Abstract
Malassezia yeasts are almost exclusively the single eukaryotic members of the fungal flora of the skin. Malassezia globosa and Malassezia restricta are found on the skin of practically all humans. Malassezia globosa is highly implicated in the pathogenesis of dandruff and its genome encodes for only one carbonic anhydrases (CAs, EC 4.2.1.1) belonging to the β-class (MgCA). It has been indeed demonstrated that in many pathogenic microorganisms, CAs are essential for their life cycle and their inhibition can lead to growth impairment and defects. In the previous work, the recombinant MgCA was investigated for its inhibition profile with sulfonamides, which in models of dandruff infection were able to protect animals from the fungal infection, allowing us to propose this enzyme as a new antidandruff target. MgCA was cloned as GST-fusion protein, but the yield was rather low and the protein was often found in inclusion bodies. Here, we propose an alternative procedure consisting in cloning the recombinant MgCA as His-Tag fusion protein. This procedure resulted in a good method to express and purify the active recombinant MgCA, and the protein recovery was better with respect to that used for preparing MG-CA (β-CA cloned as GST-fusion protein).
Introduction
Carbon dioxide (CO2) and its hydration product bicarbonate () are substrates and products of various metabolic reactions in the cells of different living organismsCitation1–7. CO2 is the final product of the respiration in all domains of life, from microorganisms to mammalsCitation8–10; bicarbonate (
) is a substrate for several carboxylating enzymes involved in biosynthetic pathways such as biosynthesis of fatty acids, amino acids and nucleotidesCitation11–14. The interconversion of CO2 and
is spontaneously balanced to maintain the equilibrium between dissolved inorganic CO2, carbonic acid (H2CO3), bicarbonate (
) and carbonate (
). Almost, all life on earth depend on the production of sugars from sunlight and CO2 (photosynthesis) and the metabolic breakdown (respiration) of those sugars to produce the energy needed for movement, growth and reproductionCitation8,Citation9,Citation14,Citation15. The uncatalyzed hydration–dehydration reaction CO2 + H2O ⇄
+ H+ is slow at physiological pH and thus, in biological systems, the reaction is accelerated by enzymatic catalysts, called carbonic anhydrases (CAs, EC 4.2.1.1). The conversion of CO2 to bicarbonate and protons is a physiologically relevant reaction in all life kingdomsCitation8,10,14,16–18. Virtually, CA isozymes have been found in all mammalian tissues and cell types, acting as CO2 transporter or involving in other physiological processes. CAs are also found in plants, fungi, algae, protozoa, cyanobacteria and bacteriaCitation8,19–25. Six different, genetically distinct CA families are known to date: α-, β-, γ-, δ-, ζ- and η-CAsCitation8,Citation20,Citation22. Many representatives of all these enzyme classes have been crystallized and characterized in detail. α-CAs are normally monomers and rarely dimers; β-CAs are dimers, tetramers or octamers; γ-CAs are trimers. Only the ζ-CA crystallized has three slightly different active sites on the same polypeptide chainCitation26, whereas no X-ray crystal structures of δ- and η-CAs are available up to now. CAs contain Zn2+ in their active site, coordinated by three histidine residues and a water molecule/hydroxide ion (in the α- and γ-CAs) or two Cys and one His residues (in the β class), with the fourth ligand being a water molecule/hydroxide ionCitation22,Citation23,Citation27. In the η-CAs, the coordination pattern of the Zn(II) ion is totally different from the other classes, with one Gln and two His acting as ligandsCitation21.
It has been demonstrated that in many pathogenic microorganisms (bacteria, fungi and protozoa), CAs are essential for their life cycleCitation2 and their inhibition can lead to growth impairment and defectsCitation22. CAs are abundant in fungiCitation22, but these enzymes have only recently been characterized in detail. Moreover, the physiological concentrations of CO2/ induce prominent virulence in Candida albicans (filamentation) and Candida glabrata or Cryptococcus neoformans (capsule biosynthesis) through direct activation of the fungal adenylyl cyclase, which is involved in the fungal differentiationCitation13,28–34. These findings suggest a link between cAMP signaling and CO2/
sensing capable of influencing the fungal metabolism and pathogenesisCitation33–36. Nonpathogenic and pathogenic fungi, such as Saccharomyces cerevisiae, C. albicans and C. glabrata, present only a single copy of CA belonging to a β-CA class; while in other fungi it is possible to find multiple copies of β-CA- and α-CA-encoding genesCitation29,37–39. Candida albicans CA, CaNce103, and the analogous CA, CgNce103, encoded by the genome of C. glabrata, are essential for pathogenesis of these fungi in niches where the available CO2 is limited (e.g. the skin)Citation29,Citation32,Citation33. Again, the CA, named Can2, identified in the genome of C. neoformans is essential for its growthCitation29,31–33,40.
Malassezia yeasts are almost exclusively the single eukaryotic members of the microbial flora of the skin. Malassezia have been recognized for more than 150 yearsCitation41,Citation42 as members of the human cutaneous flora and etiologic agents of certain skin diseases. At the begining of 1800, it was noted that yeast cells and filaments were present in the skin scales of patients with pityriasis versicolorCitation41,Citation42, whereas yeast cells, but no filaments, were observed in scales from healthy scalp, seborrheic dermatitis scalp, and dandruff. Dandruff is a frequent pathological skin condition confined to the scalp and is characterized by flaking with minimal to absent inflammation. Malassezia genus includes seven species, the three former taxa (M. furfur, M. pachydermatis, and M. sympodialis) and four new taxa (M. globosa, M. obtusa, M. restricta, and M. slooffiae)Citation43. With the exception of M. pachydermatis, all species afore-mentioned require lipids for their growthCitation44. Moreover, M. globosa and M. restricta are found on the skin of practically all humansCitation44. It has been demonstrated that oleic acid responsible of the sebum of the scalp is produced from the hydrolysis of triglycerides by M. globosa lipases. These data highly implicate M. globosa in the pathogenesis of dandruff. Similar to the above-mentioned fungi/yeasts, M. globosa genome contains only one β-CA, denominated MgCACitation22,Citation45,Citation46. This enzyme has been cloned and investigated several years ago for its inhibition profile with sulfonamides, which in models of dandruff infection were able to protect animals from the fungal infection, allowing us to propose this enzyme as a new antidandruff targetCitation46. In the previous work, a chimeric MgCA was produced having at the N-terminal the GST amino acid sequence, but the MgCA yield was rather low and the protein was often found in inclusion bodiesCitation46.
Here, we propose an alternative procedure for the preparation of recombinant MgCA encoded in the genome of M. globosa, the yeast responsible for dandruff, following a different cloning strategy compared with that described by Hewitson et al.Citation46. We propose to use a synthetic gene encoding MgCA to which a tag containing six His residues has been attached at the amino terminal part for allowing the purification with Ni(II)-based affinity columns. The yield and purity of MgCA obtained in this way was better compared with that of the earlier paperCitation44.
Materials and methods
Gene identification
The identification of the gene encoding for M. globosa β-CA (MgCA) was performed at the link http://www.ncbi.nlm.nih.gov/genome/selecting the genome of “M. globosa”. The β-CA gene of M. globosa (accession number: XP_001730815.1) was identified running the “BLAST” program and using the nucleotide sequences of β-CAs as query sequenceCitation47,Citation48.
Construct preparation, protein expression and purification
The GeneArt Company, specialized in gene synthesis, designed the synthetic M. globosa gene encoding for the β-CA, and containing 4 base pair sequences (CACC) necessary for directional cloning at the 5′ end of the MgCA gene. The fragment was subsequently cloned into the expression vector pET100/D-TOPO (Invitrogen, Carlsbad, CA), creating the plasmid pET100D-Topo/MgCA. In order to confirm the integrity of the M. globosa gene and the fact that no errors occurred at the ligation sites, the vector containing the fragment was sequenced. Competent Escherichia coli BL21 (DE3) Codon Plus cells were transformed with pET100/D-Topo/MgCA, grown at 37 °C, induced with 1 mM IPTG. Zn(SO4) was added after 30 min and after additional growth for 3 h, cells were harvested and disrupted by sonication at 4 °C in 20 mM buffer phosphate, pH 8.0. Following sonication, the sample was centrifuged at 1200×g at 4 °C for 30 min. The supernatant was dialyzed against 0.02M phosphate buffer (pH 8.0) containing 0.01 M imidazole and 0.5M NaCl at 4 °C and loaded onto a His-select HF Nickel affinity column (1.0 by 1.0 cm, GE Healthcare, Little Chalfont, Buckinghamshire, UK). The column was equilibrated with 0.02M phosphate buffer (pH 8.0) containing 0.01 M imidazole and 0.5M NaCl at a flow rate of 1.0 ml/min. The VchCAβ was eluted with 0.02M phosphate buffer (pH 8.0) containing 0.5 M NaCl and 0.25 mM imidazole at a flow rate of 1.0 ml/min. Active fractions (1 ml) were collected and combined for a total volume of 5 ml. Subsequently, they were dialyzed, concentrated and analyzed by SDS-PAGE. At this stage of purification, the enzyme was at least 95% pure and the obtained recovery was of 0.03 mg of the recombinant yeast MgCA.
Sequence analysis
Multialignment of amino acid sequences was performed using the program MUSCLE (MUltiple Sequence Comparison by Log-Expectation), a new computer program for creating multiple alignments of protein sequenceCitation49.
SDS-PAGE
Sodium dodecyl sulfate (SDS)–polyacrylamide gel electrophoresis (PAGE) was performed as described by Laemmli using 12% gelsCitation50.
Protonography
Wells of 12% SDS-gel were loaded with bCA and MgCA mixed with loading buffer without 2-mercaptoethanol and without boiling the samples, in order to avoid protein denaturation. The gel was run at 180V until the dye front ran off the gel. Following the electrophoresis, the 12% SDS-gel was subject to protonography to detect the bCA and MgCA hydratase activity on the gel as described by Capasso and coworkersCitation3,Citation19,Citation20.
Enzyme kinetics
An Applied Photophysics stopped-flow instrument has been used for assaying the CA catalyzed CO2 hydration activityCitation51. Bromothymol blue (at a concentration of 0.2 mM) has been used as an indicator, working at the absorbance maximum of 557 nm, with 10–20 mM TRIS (pH 8.3) as buffer, and 20 mM Na2SO4 for maintaining constant ionic strength (this anion is not inhibitory and has a KI> 200 mM against this enzyme), following the initial rates of the CA-catalyzed CO2 hydration reaction for a period of 10–100 s. The CO2 concentrations ranged from 1.7 to 17 mM for the determination of the kinetic parameters and inhibition constants. For each measurement at least six traces of the initial 5–10% of the reaction have been used for determining the initial velocity. The uncatalyzed rates were determined in the same manner and subtracted from the total observed rates. Stock solutions of inhibitor (1–10 mM) were prepared in distilled-deionized water and dilutions up to 0.01 μM were done thereafter with distilled-deionized water. Inhibitor and enzyme solutions were preincubated together for 15 min at room temperature prior to assay, in order to allow for the formation of the E–I complex. The inhibition constants were obtained by non-linear least-squares methods using the Cheng–Prusoff equation whereas the kinetic parameters for the uninhibited enzymes from the Lineweaver–Burk plots, as reported earlier, and represent the mean from at least three different determinations.
Results and discussion
The full nucleotide sequence encoding for MgCA showed an open-reading frame of 241 residues polypeptide chain which contained all the typical features of a β-CA, including the three residues that are involved in the catalytic mechanism of the enzyme (two cysteines and one histidine, see ). The predicted molecular mass of the enzyme from its amino acid sequence is of 26.98 kDa. We performed a BLAST search to compare MgCA amino acid sequence to other protein sequences present in the data bank. The result of BLAST analysis showed that the first 50 top-ranking library sequences were represented by CAs from fungi and bacteria (). Intriguingly, MgCA showed 77% identity with the β-CA from M. sympodialis, the only malasseziacea present in the top raking list generated by BLAST program (). Malassezia sympodialis has been reported as the most frequent skin-colonizing yeast in both patients with atopic eczema, as well as in healthy individualsCitation43,Citation44. It has also been associated with folliculitis and catheter-related infectionsCitation43,Citation44. In animals, it has caused otitis externa (in cats), whereas it is a known commensal of swineCitation52,Citation53.
Figure 1. Nucleotide and translated amino acid (bold letters) sequences of MgCA. The open reading frame is indicated by capital letters. Zinc ligands are indicated in red, while the catalytic dyad involved in the activation of the zinc-coordinated water in blue. .
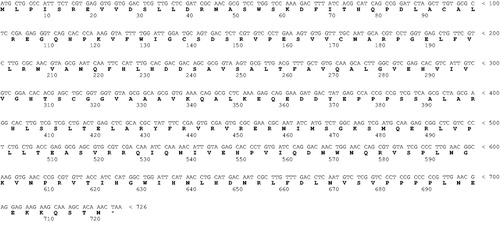
Figure 2. Blast output reporting the first 33 sequences. By going down the list, it is possible to see less than perfect matches, slowly degrading as the corresponding score decreases and the E value increases. The E value is an assessment of the statistical significance of the score. E value close to 1 is a warning that the alignment is not reliable.

The recombinant MgCA was isolated and purified to homogeneity at room temperature from E. coli (DE3) codon plus cell extract. Carbonic anhydrase activity was recovered in the soluble fraction of the cell extract obtained after sonication and centrifugation. Using the affinity column (His-select HF Nickel affinity gel), MgCA was purified to apparent homogeneity, as indicated by SDS–PAGE (, lane B). The analysis by SDS–PAGE of MgCA showed a band of about 29 kDa (monomeric form) under reducing condition (). The recovery of purified protein concentration was 1 mg of purified protein using the cloning, expression and purification aforementioned and starting from 2 L of bacterial culture.
Figure 3. SDS-PAGE of the recombinant MgCA purified from E. coli codon plus cells. Lane A, molecular markers, M.W. starting from the top: 250, 150, 100, 75, 50, 37, 25 and 20; Lane B, purified SspCA from His-tag affinity column.
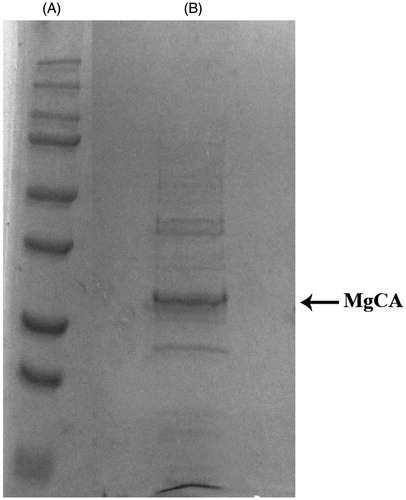
Using the stopped-flow technique, the kinetic parameters were determined for the newly purified recombinant MgCA using CO2 as a substrate. MgCA showed a significant catalytic activity, with a kcat of 9.2 × 105 s−1 and a kcat/Km of 8.3 × 107 M–Citation1 × s − Citation1. shows a comparison of the kinetic parameters of MgCA with those of MG-CA (β-CA cloned as GST-fusion protein)Citation46, hCA I and II (α-CA from Homo sapiens, isoform I and II, respectively) and Can2 (β-CA from C. neoformans). It may be observed that MgCA and MG-CA showed kinetic parameters about 2 times higher than the other β-CA reported in (Can2), and four times higher than the human α-CA isoform I. Moreover, data from show that the MgCA cloned as His-fusion protein has kinetic data similar to those of the GST-fusion protein reported earlier, but the activity of the His-tagged MgCA is better. It is interesting to note that both proteins obtained with two different cloning strategies are not affected by acetazolamide inhibition. Intriguingly, this lack of effectiveness of AAZ for inhibiting a β-class enzyme has been observed only for Cab, an enzyme isolated and characterized from the Archaeon Methanobacterium thermoautotrophicum.
Table 1. Kinetic parameters for the CO2 hydration reaction catalyzed by MG-CA (GST-fusion protein), MgCA (His-fusion protein), hCA I and II (α-CA from H. sapiens, isoform I and II, respectively) and Can2 (β-CA from C. neoformans).
The hydratase activity of MgCA on the SDS–PAGE gel was investigated and compared with that obtained for bovine bCA (α-CA). The gels were run under denaturing and non-reducing conditions. The protonogram, shown in , was obtained loading on the SDS–PAGE samples of MgCA and bCA at 10 μg/well. As described in the experimental section, the protonography is based on monitoring the pH variation in the gel due to the CA-catalyzed conversion of CO2 to bicarbonate and protons. The protonogram was thereafter stained with bromothymol blue, which is a widely used pH indicator. This dye appears blue in its deprotonated form, while its color changes to yellow in the protonated form. Thus, the production of H+ ions during the CO2 hydration reaction, due to the CA hydratase activity, lowers the pH of the solution until the color transition point of the dye is reached, that is, at pH 6.8. The developed protonogram showed a yellow band at a molecular weight of 29 kDa corresponding to the β-hydrates activity of MgCA (). The protonogram showed only one band corresponding to the monomer of MgCA (29 kDa). As described in the literature, β-CA fold resembles neither that of α-CA nor that of γ-CA. In the β-CAs, pair of monomers are joined to form an active enzymeCitation54. By using the protonogram, we observed a yellow band corresponding to the β-monomer position. This is possible because following the electrophoresis, SDS was removed from the gel. This procedure potentially led to the rearrangement of β-CA monomers in the gel, reconstituting the active dimeric form of the enzyme. β-CA activity corresponding to the dimeric band is absent in the protonogram because the concentration of SDS used in the loading buffer determines the dissociation of the MgCA dimer.
Figure 4. MgCA protonogram. The yellow bands correspond to the hydratase activity on the gel responsible for the drop of pH from 8.2 to the transition point of the dye. Incubation time was of 60 s. β-CA is present in the monomeric (29 kDa) form (see text for details). Lane A, molecular markers, M.W. starting from the top: 250, 150, 100, 75, 50, 37, 25 and 20 kDa; Lane B: MgCA; Lane C: commercial bovine CA (bCA).
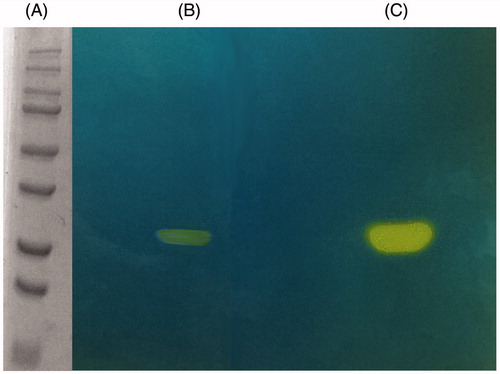
Conclusion
The results of this study are significant, because they establish that the MgCA fusion protein cloned as His-Tag fusion protein is a good method to express and purify the active recombinant protein; the yield and purification efficiency of MgCA fusion protein by affinity column (His-select HF Nickel affinity gel) is better compared with that used for preparing MG-CA (β-CA cloned as GST-fusion protein)Citation46; the CO2 hydratase activity by using protonography allows us to speculate that this technique may also be extended to enzymes belonging to other CA classes such as the β-CA (MgCA) from M. globosa.
Declaration of interest
We thank the Distinguished Scientist Fellowship Program (DSFP) at KSU for funding this project. This work was also financed in part by an FP7 EU project (Dynano). The authors report no conflict of interest.
References
- Capasso C, Supuran CT. An overview of the selectivity and efficiency of the bacterial carbonic anhydrase inhibitors. Curr Med Chem 2015;22:2130–9
- Capasso C, Supuran CT. An overview of the alpha-, beta- and gamma-carbonic anhydrases from bacteria: can bacterial carbonic anhydrases shed new light on evolution of bacteria? J Enzyme Inhib Med Chem 2015;30:325–32
- De Luca V, Del Prete S, Supuran CT, Capasso C. Protonography, a new technique for the analysis of carbonic anhydrase activity. J Enzyme Inhib Med Chem 2015;30:277–82
- Supuran CT, Winum JY. Carbonic anhydrase IX inhibitors in cancer therapy: an update. Future Med Chem 2015;7:1407–14
- Vullo D, De Luca V, Del Prete S, et al. Sulfonamide inhibition studies of the gamma-carbonic anhydrase from the Antarctic cyanobacterium Nostoc commune. Bioorg Med Chem 2015;23:1728–34
- Neri D, Supuran CT. Interfering with pH regulation in tumours as a therapeutic strategy. Nat Rev Drug Discov 2011;10:767–77
- Supuran CT. Carbonic anhydrases: novel therapeutic applications for inhibitors and activators. Nat Rev Drug Discov 2008;7:168–81
- Supuran CT, Capasso C. The η-class carbonic anhydrases as drug targets for antimalarial agents. Expert Opin Ther Targets 2015;19:551–63
- Ozensoy Guler O, Capasso C, Supuran CTA. Magnificent enzyme superfamily: carbonic anhydrases, their purification and characterization. J Enzyme Inhib Med Chem 2015. [Epub ahead of print]. doi: 10.3109/14756366.2015.1059333
- Carta F, Supuran CT, Scozzafava A. Sulfonamides and their isosters as carbonic anhydrase inhibitors. Future Med Chem 2014;6:1149–65
- De Monte C, Carradori S, Gentili A, et al. Dual cyclooxygenase and carbonic anhydrase inhibition by nonsteroidal anti-inflammatory drugs for the treatment of cancer. Curr Med Chem 2015;22:2812–18
- Migliardini F, De Luca V, Carginale V, et al. Biomimetic CO2 capture using a highly thermostable bacterial alpha-carbonic anhydrase immobilized on a polyurethane foam. J Enzyme Inhib Med Chem 2014;29:146–50
- Cottier F, Leewattanapasuk W, Kemp LR, et al. Carbonic anhydrase regulation and CO(2) sensing in the fungal pathogen Candida glabrata involves a novel Rca1p ortholog. Bioorg Med Chem 2013;21:1549–54
- Capasso C, Supuran CT. Anti-infective carbonic anhydrase inhibitors: a patent and literature review. Expert Opin Ther Pat 2013;23:693–704
- Capasso C, Supuran CT. Sulfa and trimethoprim-like drugs – antimetabolites acting as carbonic anhydrase, dihydropteroate synthase and dihydrofolate reductase inhibitors. J Enzyme Inhib Med Chem 2014;29:379–87
- Supuran CT, Winum JY. Designing carbonic anhydrase inhibitors for the treatment of breast cancer. Expert Opin Drug Discov 2015;10:591–7
- McKenna R, Supuran CT. Carbonic anhydrase inhibitors drug design. Subcell Biochem 2014;75:291–323
- Supuran CT. Carbonic anhydrase inhibitors: an editorial. Expert Opin Ther Pat 2013;23:677–9
- Del Prete SD, Luca V, Supuran CT, Capasso C. Protonography, a technique applicable for the analysis of eta-carbonic anhydrase activity. J Enzyme Inhib Med Chem 2015. [Epub ahead of print]. doi: 10.3109/14756366.2014.990963
- Del Prete S, De Luca V, Iandolo E, et al. Protonography, a powerful tool for analyzing the activity and the oligomeric state of the γ-carbonic anhydrase identified in the genome of Porphyromonas gingivalis. Bioorg Med Chem 2015;23:3747–50
- De Simone G, Di Fiore A, Capasso C, Supuran CT. The zinc coordination pattern in the η-carbonic anhydrase from Plasmodium falciparum is different from all other carbonic anhydrase genetic families. Bioorg Med Chem Lett 2015;25:1385–9
- Capasso C, Supuran CT. Bacterial, fungal and protozoan carbonic anhydrases as drug targets. Expert Opin Ther Targets 2015. [Epub ahead of print]. doi: 10.1517/14728222.2015.1067685
- Del Prete S, Vullo D, Fisher GM, et al. Discovery of a new family of carbonic anhydrases in the malaria pathogen Plasmodium falciparum-the η-carbonic anhydrases. Bioorg Med Chem Lett 2014;24:4389–96
- Supuran CT. Carbonic anhydrase inhibitors. Bioorg Med Chem Lett 2010;20:3467–74
- Supuran CT. Carbonic anhydrases-an overview. Curr Pharm Des 2008;14:603–14
- Alterio V, Langella E, Viparelli F, et al. Structural and inhibition insights into carbonic anhydrase CDCA1 from the marine diatom Thalassiosira weissflogii. Biochimie 2012;94:1232–41
- Lehneck R, Neumann P, Vullo D, et al. Crystal structures of two tetrameric β-carbonic anhydrases from the filamentous ascomycete Sordaria macrospora. FEBS J 2014;281:1759–72
- Sechi M, Innocenti A, Pala N, et al. Inhibition of alpha-class cytosolic human carbonic anhydrases I, II, IX and XII, and beta-class fungal enzymes by carboxylic acids and their derivatives: new isoform-I selective nanomolar inhibitors. Bioorg Med Chem Lett 2012;22:5801–6
- Monti SM, Maresca A, Viparelli F, et al. Dithiocarbamates are strong inhibitors of the beta-class fungal carbonic anhydrases from Cryptococcus neoformans, Candida albicans and Candida glabrata. Bioorg Med Chem Lett 2012;22:859–62
- Pacchiano F, Carta F, Vullo D, et al. Inhibition of β-carbonic anhydrases with ureido-substituted benzenesulfonamides. Bioorg Med Chem Lett 2011;21:102–5
- Carta F, Innocenti A, Hall RA, et al. Carbonic anhydrase inhibitors. Inhibition of the beta-class enzymes from the fungal pathogens Candida albicans and Cryptococcus neoformans with branched aliphatic/aromatic carboxylates and their derivatives. Bioorg Med Chem Lett 2011;21:2521–6
- Innocenti A, Hall RA, Scozzafava A, et al. Carbonic anhydrase activators: activation of the beta-carbonic anhydrases from the pathogenic fungi Candida albicans and Cryptococcus neoformans with amines and amino acids. Bioorg Med Chem 2010;18:1034–7
- Guzel O, Maresca A, Hall RA, et al. Carbonic anhydrase inhibitors. The beta-carbonic anhydrases from the fungal pathogens Cryptococcus neoformans and Candida albicans are strongly inhibited by substituted-phenyl-1H-indole-5-sulfonamides. Bioorg Med Chem Lett 2010;20:2508–11
- Schlicker C, Hall RA, Vullo D, et al. Structure and inhibition of the CO2-sensing carbonic anhydrase Can2 from the pathogenic fungus Cryptococcus neoformans. J Mol Biol 2009;385:1207–20
- Innocenti A, Winum JY, Hall RA, et al. Carbonic anhydrase inhibitors. Inhibition of the fungal beta-carbonic anhydrases from Candida albicans and Cryptococcus neoformans with boronic acids. Bioorg Med Chem Lett 2009;19:2642–5
- Innocenti A, Leewattanapasuk W, Muhlschlegel FA, et al. Carbonic anhydrase inhibitors. Inhibition of the beta-class enzyme from the pathogenic yeast Candida glabrata with anions. Bioorg Med Chem Lett 2009;19:4802–5
- Bozdag M, Carta F, Vullo D, et al. Dithiocarbamates with potent inhibitory activity against the Saccharomyces cerevisiae beta-carbonic anhydrase. J Enzyme Inhib Med Chem 2015. [Epub ahead of print]. doi: 10.3109/14756366.2015.1010529
- Supuran CT. Carbonic anhydrase inhibition/activation: trip of a scientist around the world in the search of novel chemotypes and drug targets. Curr Pharm Des 2010;16:3233–45
- Vullo D, Leewattanapasuk W, Muhlschlegel FA, et al. Carbonic anhydrase inhibitors: inhibition of the beta-class enzyme from the pathogenic yeast Candida glabrata with sulfonamides, sulfamates and sulfamides. Bioorg Med Chem Lett 2013;23:2647–52
- Carta F, Garaj V, Maresca A, et al. Sulfonamides incorporating 1,3,5-triazine moieties selectively and potently inhibit carbonic anhydrase transmembrane isoforms IX, XII and XIV over cytosolic isoforms I and II: solution and X-ray crystallographic studies. Bioorg Med Chem 2011;19:3105–119
- Di Silverio A, Zeccara C, Serra F, et al. Pityriasis versicolor in a newborn. Mycoses 1995;38:227–8
- Gadelha AR. [Pityriasis versicolor in a newborn infant]. Med Cutan Ibero Lat Am 1984;12:237–8
- Barac A, Pekmezovic M, Milobratovic D, et al. Presence, species distribution, and density of Malassezia yeast in patients with seborrhoeic dermatitis – a community-based case-control study and review of literature. Mycoses 2015;58:69–75
- Gaitanis G, Magiatis P, Hantschke M, et al. The Malassezia genus in skin and systemic diseases. Clin Microbiol Rev 2012;25:106–41
- Singh S, Supuran CT. In silico modeling of beta-carbonic anhydrase inhibitors from the fungus Malassezia globosa as antidandruff agents. J Enzyme Inhib Med Chem 2015. [Epub ahead of print]. doi: 10.3109/14756366.2015.1031127
- Hewitson KS, Vullo D, Scozzafava A, et al. Molecular cloning, characterization, and inhibition studies of a β-carbonic anhydrase from Malassezia globosa, a potential antidandruff target. J Med Chem 2012;55:3513–20
- Mount DW., ed. Sequence database searching for similar sequences. Chapter 6. In: Bioinformatics: sequence and genome analysis. 2nd ed. Cold Spring Harbor (NY): Cold Spring Harbor Laboratory Press; 2004. doi: 10.1101/pdb.top17
- Altschul SF, Gish W, Miller W, et al. Basic local alignment search tool. J Mol Biol 1990;215:403–10
- Edgar RC. MUSCLE: multiple sequence alignment with high accuracy and high throughput. Nucleic Acids Res 2004;32:1792–7
- Laemmli UK. Cleavage of structural proteins during the assembly of the head of bacteriophage T4. Nature 1970;227:680–5
- Khalifah RG. The carbon dioxide hydration activity of carbonic anhydrase. I. Stop-flow kinetic studies on the native human isoenzymes B and C. J Biol Chem 1971;246:2561–73
- Chai FC, Auret K, Christiansen K, et al. Malignant otitis externa caused by Malassezia sympodialis. Head Neck 2000;22:87–9
- Mayser P, Pickel M, Haze P, et al. Different utilization of neutral lipids by Malassezia furfur and Malassezia sympodialis. Med Mycol 1998;36:7–14
- Kimber MS, Pai EF. The active site architecture of Pisum sativum beta-carbonic anhydrase is a mirror image of that of alpha-carbonic anhydrases. EMBO J 2000;19:1407–18