–15 such as vanadyl curcumin complexesCitation14,Citation15 have been synthesized, characterized and their biological activities evaluated. Anticancer activity and selective cytotoxicity, antiAlzheimer’s disease activity, and antioxidative/neuroprotective effects of metal curcumin complexes have been previously reportedCitation16.
Vanadium is a ubiquitous trace element that has biological function. The total content of vanadium ranges between 50 and 200 μg in the human body. Vanadium presents at very low doses, 0.01–1 μg, in each individual organ and is thought to play a significant role in many physiological processes. It is able to influence on signal transduction cascades with remarkable sensitivity at several regulatory points. In tissues, 10% of vanadium presents in the ionic form and about 90% is bound with proteins. Vanadium showed potential therapeutic value in treating diabetesCitation17–19. In addition, a number of complexes containing vanadium as a metal centers have reported to possess a wide spectrum of antitumor propertiesCitation20,Citation21. Vanadium is also known to have antioxidant propertiesCitation19.
Recently, in order to have advantage of the pharmacological properties of both curcumin and vanadyl, the metal ion can be complexed to curcumin. In addition, complexation of curcumin with metals has gained considerable attention in recent years for improvement of its stability. Low stability and limited solubility of curcumin in aqueous media decrease its bioavailability. Protection of curcumin from physical and chemical damages by the complexation increases its applications. Curcuminoids have a β-diketone moiety; thus they are able to form stable complexes with metal cationsCitation11–15,Citation22,Citation23.
There are some reports on the synthesize and characterization of curcumin and curcuminoid vanadyl complexesCitation14,Citation15. It has been previously reported that vanadyl curcumin is more effective for inhibiting synoviocyte proliferation, smooth muscle cell growth and mouse lymphoma cell growth than curcumin aloneCitation14. Mohammadi et al. synthesized vanadyl curcumin (VO(cur)2) and vanadyl diacetylcurcumin (VO(DAC)2), and investigated their chemical and biological propertiesCitation15.
The aim of the present study is trying to shed some light on the effects of two vanadyl curcuminoids complexes on the peroxidase enzyme. Here, we report an experimental study aimed to evaluate the effect of vanadyl curcumin and vanadyl diacetylcurcumin on the horseradish peroxidase (HRP) enzyme. Curcumin has been proven to inhibit numerous enzymes and some effects of curcumin are mediated through enzyme inhibitionCitation24–27. In this investigation, a peroxidase enzyme was chosen, because on one hand both vanadyl ion and curcumin have antioxidant properties and on the other hand, peroxidase enzymes contribute to antioxidant defense of organisms by removing hydrogen peroxide (H2O2). Peroxidases catalyze the breakdown of hydrogen peroxide, which is one of the reactive oxygen species and dangerous to the cellCitation28,Citation29. Accordingly, it is reasonable to expect that the antioxidant properties of curcumin and its derivatives may be attributed to their effects on the peroxidase enzymes.
HRP (donor: hydrogen peroxide oxidoreductase, E.C. 1.11.1.7), a plant peroxidase, has been studied for more than a century. It oxidizes a wide variety of substrates by hydrogen peroxide. HRP-C used in this investigation is a monomeric glycoprotein with molecular weight of 44 kDa. It contains eight N-linked carbohydrate chains and one noncovalently bound hemin moiety. It has also four disulfide bridges and two Ca2+ binding sites, and an extensive hydrogen bond networkCitation29,Citation30.
In this study, the enzymatic parameters of HRP such as Vmax (maximum rate of reaction), Km (Michaelis constant), kcat (turnover number), Ea (activation energy of the enzymatic reaction), ΔH# (enthalpy of activation), ΔS# (entropy of activation) and ΔG# (free energy of activation) were measured in the absence and presence of vanadyl curcumin and vanadyl diacetylcurcumin. Furthermore, the activity of the enzyme was measured at oxidative concentrations of hydrogen peroxide to evaluate effect of the complexes on oxidative stability of the enzyme. Hydrogen peroxide as a well-known oxidizing agent can inactivate enzymes by oxidizing the cysteine (–SH) or methionine residues (–SCH3)Citation31. Further aim of this work is also to evaluate structural change of the enzyme in the presence of the complexes.
In addition to investigating the enzymatic effects of the complexes, their antibacterial activity was evaluated. Curcumin has been subjected to a variety of antimicrobial investigations due to extensive traditional uses and low side effectsCitation32–35. Tajbakhsh et al. investigated antibacterial activity of indium curcumin complexes; their result indicated that the complexes have more antibacterial effect than curcumin itselfCitation36. In this study, antibacterial activity of vanadyl curcumin complexes was assessed against two bacteria species including Escherichia coli and Staphylococcus aureus by broth microdilution method.
In this investigation, anticancer effects of vanadyl curcumin and vanadyl diacetylcurcumin complexes were also evaluated. Curcumin blocks cancer cell growth in vitro and in vivo. It inhibits tumorigenesis and cell proliferation in a variety of cancer cell linesCitation1–3,Citation7. Mohammadi et al. evaluated cytotoxicity of vanadyl curcumin complexes by MTT (methyl thiazolyltetrazolium) assay. Testing of cell toxicity of the compounds in mouse lymphoma cell showed that the vanadyl complexes are remarkably potent than curcuminCitation15. Here, in continuing efforts toward the assessment of the anticancer activity of vanadyl curcumin complexes, the cytotoxic effect of these complexes was assessed on MCF-7 (C135) breast cancer, bladder (C5637) and LNCaP (C439) prostate carcinoma cell lines.
Material and methods
Materials
HRP (K16937816), Mueller–Hinton medium, hydrogen peroxide, MTT and trypan blue were purchased from Merck (Darmstadt, Germany). All materials for cell culture were purchased from GIBCO (Carlsbad, CA). All other materials were purchased from Sigma (St. Louis, MO). Vanadyl curcumin and vanadyl diacetylcurcumin were synthesized and purified by Mohammadi et al.Citation15.
Determination of the protein concentration and the enzymatic activity
Concentration of the enzyme was spectrophotometrically measured using an extinction coefficient of 102 mM−1 cm−1 at 403 nm. 4-Aminoantipyrine and hydrogen peroxide as color-generating substrates were used for the determination of the enzymatic assay by colorimetric methodCitation37. Six hundred microliters reaction mixture containing 1.25 mM 4-aminoantipyrine, 85 mM phenol and 0.85 mM hydrogen peroxide was prepared in phosphate buffer 100 mM, pH 6. Fifty microliters of the enzyme (0.001 mg/mL) was then added to the substrates solution, and after 3 s, the rate of color generation at 510 nm was measured by UV M501-Camspect spectrophotometer (UK). The rate of reaction was determined using the following equation:
(1)
Various concentrations of H2O2 in the range of 0.2–85 mM and the saturated concentrations of phenol and 4-aminoantipyrine were prepared to determine Km (Michaelis constant) value for H2O2. For determination of Km of phenol, the enzyme was assayed in the range of 0.013–0.78 mM phenol and the saturated concentrations of 4-aminoantipyrine and H2O2.
Determination of the activation energy and thermodynamic parameters of activation
The rate of enzymatic reaction was measured at the temperature ranged between 10 and 30 °C to determine the activation energy and thermodynamic parameters of activationCitation38. The Arrhenius plot was constructed from experimentally determined kcat values. The plot was used to calculate Ea as follows:
(2)
ΔG# was determined by analysis of the Eyring plot (ln(kcat/T) versus 1/T) as follows:
(3)
The activation enthalpy ΔH# and ΔS# at temperature T were calculated using the following equations:
(4)
(5)
Determination of the enzyme stability under the oxidative condition
In order to investigate the effect of oxidative condition on the enzyme activity, it was assayed at the high concentrations of H2O2. As noted, the optimum concentration of H2O2 as substrate is 0.85 mM; the higher concentrations of H2O2 cause to decrease in activity of the enzyme, because H2O2 is also an oxidizing agentCitation39. Accordingly, we assayed the enzyme in the range of 0.78–0.62 mM H2O2 to investigate the effect of complexes on the stability of HRP under the oxidative condition.
Circular dichroism
Circular dichroism measurements were performed on an Aviv Model 215 Circular dichroism Spectrometer (Lakewood, NJ) at room temperature. The spectra of near-UV and visible regions were recorded in a quartz cell of 1 cm path length. In all measurements, the enzyme concentration was 0.22 mg/mL. The molar ellipticity was determined asCitation40:
(6)
where c is the enzyme concentration in milligram per milliliter, l is the light path length in centimeters, MRW is the mean residue weight of HRP and θ is the observed ellipticity in degrees.
Fluorescence spectroscopy
Fluorescence spectra of the protein were obtained at room temperature using a Varian Cary Eclipse fluorescence spectrophotometer (Australia) in a quartz cell of 1 cm path length in 20 mM phosphate buffer, pH 6. The excitation wavelength was separately set at 280 and 295 nm to record the intrinsic fluorescence spectra of HRP. The synchronous fluorescence spectra were obtained using Δλ = 60 nm and Δλ = 15. Concentration of the protein was 0.05 mg/mL.
Cell culture and cytotoxicity assay
MCF-7 (C135) breast cancer, bladder (C5637) and LNCaP (C439) prostate carcinoma cell lines were cultured in RPMI (Roswell Park Memorial Institute) 1640 medium, supplemented with 10% fetal bovine serum and 50 IU/mL penicillin and 50 µg/mL streptomycin in a CO2 incubator at 37 °C. One hundred microliters of the cell suspension containing 106 cells/mL was added to each well of the plate. The desired concentrations of the complex were then added to the cell culture medium. Next, the plate was incubated at 37 °C for overnight. MTT solution (5 mg/mL) was then added to each well and the plates incubated for 4 h at 37 °C. Absorbance of the samples was measured at 630 nm with enzyme-linked immunosorbent assay (ELISA) plate reader, after the plate was spun down for 15 min at low speed, for dissolution of formazan crystalsCitation41.
Antibacterial susceptibility testing
Antibacterial activity of the complexes was investigated using dilution test methodCitation42. One of the bacterial colonies was selected and grown in 2 mL Mueller-Hinton broth (MHB) medium at 37 °C until the turbidity of 0.5 McFarland was achieved. Then, the stock solution of the complex was prepared in 20 mM phosphate buffer and poured into the sterile tube. Next, twofold serial dilutions were carried out. After that, the 0.5 McFarland standards was diluted 1000 times and 100 µL of the diluted suspension was poured into the sterilized tubes and all the tubes were incubated for 1 h at 25 °C. Finally, 100 µL sterile MHB medium was poured into each sterile tube and all the tubes were incubated overnight (12–16 h) at 35–37 °C. In each experiment, one positive control consisted of inoculums, buffer and MHB and one negative control consisted of buffer and MHB also were incubated. Minimal inhibitory concentration (MIC) was determined as the lowest concentration of the complexes displaying no visible growth in the tubes.
Results and discussion
Circular dichroism
Circular dichroism and fluorescence spectroscopy were used to evaluate structural changes of HRP contributing to interaction with the complexes. Near-UV CD spectra give information on the tertiary structure of proteins. In the visible region, the polypeptide chain has no absorbance, although heme, the catalytic group of HRP, absorbs visible light. Therefore, visible CD spectra of HRP give information on the tertiary structure of HRP around the heme catalytic center. It is noteworthy that free form of heme is optically inactive compound; while in asymmetric environment of the protein it becomes optically activeCitation40,Citation43,Citation44.
Near-UV and visible CD spectra of HRP in the absence and presence of 1:1 molar ratio of the complexes to the enzyme are presented in . As shown, HRP has a negative peak at 290 nm and a positive peak at 260 nm. Intensity of the negative peak remarkably increases in the presence of vanadyl diacetylcurcumin, probably indicating that the complex increases rigidity of the environment in vicinity of (some) Trp and Tyr residues. In addition, the negative peak shifts to the lower wavelengths; this blue shift may result from a decrease in polarity of the microenvironment around (some) Trp and Tyr residuesCitation43. As shown in , unlike the negative peak, intensity of the positive one at 260 nm decreases, probably indicating that rigidity of the environment in vicinity of (some) Phe residues increases in the presence of VO(DAC)2.
Figure 1. Circular dichroism spectra of HRP in absence (control) and presence of vanadyl curcumin (VO(cur)2) and vanadyl diacetylcurcumin (VO (DAC)3). The molar ratio of HRP to the both curcumin complexes is 1:1.
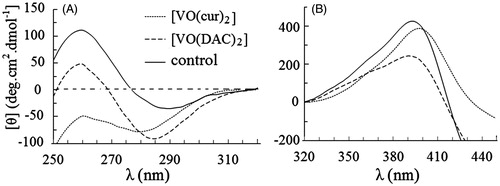
As shown in , in the presence of another complex, vanadyl diacetylcurcumin, the near UV CD spectrum of the protein is relatively monotonous implying considerable changes in the environmental asymmetry of aromatic amino acid residuesCitation43.
Circular dichroism spectra of the enzyme in the visible region are shown in . Obviously, intensity of the enzyme spectrum remarkably decreases upon interaction of the enzyme with vanadyl diacetylcurcumin. A slight decrease and meaningful red shift is also shown after binding of the enzyme to vanadyl curcumin. These changes indicate that the complexes influence on the heme microenvironment of HRP. It is more likely that an increase in the polarity and/or a decrease in rigidity of the microenvironment in the vicinity of the heme catalytic center occur in the presence of the complexesCitation43.
Fluorescence spectroscopy
Fluorescence spectroscopy is a sensitive technique capable of giving information about various aspects of the structure and dynamics of proteinsCitation44,Citation45. Intensity of intrinsic fluorescence of HRP is dependent on the structural change and/or the fluorescence energy transfer from tryptophan to heme. Heme quenches emission of Trp residueCitation46,Citation47. In the fluorescence measurements, HRP was excited at 280 and 295 nm. The fluorescence emission of the protein excited at 295 nm arises solely from the only one tryptophan (Trp117) residue of HRPCitation48, but the emission of the protein excited at 280 nm arises from Trp and Tyr residuesCitation44,Citation45.
Fluorescence spectra of HRP excited at 280 and 295 nm were obtained at the fixed concentration of the enzyme (1 µM) and various concentrations of vanadyl diacetylcurcumin () and vanadyl curcumin () in the range of 0–20 µM. As shown in , fluorescence intensity of the enzyme excited at 280 nm decreases with increasing concentration of vanadyl diacetylcurcumin. This change is also shown, when the enzyme is excited at 295 nm (). The intrinsic emission spectra of the enzyme in the presence of vanadyl curcumin are shown in . The quenching effect is observed again at the both exciting wavelengths, although the spectra are not quenched uninterruptedly upon binding to vanadyl curcumin; obviously, emission of the spectra is quenched till 7 µM vanadyl curcumin, intensity of the spectra then increases and λmax shifts to the lower wavelengths. The blue shift is more obvious, when the only Trp residue of HRP is selectively excited at 295 nm. The decrease in intensity of the spectra may occur as a consequence of the quenching effect of heme groupCitation46,Citation47. When the distance between the Trp residue and heme group reduces, the quenching effect of heme on the Trp emission is strengthened, causing to a decrease in the intensity of the spectra. The blue shift in λmax may result from the exposure of the Trp and/or Tyr residues to a more hydrophobic environmentCitation44,Citation45.
Figure 2. Intrinsic fluorescence spectra of HRP excited at 280 nm (A) and 295 nm (B) in 0–20 µM concentrations of vanadyl diacetylcurcumin. In the inset figures fluorescence intensity at λmax has been plotted against molar concentration of the complex.
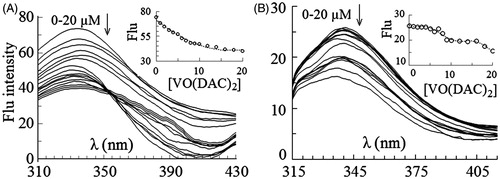
Figure 3. Intrinsic fluorescence spectra of HRP excited at 280 nm (A) and 295 nm (B) in 0–20 µM concentrations of vanadyl curcumin (B). In the inset figures fluorescence intensity at λmax has been plotted against molar concentration of the complex.
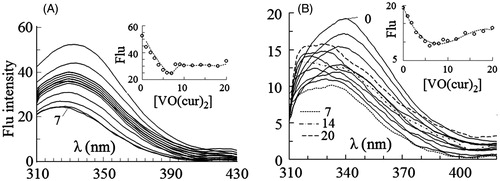
The change in conformation of HRP can be also demonstrated by synchronous fluorescence spectra. Synchronous fluorescence spectroscopy gives information about the structural changes of proteins, when either tyrosine or tryptophan present in polypeptide chain. The synchronous fluorescence gives the characteristic information of tyrosine and tryptophan residues at Δλ = 15 nm and Δλ = 60 nm, respectivelyCitation49.
Synchronous spectra for the excitation of tryptophan and tyrosine in the presence of vanadyl curcumin (Supplementary data, Figure 1S) showed a gradual decrease in the intensity upon addition of 1–7 µM vanadyl curcumin to the protein solution. From this point forward, the intensity of emission increases. At Δλ = 15, the change of intensity is concomitant with remarkable red shift, while at Δλ = 60, no meaningful shift is observed. This result agrees with the result of intrinsic fluorescence of the enzyme at the excitation of 280 and 295 nm; similar to the intrinsic fluorescence, the change in the synchronous spectra can be explained by the distance between heme group and Trp residue and the change in the microenvironment of Tyr and Trp residuesCitation45,Citation46. Synchronous spectra for vanadyl diacetylcurcumin (Supplementary data, Figure 2S), another complex, show that the intensity of synchronous spectra of both Trp and Tyr residues decreases after addition of the complex. A red shift is also observed for the spectra of Tyr residues, while no shift is shown in the spectra of Trp residue. This change probably indicates that the average environment of Tyr residues become relatively more hydrophilic after the enzyme binds to vanadyl diacetylcurcumin. It is also possible that the average accessible surface area of these residues increases after the interaction with vanadyl diacetylcurcuminCitation44,Citation45.
Effect of VO(cur)2 and VO(DAC)2 on the catalytic activity of HRP
In order to investigate the effect of complexes on the activity of HRP, the complex was mixed with the oxidizing substrate (phenol + 4-aminoantipyrine), hydrogen peroxide as the other substrate was then added and the rate of the enzymatic reaction was measured. Concentration of the complexes varied in the range of 0–10 µM. All experiments were carried out at phosphate buffer 100 mM, pH 6. This pH value was chosen for the experiments, because curcumin is unstable at neutral and basic pH and decomposes to the other compounds, but under acidic conditions, the degradation of curcumin is much slowerCitation22,Citation23. It is noteworthy that the optimum pH of HRP is also in the range of 6–6.5Citation50. The percentage of the enzyme activity at the various concentrations of the complexes is shown in . The enzyme activity without the complexes was considered 100%. Obviously, the activity of the enzyme significantly increases with increasing the concentrations of the both complexes. The result also indicates that vanadyl diacetylcurcumin is almost two times more effective than vanadyl curcumin; for example, the activity increases 23% and 40% at 1 µM concentration of vanadyl curcumin and vanadyl diacetylcurcumin, respectively.
The effect of VO(cur)2 and VO(DAC)2 on the stability of HRP under the oxidative condition
In order to evaluate the stability of HRP under the oxidative condition, the enzyme was assayed at various concentrations of H2O2 as an oxidative agent. H2O2 is also one of the substrate of HRP and reduced to H2O by the enzyme. As reported in the “Methods” section, the optimum concentration of H2O2 is 0.85 mM for the enzymatic assay of HRP. Our previous result demonstrated that the activity of the enzyme decreases above the optimum concentration of H2O2Citation39. It has been reported that H2O2 can oxidize the cysteine (–SH) or methionine residues (–SCH3), and inactivate enzymes by oxidizing their thiol groupsCitation31. Accordingly, we measured activity of the enzyme at the optimum concentration of H2O2 (0.78 mM) as the control and the higher concentrations of H2O2 in the range of 1.5–6.2 mM. The rate of the enzyme reaction versus concentration of H2O2 is presented in . The rate of reaction was considered as 100% at the optimum concentration of H2O2. As shown, activities of all samples exponentially decrease with increasing concentration of H2O2, although in the presence of the complexes, the enzyme is more stable under the oxidizing condition. Obviously, the remaining activity of the enzyme in the presence of the different concentrations of vanadyl curcumin () and vanadyl diacetylcurcumin () is more than that in the absence of the complexes.
Figure 5. The rate of reaction of HRP (V) at different concentrations of hydrogen peroxide in absences (0) and presence of 1–3 µM (1, 2 and 3) vanadyl curcumin (A) and vanadyl diacetylcurcumin (B). In the inset figures, the enzyme activity was considered 100% in the absence of the complex.
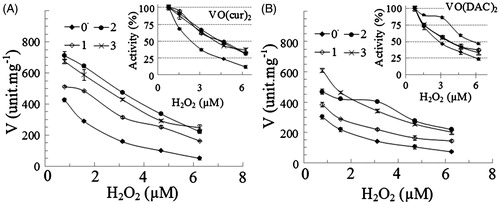
In order to evaluate the stability of the enzyme under the oxidative condition, the rate of enzymatic reaction was also measured at different concentrations of the both complexes in the range of 0–10 µM. In this experiment, oxidizing concentration of hydrogen peroxide was 4X (X is the optimum concentration of H2O2 for the enzymatic assay). The result is shown in .
Figure 6. Percentage of decrease in activity of HRP on the oxidative condition (at 3.12 µM concentration of H2O2 that is four-fold more than the optimum concentration of H2O2 for the enzymatic assay of HRP) at various concentrations of vanadyl curcumin (VO(cur)2) and vanadyl diacetylcurcumin (VO(DAC)2).
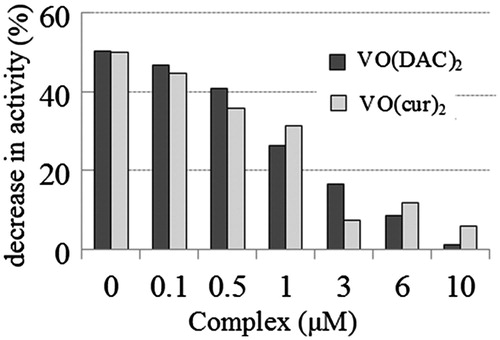
Percentage of decrease in activity was determined as:
(7)
where V4X and VX are the rate of enzymatic reaction at 4X and X concentration of H2O2 at each concentration of the complexes and V0 is the rate of enzymatic reaction in the absence of the complex. As shown, the activity of the enzyme decreases in the absence and presence of the complexes under the oxidative condition, but the complex reduces percentage of the decrease in activity of the enzyme under this condition.
IC50 [half minimal (50%) inhibitory concentration (IC)] of hydrogen peroxide was also calculated for quantitative analysis of the oxidative stability. It indicates how much of hydrogen peroxide is needed to inhibit the enzyme by half. The values of IC50 are presented in . As shown, the presence of the both complexes leads to increase in IC50 values.
Table 1. IC50 values (µM) of hydrogen peroxide in absence (control) and presence of 1, 2 and 3 µM vanadyl curcumin (VO(cur)2) and vanadyldiacetylcurcumin (VO(DAC)3).
The effect of VO(cur)2 and VO(DAC)2 on the kinetic parameters of the enzymatic reaction
The enzymatic parameters including Vmax, kcat and Km for the both substrates were measured in order to evaluate the effect of complexes on the kinetics of the enzymatic reaction. In order to calculate Km, the experimental data are plotted on Eadie–Hofstee plots (Supplementary data, Figures 1S and 2S)Citation51. The values of Vmax, kcat and Km at 0, 0.5 and 3 µM concentration of vanadyl curcumin and vanadyl diacetylcurcumin are, respectively, summarized in and . As presented, the rate of enzymatic reaction increases upon interaction of the enzyme with the both complexes. For example, Vmax and kcat values at 3 µM vanadyl curcumin and vanadyl diacetylcurcumin are, respectively, 1.4-and 1.7-fold higher than that of the enzyme without the complex. The Km values for hydrogen peroxide substrate increase suggesting that the affinity of the enzyme to hydrogen peroxide decreases due to its interaction with the complexesCitation51.
Table 2. Michaelis constant for H2O2 substrate (1 Km) and phenol substrate (2 Km), maximum rate of the enzymatic reaction (Vmax), catalytic rate constant (kcat) and catalytic efficiency (kcat/km) of HRP in the absence (control) and presence of 0.5 and 3 µM vanadyl curcumin (VO(cur)2).
Table 3. Michaelis constant for H2O2 substrate (1 Km) and phenol substrate (2 Km), maximum rate of the enzymatic reaction (Vmax), catalytic rate constant (kcat) and catalytic efficiency (kcat/Km) of HRP in the absence (control) and presence of 0.5 and 3 µM vanadyl diacetylcurcumin (VO(DAC)2).
As shown in and , unlike the hydrogen peroxide case, the Km value for phenol decreases due to the interaction of vanadyl curcumin and vanadyl diacetylcurcumin with the enzyme indicating higher affinity for the substrate towards the enzymeCitation51. As shown in tables, catalytic efficiency for hydrogen peroxide decreases, while it increases for phenol in the presence of the both complexes.
The effect of VO(cur)2 and VO(DAC)2 on the activation energy of enzymatic reaction and the enthalpy, entropy and free energy of activation
The thermodynamic parameters for the enzymatic reaction were calculatedCitation38 by measuring activities at different temperatures in the range of 10–30 °C. The Arrhenius and Eyring plots were plotted to calculate activation energy of the enzymatic reaction and free energy of activation (Supplementary data, Figure 5S). Activation energy of enzymatic reaction (Ea), free energy (ΔG#), enthalpy (ΔH#) and entropy (ΔS#) of activation are summarized in . As shown, Ea was found to be 57.1 kJ mol−1 without the complexes and 39.9 and 51.6 kJmol−1 in the presence of vanadyl curcumin and vanadyl diacetylcurcumin, respectively. The values indicate that Ea decreases upon interaction of the enzyme with both complexes; obviously, unlike vanadyl curcumin, vanadyl diacetylcurcumin has slight effect on the activation energy of the enzyme. The result demonstrates that the complexes accelerate velocity of the chemical reactions by stabilizing the transition state of the reactionCitation38. Therefore, the increase in the rate of enzymatic reaction (Vmax) observed in the presence of the complexes can be explained by the change of activation energy. The change in ΔG# and ΔH# is similar to Ea; a slight decrease upon the interaction with vanadyl diacetylcurcumin and noticeable decrease upon interaction with another vanadyl complex. The lower enthalpy value of the enzyme in the presence of the complexes especially vanadyl curcumin describes that formation of the transition state or activated complex between the enzyme and substrate is more efficient. Likewise, lower ΔG# value suggests that conversion of its transition complex into products is more spontaneous after the enzyme binds to the complexes. As shown in , the change in ΔS# is negligible, so the reaction is enthalpy driven.
Table 4. Activation energy of the enzymatic reaction (Ea), the free energy of activation (ΔG#), the enthalpy of activation (ΔH#) and the entropy of activation (ΔS#) in the absence (control) and presence of 3 µM vanadylcurcumin (VO(cur)2) and vanadyldiacetylcurcumin (VO(DAC)3).
Antibacterial activity and cytotoxicity of VO(cur)2 and VO(DAC)2
The cytotoxicity of vanadyl curcumin and vanadyl diacetylcurcumin toward MCF-7 (C135) breast cancer, bladder (C5637) and LNCaP (C439) prostate carcinoma cell lines was assayed using MTT assay. The percentage of viable cells at different concentrations of vanadyl curcumin and vanadyl diacetylcurcumin is depicted in and , respectively. Microscopic images of the three cell lines after treatment with vanadyl curcumin are also shown in Supplementary data (Figures 6S, 7S and 8S). Obviously, the both complexes can effectively inhibit proliferation of the cell lines. There is also no substantial difference between cytotoxic effect of vanadyl curcumin and vanadyldiacetyl curcumin. The both complexes, in general, induce a substantial loss of viability of bladder cell line.
Figure 7. Viability of MCF-7 (C135) breast cancer, bladder (C5637) and LNCaP (C439) prostate carcinoma cell lines at various concentrations of vanadyl curcumin (VO(cur)2) assayed by MTT method.
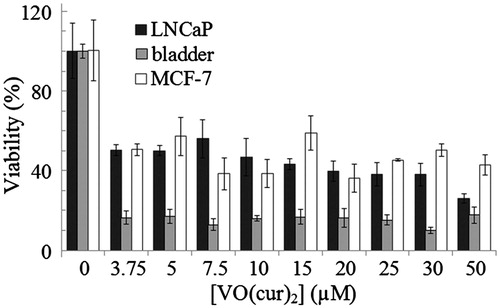
Figure 8. Viability of MCF-7 (C135) breast cancer, bladder (C5637) and LNCaP (C439) prostate carcinoma cell lines at various concentrations of vanadyl diacetylcurcumin (VO(DAC)2) assayed by MTT method.
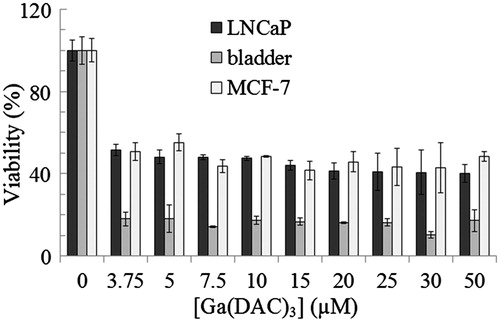
As noted, the antibacterial activity of the complexes was evaluated using dilution test method. Experiments were performed using Gram-negative and Gram-positive organisms, namely, E. coli (KCTC-1924) and S. aureus (KCTC-1916), respectively. The bacterial growth was estimated by measuring the turbidity (OD at 630 nm) of the samples (Supplementary data, Figures 9S and 10S). In addition, a standard loop was dipped into the tubes containing the samples; a loopful of the suspension was removed, subcultured to a Mueller-Hinton Agar plate and incubated at 37 °C for 24 h (Supplementary data, Figures 11S, 12S and 13S). The results indicate that vanadyl curcumin inhibits E. coli and S. aureus growth, but vanadyldiacetyl curcumin only inhibits E. coli growth in a dose-dependent manner; obviously, vanadyl diacetylcurcumin has no antibacterial activity against S. aureus. MIC values of vanadyl curcumin for the both organisms and vanadyl diacetylcurcumin for the Gram-negative one were found as 200 µM. Bacterial colonies were shown in the plates at 200 µM concentrations of the complexes indicating MBC is more than 200 µM. As is well known, MIC was defined at the lowest concentration that completely inhibited visible bacterial growth and the minimum bactericidal concentration (MBC) is defined as the lowest concentration of antibacterial agent at which no visible bacterial growth (colony) is observed after subculturingCitation42.
Conclusion
In this investigation, the effect of vanadyl curcumin and vanadyldiacetyl curcumin on the structure and function of a peroxidase enzyme was evaluated. The results indicated the ability of these compounds to not only activate peroxidation reaction in HRP, but also increase oxidative stability of the enzyme. Therefore, it can be concluded that the complexes on one hand improve the antioxidant activity of the peroxidase enzyme and therefore help the enzyme eliminate hydrogen peroxide more efficiently and on the other hand the complexes increase the activity under oxidative condition, so they prevent the loss of the enzyme activity even at oxidative concentrations of hydrogen peroxide. The both effects result in increasing the antioxidant efficiency of the peroxidase enzyme.
We carried out the experiments on HRP; whether vanadyl curcumin complexes can influence on the other peroxidase enzyme as well remains to be determined.
This investigation also presents the possibility that the complexes might serve as potential antitumor agents especially for bladder cancers, but they are not good antibacterial compounds, because their MIC values are too high.
Supplementary Material available online Supplementary Figures S1-S13
Supplementary_Figures_S1_S13.pdf
Download PDF (627.2 KB)Declaration of interest
Financial support given by Research Council of Institute for Advanced Studies in Basic Sciences is gratefully acknowledged.
References
- Goel A, Kunnumakkara AB, Aggarwal BB. Curcumin as “Curecumin”: from kitchen to clinic. Biochem Pharmacol 2010;75:787–809
- Maheshwari RK, Singh AK, Gaddipati J, Srimal RC. Multiple biological activities of curcumin: a short review. Life Sci 2006;78:2081–7
- Sharma RA, Gescher AJ, Steward WP. Curcumin: the story so far. Eur J Cancer 2005;41:1955–68
- Sunanda BPV, Latha K, Rammohan B, et al. Evaluation of the neuroprotective effects of curcumin (turmeric) against scopolamine induced cognitive impairment in mice. Int J Pharm Sci Rev Res 2014;6:133–6
- Zhao J, Zhao Y, Zheng W, et al. Neuroprotective effect of curcumin on transient focal cerebral ischemia in rats. Brain Res 2008;1229:224–32
- Chuengsamarn S, Rattanamongkolgul S, Luechapudiporn R, et al. Curcumin extract for prevention of type 2 diabetes. Diabetes Care 2012;35:2121–7
- Salem M, Rohani S, Gillies ER. Curcumin, a promising anticancer therapeutic: a review of its chemical properties, bioactivity and approaches to cancer cell delivery. R Soc Chem Adv 2014;4:10815–29
- Menon VP, Sudheer AR. Antioxidant and antiinflammatory properties of curcumin. Adv Exp Med Biol 2007;595:105–25
- Zorofchian Moghadamtousi S, Abdul Kadir H, Hassandarvish P, et al. A review on antibacterial, antiviral, and antifungal activity of curcumin. Biol Med Res Int 2014;2014:1–12
- Akbik D, Ghadiri M, Chrzanowski W, Rohanizadeh R. Curcumin as a wound healing agent. Life Sci 2014;116:1–7
- Vajragupta O, Boonchoong P, Berliner LJ. Manganese complexes of curcumin analogues: evaluation of hydroxyl radical scavenging ability, superoxide dismutase activity and stability towards hydrolysis. Free Radic Res 2004;38:303–14
- Barik A, Mishra B, Kunwar A, et al. Comparative study of copper(II)-curcumin complexes as superoxide dismutase mimics and free radical scavengers. Eur J Med Chem 2007;42:431–9
- Valentini A, Conforti F, Crispini A, et al. Synthesis, oxidant properties, and antitumoral effects of a heteroleptic palladium (II) complex of curcumin on human prostate cancer cells. J Med Chem 2008;52:484–91
- Thompson KH, Bohmerle K, Polishchuk E, et al. Complementary inhibition of synoviocyte, smooth muscle cell or mouse lymphoma cell proliferation by a vanadyl curcumin complex compared to curcumin alone. J Inorg Biochem 2004;98:2063–70
- Mohammadi K, Thompson KH, Patrick BO, et al. Synthesis and characterization of dual function vanadyl, gallium and indium curcumin complexes for medicinal applications. J Inorg Biochem 2005;99:2217–25
- Wanninger S, Lorenz V, Subhan A, Edelmann FK. Metal complexes of curcumin – synthetic strategies, structures and medicinal applications. Chem Soc Rev 2015;44:4986–5002
- Rehder D. Bioinorganic vanadium chemistry. 2nd ed. Hamburg: John Wiley & Sons; 2008
- Mukherjee B, Patra B, Mahapatra S, et al. Vanadium – an element of atypical biological significance. Toxicol Lett 2004;150:135–43
- Crans DC, Smee JJ, Gaidamauskas E, Yang L. The chemistry and biochemistry of vanadium and the biological activities exerted by vanadium compounds. Chem Rev 2004;104:849–902
- Novotny L, Kombian SB. Vanadium: possible use in cancer chemoprevention and therapy. J Can Res Updates 2014;3:97–102
- Kostova I. Titanium and vanadium complexes as anticancer agents. Anticancer Agents Med Chem 2009;9:827–42
- Wang YJ, Pan MH, Cheng AL, et al. Stability of curcumin in buffer solutions and characterization of its degradation products. J Pharm Biomed Anal 1997;15:1867–76
- Shen L, Ji HF. The pharmacology of curcumin: is it the degradation products. Trends Mol Med 2012;18:138–44
- Reddy S, Aggarwal BB. Curcumin is a noncompetitive and selective inhibitor of phosphorylase kinase. FEBS Lett 1994;341:19–22
- SkrzypczakJankun E, McCabe NP, Selman SH, Jankun J. Curcumin inhibits lipoxygenase by binding to its central cavity: theoretical and X-ray evidence. Int J Mol Med Sci 2000;6:521–7
- Sui Z, Salto R, Li J, et al. Inhibition of the HIV-1 and HIV-2 proteases by curcumin and curcumin boron complexes. Bioorg Med Chem 1993;1:415–22
- Shen L, Ji HF. Insights into the inhibition of xanthine oxidase by curcumin. Bioorg Med Chem Lett 2009;19:5990–3
- Ngo TT. Peroxidase in chemical and biochemical analysis. Anal Lett 2010;43:1572–87
- Veitch NC. Horseradish peroxidase: a modern view of a classic enzyme. Phytochemistry 2004;65:249–459
- Gajhede M, Schuller DJ, Henriksen A, et al. Crystal structure of horseradish peroxidase C at 2.15 Å resolution. Nat Struct Mol Biol 1997;4:1032–8
- Halliwell B, Gutteridge J. Free radicals in biology and medicine. 2nd ed. Oxford: Oxford University Press; 1989
- Liu CH, Huang HY. Antimicrobial activity of curcumin-loaded myristic acid microemulsions against Staphylococcus epidermidis. Chem Pharm Bull 2012;60:1118–24
- Niamsa N, Sittiwet C. Antimicrobial activity of Curcuma longa aqueous extract. J Pharmacol Toxicol 2009;4:173–7
- Lawhavinit OA, Kongkathip N, Kongkathip B. Antimicrobial activity of curcuminoids from Curcuma longa L. on pathogenic bacteria of shrimp and chicken. Witthayasan Kasetsat 2010;44:364–71
- Mun SH, Joung DK, Kim YS, et al. Synergistic antibacterial effect of curcumin against methicillin-resistant Staphylococcus aureus. Phytomedicine 2013;15:714–18
- Tajbakhsh S, Mohammadi K, Deilami I, et al. Antibacterial activity of indium curcumin and indium diacetylcurcumin. Afr J Biotechnol 2008;7:3832–5
- Trinder P. Determination of glucose in blood using glucose oxidase with an alternative oxygen acceptor. Ann Clin Biochem 1969;6:24–7
- Low PS, Bada JL, Somero GN. Temperature adaptation of enzymes: roles of the free energy, the enthalpy, and the entropy of activation. Proc Natl Acad Sci 1973;70:430–2
- Hassani L, Nourozi R. Modification of lysine residues of horseradish peroxidase and its effect on stability and structure of the enzyme. Appl Biochem Biotechnol 2014;172:3558–69
- Kelly SM, Price NC. The use of circular dichroism in the investigation of protein structure and function. Curr Protein Pept Sci 2000;1:349–84
- Mosmann T. Rapid colorimetric assay for cellular growth and survival: application to proliferation and cytotoxicity assays. J Immunol Methods 1983;65:55–63
- Reller LB, Weinstein M, Jorgensen JH, Ferraro MJ. Antimicrobial susceptibility testing: a review of general principles and contemporary practices. Clin Infect Dis 2009;49:1749–55
- Kelly SM, Jess TJ, Price NC. How to study proteins by circular dichroism. Biochem Biochim Biophys Acta 2005;1751:119–39
- Campbell ID, Dwek RA. Biological spectroscopy. Menlo Park (CA): Benjamin Cummings Pub. Co.; 1984
- Joseph R, Lakowicz JR. Principles of fluorescence spectroscopy. 3rd ed. New York: Springer; 2006
- Tsaprailis G, Chan DWS, English AM. Conformational states in denaturants of cytochrome c and horseradish peroxidases examined by fluorescence and circular dichroism. Biochemistry 1998;37:2004–16
- Wu BP, Wen Q, Xu H, Yang Z. Insights into the impact of deep eutectic solvents on horseradish peroxidase: activity, stability and structure. J Mol Catal B Enzym 2014;101:101–7
- Welinder KG. Amino acid sequence studies of horseradish peroxidase. Amino and carboxyl termini, cyanogen bromide and tryptic fragments, the complete sequence, and some structural characteristics of horseradish peroxidase C. Eur J Biochem 1979;96:483–502
- Bhogale A, Patel N, Mariam J, et al. Comprehensive studies on the interaction of copper nanoparticles with bovine serum albumin using various spectroscopies. Coll Surf B: Biointerfaces 2014;113:276–84
- Schomberg D, Salzmann M, Stephan D. Enzyme handbook 7. Berlin: Springer; 1994
- Copeland RA. Enzymes, A practical introduction to structure, mechanism and data analysis. 2nd ed. New York: Wiley-VCH; 2004