Abstract
Peroxidases are ubiquitous enzymes that play an important role in living organisms. Current spectrophotometrically based peroxidase assay methods are based on the production of chromophoric substances at the end of the enzymatic reaction. The ambiguity regarding the formation and identity of the final chromophoric product and its possible reactions with other molecules have raised concerns about the accuracy of these methods. This can be of serious concern in inhibition studies. A novel spectrophotometric assay for peroxidase, based on direct measurement of a soluble aniline diazo substrate, is introduced. In addition to the routine assays, this method can be used in comprehensive kinetics studies. 4-[(4-Sulfophenyl)azo]aniline (λmax = 390 nm, ɛ = 32 880 M−1 cm−1 at pH 4.5 to 9) was introduced for routine assay of peroxidase. This compound is commercially available and is indexed as a food dye. Using this method, a detection limit of 0.05 nmol mL−1 was achieved for peroxidase.
Introduction
Peroxidases have found a special place in biotechnology, making them one of the most essential enzymatic groups. Using a hydroperoxide such as H2O2, peroxidases catalyze the oxidation of a wide variety of electron donor substrates; including phenols, aromatic amines, thioanisoles, and iodide. The reaction is a three-step cyclic process. Initially, the enzyme is oxidized by H2O2 then reduces back to the native form in two sequential steps, involving the formation of two enzyme intermediates, Compounds I and IICitation1.
(1)
(2)
(3)
Because of their unique physicochemical properties and their ability to oxidize different organic hydrogen donors (ZH), peroxidases have been employed in many industrial processesCitation2,Citation3. There is ongoing research with respect to discovering novel enzymes via scanning and high-throughput screening methodsCitation4,Citation5. Most of the current research in enzyme biotechnology are looking for enhancing the physicochemical features of the known enzymes through molecular approachesCitation6,Citation7, genetic engineering methodsCitation8, and techniques such as directed evolutionCitation9. The success of these approaches is heavily dependent on rapid and reliable assay methods.
Several assay methods exist for peroxidases but most of them are based on spectrophotometric approaches because of their low-cost and high precision. In these methods, the catalyzed reaction with the ZH is continuously monitored. Different features of some of the better known spectrophotometric assays are summarized in . As will be seen none of the substrates employed in these methods are themselves chromophores. Consequently, all these methods rely on the formation of chromophoric compounds, which result from the radicals produced at the end of the enzymatic reaction. The 2,2′-azino-bis(3-ethylbenzothiazoline-6-sulphonic acid) (ABTS) radical cation is chromophoric but not stable. The fate of the 3,3′,5,5′-tetramethylbenzidine (TMB) radical is not clearly resolved; however, there is evidence that a blue color imine is formed directly from the corresponding radicalCitation27,Citation28. The chromophoric products of the other methods are the result of intermolecular reactions between either the radicals themselves, or the radicals and a helper compound such as 4-aminoantipyrine (4AA).
The radical species produced can result in a complex profile of reaction products. This includes dimers, trimers, and higher oligomers that may act as reducing substrates in subsequent reactions. This complexity increases ambiguity regarding the identity of the final chromophoric productsCitation29,Citation30. Solubility and toxicity of the organic substrates are also matters of concern.
Table 1. Features of some selected peroxidase assay methods from the literature.
Regardless of these disadvantages, any of these assays can be used as routine assays for peroxidase but not for comprehensive kinetic purposes. In advanced kinetic studies, it is desirable to see how a small modification in the structure of the substrate or electron density on the binding terminal affects rate of the reaction. In this paper, a direct spectrophotometric method, based on the consumption of a water-soluble and safe chromophoric azo substrate is introduced for use in a routine assay of peroxidase. Using the general structure of the azo substrates, structure 3 in (), these substrates can also be used for advanced kinetic studies of peroxidases.
Materials and methods
Chemical, enzyme, and instruments
Horseradish peroxidase (HRP, EC 1.11.1.7; 100 U mg−1), and all the prerequisite chemicals for synthesis, were obtained from Merck (Darmstadt, Germany). The azo derivatives of aniline were synthesized as described below. The chemical structures were characterized based on various spectroscopic methods. NMR analysis was performed on a 500 MHZ Brucker spectrometer (Ultrashield) spectrometer. Proton and carbon chemical shifts are relative to tetramethylsilane. Spectrophotometric measurements were conducted on a Specord 210 spectrophotometer (AnalytikJena, Germany), equipped with a temperature controlling accessory. Infrared spectra were recorded using a Brucker (Tensor 27) FTIR spectrometer. Extinction coefficients of the dyes were determined at their λmax(s) in phosphate buffer solutions (PBS) at pH 7 and 20 °C. The HRP enzymatic reactions were carried out in PBS (0.01 M, pH 7) at 20 °C.
Synthesis of diazo dyes of aniline
A modified synthetic procedure was developed for the facile preparation of diazo derivatives of aniline (3) (). This method consisted of four simple and high yielding steps: making the diazonium salts with the general structure of 1, protecting the aniline amino group (2) and coupling reactions 1 with 2 followed by removing the protecting group.
Preparation of diazonium salts
Diazonium salts were synthesized by two different methods. 1a, 1b, 1c, 1e were synthesized through method 1 described in as previously described with no further modificationsCitation31. 1d was synthesized according to the procedure described by Jayakannan et al.Citation32
Synthesis of sodium anilinomethanesulfonate (2)
The procedure described by Zheng et al. was modified and used for the synthesis of sodium anilinomethanesulfonateCitation33. Sodium bisulfite (0.1 mol) was added to a solution of formaldehyde (0.1 mol) and distilled water (40 mL) with stirring. The mixture was heated to 65 °C for 1 h. To this mixture, aniline (0.08 mol) was added drop wise over 15 min and stirred for 2 h at 65 °C. The reaction mixture was cooled to about 0 °C which resulted in a bright gray precipitate that was filtered and recrystallized with water.
Synthesis of diazo derivatives of aniline (3)
2 (0.01 mol) was dissolved in double-distilled water (20 mL) containing sodium acetate (10 g) and cooled to 0 °C. The desired 1 was then added slowly to this solution at 0 °C. The resulting colorful mixture was agitated overnight at room temperature. The precipitate was filtered and washed with water. The crude product was dissolved in an aqueous solution of NaOH (1%, 50 mL) and the mixture refluxed until a change in color was evident (1–4 h). The solution was cooled to room temperature and the precipitate collected and recrystallized from the appropriate solvent.
3a: 4-[(4-methoxyphenyl)azo]aniline (CasNO 2592-28-1) was recrystallized from ethyl acetate as brownish–yellow crystals. Yield: 44%, mp: 140–142 °C. IR in KBr tablet contained: [NH2 (br. 3384 cm−1), CHAr (3042 cm−1), CH3 (2834 cm−1), -C = CAr (1602 and 1510 cm−1), -N = N- (1345 cm−1), -C-N- (1247 cm−1)]. 1HNMR in DMSO-d6; δ[-OCH3 (3.81, 3H, s), Ha(6.61, 2H, d, J = 8.75 Hz), Hb(7.57, 2H, d, J = 9 Hz), HC(7.65, 2H, d, J = 9.25 Hz,), Hd(6.81, 2H, d, J = 8.25 Hz), NH2(4.1, s, 2H)]. 3b: 4-[(4-methylphenyl)azo]aniline (CasNO 722-25-8) was recrystallized from a mixture of ethanol and water (2.5:1) as yellow crystals. Yield: 63%, mp: 142–145 °C. IR in KBr tablet contained: [NH2 (str. 3381, 3476 cm−1), CHAr (3206 cm−1), CH3 (2918 cm−1), -C = CAr (1620 and 1596 cm−1), -N = N- (1417 cm−1), -C-N- (1233 cm−1)]: 1HNMR in CDCl3; δ[-CH3 (2.40, 3H, s), Ha (6.73, 2H, d, J = 8.7 Hz,), Hb(7.73, 2H, d, J = 8.4 Hz), HC(7.81, 2H, d, J = 8.7 Hz), Hd(7.24, 2H, d, J = 8.7 Hz), -NH2(4.82, d, 2H)]. 3c: 4-aminoazobenzene (CasNO 60-09-3) was recrystallized from a mixture of ethanol and water (2.5:1) as yellow crystals. Yield: 67%, mp: 120–123 °C. IR in KBr tablet contained: [NH2 (str. 3382, 3477 cm−1), CHAr (3206 cm−1), -C = CAr (1595 cm−1), -N = N- (1415 cm−1), -C-N- (1234 cm−1)]; 1HNMR in DMSO-d6; δ[Ha(6.66, dd, 2H, J = 8.76 Hz, J = 2 Hz), Hb(7.65, 2H, dd, J = 8.75 Hz, J = 2 Hz), HC(7.73, 2H, dd, J = 7.4 Hz, J = 1.2 Hz), Hd(7.49, 2H, t, J = 8 Hz), HY(7.39, 1H, t, J = 7.4 Hz), -NH2(6.1, s)]. 3d: 4-[(4-sulfophenyl)azo]aniline (CasNO 104-23-4) was recrystallized from water as yellow crystals. Yield: 70%, mp: 290 °C. IR in KBr tablet contained: [NH2 (br. 3445 cm−1), CHAr (2923 cm−1), -C = CAr (1641 cm−1), -N = N- (1429 cm−1), -C-N- (1122 cm−1), -SO2 (1270 cm−1)]. 1HNMR in in DMSO-dCitation6: δ[Ha(7.03, 2H, d, J = 8.6 Hz), Hb, Hc,(7.75, 4H, s), Hd(7.80, 2H, d, J =8.6 Hz), -NH2(4.97, s)]. 3e: 4-[(4-sulfonamidophenyl)azo]aniline (CasNO 2435-64-1) was recrystallized from a mixture of ethanol and water (1:1) as yellow crystals. Yield: 68%, mp: 266–268 °C. IR in KBr tablet contained: [NH2 (br. 3345 cm−1), CHAr (3201 cm−1) -C = CAr (1601), -N = N- (1423 cm−1), -C-N- (1137 cm−1) -SO2 (1299 cm−1)]. 1HNMR in DMSO-dCitation6: δ[Ha(6.68, 2H, d, J = 9 Hz), Hb(7.70, 2H, d, J = 8.75 Hz), HC(7.85, 2H, d, J = 8.5 Hz), Hd(7.94, 2H, d, J = 8.75 Hz), -NH2(6.3, s), SO2NH2 (7.4, 2H, s)].
Preparation of enzyme and dye solutions
Freshly prepared enzyme solutions were used in this work. The enzyme stock solution was prepared by dissolving HRP (1.5 mg) in PBS (3 mL, 0.01 M, pH 7) and kept on ice. Most of the aniline diazo dyes are not readily soluble in water; therefore, the stock solutions of these compounds were prepared in the appropriate organic co-solvents as described in . The dye stock solutions were prepared by dissolving the desired dye (1 mg) in the appropriate co-solvent (4 mL). Desired dye concentrations were obtained by diluting the dye solutions in PBS (0.01 M, pH 7). To determine the impact of the co-solvent on the peroxidase activity, the enzymatic reaction was conducted in the media containing various amounts of co-solvent. Results showed the rate of the peroxidase reaction remained unchanged, even in medium containing up to 10% co-solvent. The amount of co-solvent did not exceed 7% of the reaction mixture in these experiments. The stability of the prepared dyes solutions was examined by monitoring the UV-visible spectrum and measuring the change of the absorption at the λmax of each dye for 60 min at 20 °C. In all cases, no change in the absorption spectra of the dye solutions, due to precipitation or auto-oxidation, was observed. The stock solution of hydrogen peroxide (0.1 M) was prepared by diluting H2O2 (0.5 mL, 30 wt%) to 50 mL in PBS (0.01 M, pH 7).
Table 2. Extinction coefficients, kinetic rates, and Michaelis–Menten parameters for the aniline diazo substrates. See experimental section for details.
Procedure for peroxidase assay and kinetic studies
The direct assay of HRP was conducted by following the oxidation of the diazo derivatives of aniline with the general structure 3 illustrated in in PBS (0.01 M, pH 7) at 20 °C. All enzymatic reactions were conducted in conventional quartz UV-visible cuvettes. Total volume of the reaction mixture was 3 mL containing at most 7% co-solvent if needed. Experiments were carried out in the presence of a constant concentration of HRP (100 μL) and H2O2 (20 μL) with seven different diazo substrate concentrations in a range of 7.5–100 μM. The desired volume of the dye solution was added into the quartz cuvette and diluted to 2880 μL with PBS (0.01 M, pH 7). To this mixture, H2O2 (20 μL) was added prior to the addition of the HRP solution (100 μL). The reference cell contained PBS (0.01 M, pH 7). The experiments were repeated five times under identical conditions.
The rate curves for each reaction were obtained by continuously monitoring the oxidation of the dye at the corresponding λmax at 20 °C for at least 4 min. The rate of the reaction at constant temperature was calculated under steady state conditions (). Michaelis–Menten constants for the substrates were calculated from the corresponding Lineweaver–Burk plots. All data presented are an average of n = 5 experiments.
Figure 2. (A) Velocity curves of the aniline diazo dyes during the reaction with HRP in the presence of a constant amount of enzyme, substrate (55 μM) in PBS (0.01 M, pH 7) at 20 °C. S.S = for steady state. (B) Overlaid spectra of 3d at pH 4.5, 7, and 9 at 20 °C. (C) Detection limit for HRP in the presence of 3d (20 mM) in PBS (0.01 M, pH 7) at 20 °C.
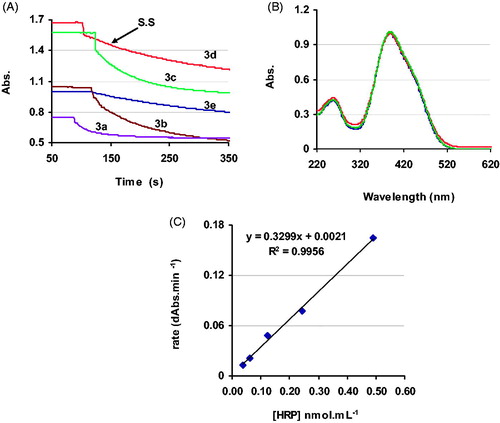
Results and discussion
Rationale for selecting diazo derivatives of aniline as peroxidase substrates
Experiments confirmed that hydrogen peroxide alone was not able to oxidize the diazo dyes in aqueous solution at pH(s) close to 7. Adding HRP into the mixture of H2O2 and the diazo compound initiated a rapid oxidation of the dye. Other oxidative enzymes, such as mushroom tyrosinase only oxidized diazo derivatives of phenol and catecholCitation34. Neurospora crassa laccaseCitation35 was effective on diazo derivatives of catechol and guaiacol. Using conditions previously introducedCitation34,Citation35, N. crassa laccase and mushroom tyrosinase had little effect on the diazo derivatives of aniline (data not shown here). In contrast, it was only HRP which could oxidize diazo derivatives of aniline with the general structure of 3 () in the presence of H2O2. Noteworthy is that phenolases usually interfere with peroxidase assays when a natural extract is being assayed. Therefore, the accuracy of peroxidase assays which are based on using substrates such as ABTS, guaiacol, phenol, and pyrocatechol are affected as these compounds participate in enzymatic reactions with tyrosinase and laccase producing very active intermediatesCitation34,Citation35.
HRP reactions with diazo derivatives of phenol, guaiacol, and catechol are too rapid to be measured easily using simple spectrophotometers. In contrast, HRP reactions with compounds 3 are kinetically slower so progress of the reaction can be easily monitored spectrophotometerically (). Reasons for the difference in the rates of the HRP reactions with diazo derivatives of aniline and phenols have been extensively discussedCitation36. It is understood that the rate of the oxidation of compounds 3 by HRP is influenced by the nature of the substituent Y (). Derivatives of 3 carrying electron donating substituents (EdS) have faster reactions with HRP and produce steeper velocity curves, while those carrying electron withdrawing substituent (EwS) show longer steady state as a result of slower reactions ().
Although some derivatives of 3 are commercially available, the synthetic route to these compounds is not complicated. Therefore, in more advanced and comprehensive kinetic studies where a specific derivative of 3 is required, it is possible to synthesize the desired substrate by following the modified procedure described in the experimental section. Generally, the synthesis of azo dyes involves a process known as diazo coupling. In this process, a diazonium salt, such as 1, is coupled with the aromatic ring of a phenol or anilineCitation31. In the case of aniline, the diazonium salt can react with both the aromatic ring and the amino groupCitation37. To direct the coupling reaction toward the aromatic ring, the amino group of aniline is protected by a sulfomethyl group, compound 2. Finally, the coupling reaction between 1 and 2 results in formation of high yields of the diazo dyes of aniline 3.
Development of the peroxidase assay
provide the overlaid spectra collected during the oxidation of compounds 3 by HRP. The UV-visible spectrum of each dye shows only one peak in the broad region between 300 and 550 nm with a λmax around 380–400 nm. The optical density of each dye decreases at its λmax while oxidized by HRP. This allows the progress of the reaction to be monitored directly by following the depletion of the substrate. shows that the velocity curve of the enzymatic reaction reaches a plateau. This is because of the advent of another absorbing species (product molecules) in the reaction mixture. Formation of the isosbestic point in the region of 350–366 nm confirms the interference of the product(s) absorption with that of the substrate (). But this phenomenon does not affect the accuracy of this method. Upon enzymatic oxidation of an aniline diazo dye, the double bond of the diazo functional group (-N = N-) is cleaved and the amino group is oxidized to nitroCitation38. The degradation products, nitro derivatives of benzene and phenol, have λmax(s) between 350 and 360 nmCitation39. This means that there is about 20–30 nm difference between the λmax(s) of the diazo substrate and its corresponding degradation products. This allows direct monitoring of the reaction’s progress and more precise estimation of substrate consumption, especially during the early stages of the reaction.
Figure 3. Overlaid spectra recorded during the HRP reaction with (A) 3a, (B) 3b, (C) 3c, (D) 3d, (E) 3e in PBS (0.01 M, pH 7) at 20 °C.
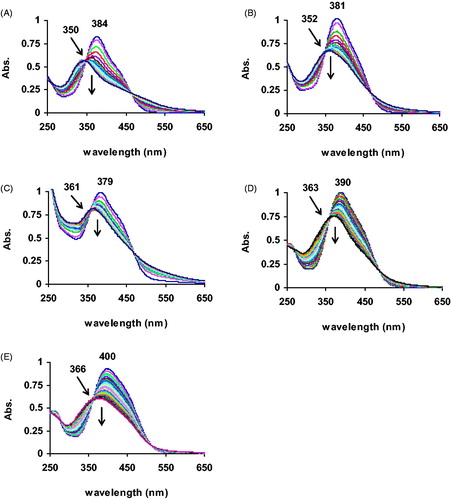
Sensitivity of a spectrophotometric method depends on the sharpness of the adsorption peak and the extinction coefficient (ɛ) of the chromophore at that peakCitation34. provides the extinction coefficients of compounds 3 in PBS at pH 7 and 20 °C. Compound 3d has the highest extinction coefficient value. During the HRP-catalyzed reaction, one isosbestic point forms at 27 nm from the λmax of 3d at 363 nm (). The velocity curve of 3d () shows that steady state was not affected by the advent of the products in the reaction mixture. As a result, 3d exhibited a long steady state of more than 100 s under the experimental conditions. A longer steady state infers a slower reaction rate that is attributed to the presence of the EwS (-SO3H) in the chemical structure. This sulfo substituent also increases the solubility of 3d in water () obviating the need for a co-solvent. The spectrophotometric behavior of 3d would indicate that it is insensitive to pH changes over a broad range of 4.5 to 9 (). This allows 3d to be used for the assay of acidic, neutral, and basic peroxidases with the same extinction coefficient value. With 3d as substrate, a detection limit of 50 pmol·mL−1 was achieved for HRP ().
Some of the peroxidase methods featured in are criticized because of carcinogenicity or mutagenicity of the organic substrates employed. 3d is soluble in water and is listed under the name of food yellow 6 in the substance services of US Environmental Protection Agency and is approved as a coloring agent for food, drugs, and cosmeticsCitation40. Considering these features and the reproducible linear spectrophotometric responses, 3d is proposed as the preferred substrate for reliable and routine assay of peroxidases.
Comparison of the methods
In addition to the fluorometric assay-kit using Amplex® RedCitation41, there are two spectrophotometric methods which are commonly used for assaying peroxidases: TMB and the combination of phenol and 4AA. The use of phenol in the latter method makes it unpleasant. Phenol is toxic and has low solubility in waterCitation15. In addition, phenol is oxidized by both tyrosinase and laccaseCitation34,Citation35 and its reaction with 4AA is not specific which means that 4AA can produce the quinoid product by reacting with other phenolic substances that can be present in natural extractsCitation16,Citation42.
The method based on TMB has several advantages as compared to phenol and 4AA. The TMB method relies on a single organic substrateCitation24 so there is no need to use a helper molecule such as 4AA to produce a chromophoric product. The extinction coefficient of the enzymatic oxidation product of TMB is greater than that of the quinoid product of phenol oxidation in the presence of 4AACitation24. TMB was identified as a suitable substrate as a result of several studies which were carried out on the enzymatic oxidation of aniline derivatives. Most of the anilines that have been studied, such as benzidine and its derivatives, are mutagenic and carcinogenicCitation11,Citation43. TMB has been found to be non-mutagenicCitation27 although it may be carcinogenic. Nevertheless, there are some limitations to the TMB method. TMB has a very low solubility in water so some modifications are required to increase its solubility. For example, TMB can form a complex with dextrin that is water soluble and responds to peroxidaseCitation44.
The enzymatic action of HRP on TMB produces an unstable chromophoric product (λmax = 653 nm, ɛ = 39 000 M−1 cm−1)Citation24,Citation44 as well as for dextrin complex. The reaction must be acidified to produce a stable product. This makes the method a two-step method but results in formation of a stable chromophoric product (λmax = 450 nm, ɛ = 59 000 M−1 cm−1)Citation44,Citation45. Recent studies seriously question accuracy of the product based assay methods for peroxidase. As mentioned, the first product of the enzymatic oxidation of an organic substrate (ZH) such as TMB is its radical (Z°). It has been shown that molecules with radical scavenging ability such as phenolic acids affect the results of the assay by reducing Z° species in the assay mixtureCitation30,Citation46. The method described in this paper is based on the oxidation of a stable substrate. The substrate identified here that is suitable for routine peroxidase assays, 3d, is soluble in water, chromophoric with a large extinction coefficient. Fresh solutions can be easily prepared and the solution is stable even in the presence of hydrogen peroxide. The oxidation of 3d with peroxidase can be monitored directly and precisely by following its optical density at 390 nm over a wide pH range. Given that, this compound is commercially available under the food dyes categoryCitation40 implies higher safety than others in .
Although all the methods described in can be used for routine peroxidase assays, by using the facile synthetic method introduced here it is possible to synthesize a suite of substrates carrying different EdS and EwS at different positions on the aromatic rings of structure 3. This approach can be very informative from a kinetics perspective.
Kinetic parameters of aniline diazo substrates
An important advantage of this direct method is that it allows the accurate study of peroxidase kinetics in a comprehensive manner. The physicochemical properties of novel peroxidases are very importantCitation2,Citation47. Accurate kinetic studies shed light on the reaction mechanisms through which peroxidases respond to different substrates. These studies are usually carried out in a comparative style using a well-studied peroxidase such as HRPCitation14. Such studies usually result in important kinetic constants that can be meaningful from a mechanistic perspective. The kinetic parameters of each diazo substrate () were calculated from the corresponding Lineweaver–Burk plots of the kinetic studies shown in .
Figure 4. Lineweaver–Burk plot of the kinetic data for the HRP reactions with various concentrations (10–100 mM) of 3d (R2 = 95.3, SEMax = ±0.006) in PBS (0.01 M, pH 7) at 20 °C. SEMax reports the maximum standard deviation observed for the corresponding data.
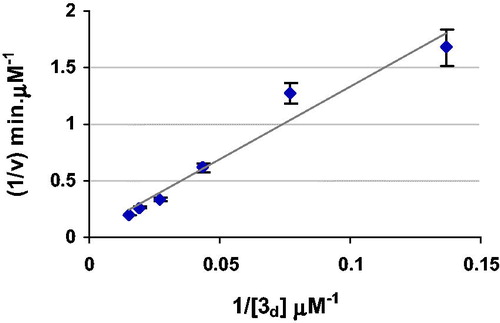
The instantaneous rate values (dC/min) corroborate that the dyes carrying EdS exhibit a faster reaction rate with HRP. The peroxidase reaction starts with the displacement of a water molecule by H2O2 in the coordination sphere of the heme iron in the active site (). Due to formation of a bond between Fe3+ and one of the peroxide oxygens, the O–O bond is heterolytically cleaved. The hydroxide ion forms a water molecule and leaves the active site. The bound oxygen participates in a fast intramolecular electron transfer and acquires two electrons, one withdrawn from the iron and the other from the porphyrin ringCitation48. The resulting intermediate, Compound I, is a strong electrophile. An EdS facilitates the instant electron transfer from the substrate to the HRP. The specificity constants (kcat/Km) also support faster reactions of substrates carrying EdS. For instance, in a mixture of 3a and 3d, the molecules of 3a react more than three times faster than the 3d molecules with HRP. This feature does not guarantee a lower Km value for the diazo substrate carrying an EdS.
Figure 5. Proposed mechanisms for the catalytic activity of heme peroxidase in the presence of hydrogen peroxideCitation49.
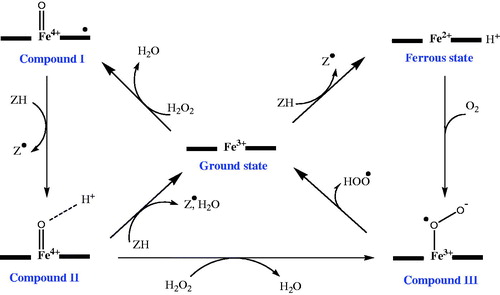
Despite the fact that the substrates with EdS have lower Km values, compared to those carrying EwS, the data in indicate that 3c, which has neither EdS nor EwS, has the lowest Km value. A correlation between the half potential of the reducing substrate (ZH) and the rate of the reaction with the activated HRP has been observedCitation43,Citation49. However, no clear correlation between the binding constant of the reducing substrate with compound I, and the rate of the reaction, has been foundCitation50. Considering the results reported here, two assumptions can be proposed to explain the low Km value for 3C.
First, studies show that there is possibility for formation of an extra intermediate (Compound III illustrated in ) at higher concentrations of hydrogen peroxide or upon direct reaction of ZH with the enzymeCitation51. Compound III is capable of initiating some radical reactionsCitation52. This phenomenon increases the complexity of HRP reactions and might have affected the kinetic constants of the substrates examined. But, all enzymatic reactions were carried out in the presence of a constant amount of H2O2 (0.7 mM) throughout this study. The high reproducibility of the results and the linear responses over the selected range of substrate concentrations () do not support this assumption.
Second, structural examinations show that the binding of the reducing substrate to the activated HRP involves specific interactions with the residues in the active site pocket of the enzymeCitation50. This means that the structural details of ZH have a strong impact on the observed binding constant. For instance, when the reducing substrate is ferulic acid, after binding the aromatic residue of ferulic acid is oriented toward the entrance of the active site in such a way that the oxygen of the methoxy substituent is involved in hydrogen binding with some residues in the active site pocketCitation53. Compound 3 has a planar structure similar to that of ferulic acid. The methoxy group of ferulic acid is at the ortho position of the phenolic ring, while the Y substituent is at the para position of the second aromatic ring of 3. This may cause unfavorable interaction(s) between the Y substituent of the diazo substrates carrying either EdS or EwS, regardless of its nature, and the HRP active site pocket.
Conclusion
A new method was developed for assaying peroxidase activity using diazo derivatives of aniline with the general structure of 3 as the substrate. Using this method, peroxidase activity can be measured directly through oxidation of the chromophoric substrate. Compounds 3 can be prepared in high yield by a simple procedure described in this paper. These compounds have rather sharp absorbance peaks in the visible region and also have large extinction coefficients. Reaction of HRP with 3d, which is a water-soluble food dye with an extinction coefficient of 32 880 M−1·cm−1 at 390 nm at a pH of 4.5 to 9, was introduced for routine peroxidase assays. However, any of the compounds 3 can be used in comprehensive peroxidase kinetic studies. Kinetics parameters introduced in this work can be used for comparative kinetic studies of novel peroxidases.
Acknowledgements
This work was carried out in the Chemistry and Biochemistry laboratories of the National Institute of Genetic Engineering and Biotechnology. The collaboration with Dr Richard W. Smith and Mrs. Janet Venne in the Mass Spectroscopy and WATSPEC Nuclear Magnetic Resonance Spectroscopy units at the University of Waterloo is greatly appreciated.
Declaration of interest
The authors report no declarations of interest.
References
- Dunford HB. Peroxidases and catalases. 2nd ed. Hoboken, NJ: Wiley; 2010
- Hamid M, Rehman KU. Potential applications of peroxidases. Food Chem 2009;115:1177–86
- Hu XJ, Wang XB, Ling-Yi Kong LY. αGlucosidase inhibitors via green pathway: biotransformation for bicoumarins catalyzed by momordica charantia peroxidase. J Agric Food Chem 2013;61:1501–8
- Rodrigues-Santos WJ, Lima PR, Teixeira Tarley CR, Kubota LT. A catalytically active molecularly imprinted polymer that mimics peroxidase based on hemin: application to the determination of p-aminophenol. Anal Bioanal Chem 2007;389:1919–29
- Reymond JL, Fluxa VS, Maillard N. Enzyme assays. Chem Commun 2009;7:34–46
- Taylor SV, Kast P, Hilvert D. Investigating and engineering enzymes by genetic selection. Angew Chem Int Ed Engl 2001;40:3310–35
- Fortea MI, Pellicer JA, Serrano-Martínez A, et al. Red cabbage (Brassica oleracea) as a new source of high-thermostable peroxidase. J Agric Food Chem 2012;60:10641–8
- Bloom JD, Meyer MM, Meinhold P, et al. Evolving strategies for enzyme engineering. Curr Opin Struct Biol 2005;15:447–52
- Leemhuis H, Kelly RM, Dijkhuizen L. Directed evolution of enzymes: library screening strategies. IUBMB Life 2009;61:222–8
- Childs RE, Bardsley WG. The steady-state kinetics of peroxidase with 2,2′-azino-di-(3-ethyl-benzthiazoline-6-sulphonic acid) as chromogen. Biochem J 1975;145:93–103
- Bomhard EM, Herbold BA. Genotoxic activities of aniline and its metabolites and their relationship to the carcinogenicity of aniline in the spleen of rats. Crit Rev Toxicol 2005;35:783–835
- Shivakumar A, Rangappa D, Krishna H, Nagaraja P. Development and kinetic validation of an assay for the quantitative determination of peroxidase: application in the detection of activity in crude plant tissues. Enzyme Microb Technol 2010;47:243–8
- Martíne Enriquez ME, Del Villar A, Chauvet D, et al. Acute toxicity of guaiacol administered subcutaneously in the mouse. Proc West Pharmacol Soc 2009;52:92–3
- Farhadi S, Haghbeen K, Marefatjo MJ, et al. Anionic peroxidase production by Arnebia euchroma callus. Biotechnol Appl Biochem 2011;58:456–63
- Michaowicz J, Duda W. Phenols – sources and toxicity. Pol J Environ Stud 2007;16:347–62
- Metelitza DI, Litvinchuk AV, Savenkova MI. Peroxidasecatalyzed co-oxidation of halogen-substituted phenols and 4-aminoantipyrine. J Mol Catalysis 1991;67:401–11
- Martelli A, Robbiano L, Carrozzino R, et al. DNA damage induced by 3,3′-dimethoxybenzidine in liver and urinary bladder cells of rats and humans. Toxicol Sci 2000;53:71–6
- Fornera S, Yazawa K, Walde P. Spectrophotometric quantification of lactose in solution with a peroxidase-based enzymatic cascade reaction system. Anal Bioanal Chem 2011;401:2307–10
- Chichirau A, Flueraru M, Chepelev LL, et al. Mechanism of cytotoxicity of catechols and a naphthalenediol in PC12-AC cells: the connection between extracellular autoxidation and molecular electronic structure. Free Radic Biol Med 2005;38:344–55
- Molaei Rad A, Ghourchian H, Mousavi-Movahedi AA, et al. Spectrophotometric assay for horseradish peroxidase activity based on pyrocatechol-aniline coupling hydrogen donor. Anal Biochem 2007;362:38–43
- Takemura Y, Wang DH, Sauriasari R, et al. Evaluation of pyrogallol-induced cytotoxicity in catalase-mutant Escherichia coli and mutagenicity in Salmonella typhimurium. Bull Environ Contam Toxicol 2010;84:347–50
- Pruitt MK, Kamau DN, Miller K, et al. Quantitative, standardized assays for determining the concentrations of bovine lactoperoxidase, human salivary peroxidase, and human myeloperoxidase. Anal Biochem 1990;191:278–86
- Ku WW, Aubrecht J, Mauthe RJ, et al. Genetic toxicity assessment: employing the best science for human safety evaluation part VII: why not start with a single test: a transformational alternative to genotoxicity hazard and risk assessment. Toxicol Sci 2007;99:20–5
- Josephy PD, Eling T, Mason RP. The horseradish peroxidase-catalyzed oxidation of 3,5,3′,5′-tetramethylbenzidine. Free radical and charge-transfer complex intermediates. J Biol Chem 1982;257:3669–75
- Yoshikawa K, Nohmi T, Harada R, et al. Differential mutagenicities of triamino benzenes against Salmonella typhimurium TA98 in the presence of S9 fractions from polychlorinated biphenyls-, phenobarbital- or 3-methylcholanthrene-pretreated rats, hamsters and mice. J Toxicol Sci 1979;4:317–26
- Fornera S, Walde P. Spectrophotometric quantification of horseradish peroxidase with o-phenylenediamine. Anal Biochem 2010;407:293–5
- Nickel KS, Cunningham BA. Improved peroxidase assay method using leuco 2,3′,6-trichloroindophenol and application to comparative measurements of peroxidatic catalysis. Anal Biochem 1969;27:292–9
- Vojinovic V, Azevedo AM, Martins VCB, et al. Assay of H2O2 by HRP catalysed co-oxidation of phenol-4-sulphonic acid and 4-aminoantipyrine: characterization and optimization. J Mol Catal B: Enzym 2004;28:129–35
- Azevedo AM, Martins VC, Prazeres DMF, et al. Horseradish peroxidase: a valuable tool in biotechnology. Biotechnol Annu Rev 2003;9:199–247
- Shaukat S, Waqar MA. Green tea phenols interference in the glucose oxidase/peroxidase test. J Food Biochem 2011;35:1170–85
- Haghbeen K, Tan EW. Facile synthesis of catechol azo dyes. Org Chem 1998;63:4503–5
- Jayakannan M, Anilkumar P, Sanju A. Synthesis and characterization of new azobenzenesulfonic acids doped conducting polyaniline. Eur Polym J 2006;42:2623–31
- Zheng Z, Wang L, Su Z, et al. Photoinduced chirality in achiral liquid crystalline polymethacrylates containing bisazobenzene and azobenzene chromophores. J Photochem Photobiol A: Chem 2007;185:338–44
- Haghbeen K, Tan EW. Direct spectrophotometric assay of monooxygenase and oxidase activities of mushroom tyrosinase in the presence of synthetic and natural substrates. Anal Biochem 2003;312:23–32
- Moshtaghioun SM, Haghbeen K, Lotfi A, et al. Direct spectrophotometric assay of laccase using diazo derivatives of guaiacol. Anal Chem 2011;83:4200–5
- Van Haandel MJ, Claassens MM, Van der Hout N, et al. Differential substrate behaviour of phenol and aniline derivatives during conversion by horseradish peroxidase. Biochim Biophys Acta 1999;1435:22–9
- Merino E. Synthesis of azobenzenes: the coloured pieces of molecular materials. Chem Soc Rev 2011;40:3835–53
- Barros VP. Iron porphyrins as biomimetical models for disperse azo dye oxidation. J Braz Chem Soc 2013;24:830–6
- Ehlerova J, Trevani L, Sedlbauer J, Tremaine PR. Spectrophotometric determination of the ionization constants of aqueous nitrophenols at temperatures up to 225 °C. J Solution Chem 2008;37:857–74
- Baltac T, Vlad G, Sebe I. The synthesis of some food azo dyes. Rev Chim (Bucharest) 2012;63:18–21
- Jiang R, Bommarius AS. Hydrogen peroxide-producing NADH oxidase (nox-1) from Lactococcus lactis. Tetrahedron: Asym 2004;15:2939–44
- Blake DA, McLean NV. A colorimetric assay for the measurement of D-glucose consumption by cultured cells. Anal Biochem 1989;177:156–60
- Job D, Dunford HB. Substituent effect on the oxidation of phenols and aromatic amines by horseradish peroxidase Compound I. Eur J Biochem 1976;66:607–14
- Cattaneo MV, Luong JH. A stable water-soluble tetramethylbenzidine-2-hydroxypropyl-beta-cyclodextrin inclusion complex and its applications in enzyme assays. Anal Biochem 1994;223:313–20
- Jang G, Roper DK. Balancing redox activity allowing spectrophotometric detection of Au(I) using tetramethylbenzidine dihydrochloride. Anal Chem 2011;83:1836–42
- Wong AIC, Huang D. Assessment of the degree of interference of polyphenolic compounds on glucose oxidation/peroxidase assay. J Agric Food Chem 2014;62:4571–6
- Banerjee G, Scott-Craig JS, Walton JD. Improving enzymes for biomass conversion: a basic research perspective. Bioenerg Res 2010;3:82–92
- Hollmann F, Arends IWCE. Enzyme initiated radical polymerizations. Polymers 2012;4:759–93
- Candeias LP, Folkes LK, Porssa M, et al. Rates of reaction of indoleacetic acids with horseradish peroxidase compound I and their dependence on the redox potentials. Biochemistry 1996;35:102–8
- Gajhede M. Plant peroxidases: substrate complexes with mechanistic implications. Biochem Soc Trans 2001;29:91–8
- Berglund GI, Carlsson GH, Smith AT, et al. The catalytic pathway of horseradish peroxidase at high resolution. Nature 2002;417:463–8
- Valderrama B, Ayala M, Vazquez-Duhalt R. Suicide inactivation of peroxidases and the challenge of engineering more robust enzymes. Chem Biol 2002;9:555–65
- Veitch NC. Horseradish peroxidase: a modern view of a classic enzyme. Phytochemistry 2004;65:249–59