Abstract
N-Protected amino acids (Gly, Ala and Phe) were reacted with amino substituted coumarin and quinolinone derivatives, leading to the corresponding N-protected amino acid–coumarin/quinolinone conjugates. The carbonic anhydrase (CA, EC 4.2.1.1) inhibitory activity of the new compounds was assessed against various human (h) isoforms, such as hCA I, hCA II, hCA IV and hCA XII. The quinolinone conjugates were inactive as enzyme inhibitors, whereas the coumarins were ineffective hCA I/II inhibitors (KIs > 50 μM) but were submicromolar hCA IV and XII inhibitors, with inhibition constants ranging between 92 nM and 1.19 μM for hCA IV, and between 0.11 and 0.79 μM for hCA XII. These coumarin derivatives, as many others reported earlier, thus show an interesting selective inhibitory profile for the membrane-bound over the cytosolic CA isoforms.
Introduction
Coumarin and quinolinone derivatives are classes of heterocyclic scaffolds exhibiting several biological properties such as carbonic anhydrase (CA, EC 4.2.1.1) inhibitionCitation1, anticoagulantCitation2 and antioxidantCitation3 properties among others. Discovered initially in a library of natural products, the first coumarin CA inhibitor (CAI) was isolated from the Australian plant Leionema elipticumCitation1. A large number of synthetic and natural product coumarins were subsequently investigated as inhibitors of these enzymesCitation4–10, due to the fact that they showed a high degree of selectivity for inhibiting a number of isoforms of the 15 presently described in humans, hCA I–hCA XIV (there are two V-type isoforms, hCA VA and hCA VB)Citation11–19. Furthermore, these enzymes are widespread in organisms all over the phylogenetic tree, and their inhibition has pharmacologic applicationsCitation11–20.
With the hope to obtain new biologically active coumarin and quinolinone derivatives, the current work focused on the synthesis of amino acid–coumarin/quinolinone conjugates by using the N-(protected α-aminoacyl)benzotriazole methodologyCitation21 and the exploration of the enzyme inhibitory activities of such compounds against physiologically important isoforms, such as the cytosolic human (h) hCAI and II, as well as the membrane-associated/transmembrane hCA IV and XII.
Material and methods
Chemistry
Anhydrous solvents and all reagents were purchased from Sigma-Aldrich (Milan, Italy), Acros (Milan, Italy) and Merck (Florence, Italy). All reactions involving air- or moisture-sensitive compounds were performed under a nitrogen atmosphere using dried glassware and syringes techniques to transfer solutions. Nuclear magnetic resonance (1H NMR, 13C NMR) spectra were recorded using a Bruker Advance III 400 MHz spectrometer in DMSO-d6. Chemical shifts are reported in parts per million (ppm) and the coupling constants (J) are expressed in Hertz (Hz). Splitting patterns are designated as follows: s, singlet; d, doublet; t, triplet; m, multiplet; brs, broad singlet; dd, double of doubles. The assignment of exchangeable protons (OH and NH) was confirmed by the addition of D2O. Positive-ion electrospray ionization mass spectra were recorded on a double-focusing Finnigan MAT 95 instrument with BE geometry. Analytical thin-layer chromatography (TLC) was carried out on Merck silica gel F-254 plates. All microwave-assisted reactions were carried out in a microwave oven system manufactured by GEM (CEM Matthews, NC) under a nitrogen atmosphere. The reaction mixtures were transferred into a 10-mL glass pressure microwave tube equipped with a magnetic stir bar. The tube was closed with a silicon septum and the reaction mixture was subjected to microwave irradiation. Melting points (mp) were measured in open capillary tubes and are uncorrected, using a Gallenkamp MPD350.BM3.5 apparatus. N-protected amino acids; benzyl (2-1H-benzo[d][1,2,3]triazol-1-yl)-2-oxoethyl)carbamate (I), tert-butyl (2-1H-benzo[d][1,2,3]triazol-1-yl)-2-oxoethyl)carbamate (II), (S)-benzyl (1-(1H-benzo[d][1,2,3]triazol-1-yl)-1-oxopropan-2-yl)carbamate (III), (S)-tert-butyl (1-(1H-benzo[d][1,2,3]triazol-1-yl)-1-oxo-3-phenylpropan-2-yl)carbamate (IV) used as starting materials were prepared according to literature procedureCitation22–24. Compounds 5Citation25 and 6Citation26 were found in the literature but their synthesis methods need harsh reaction conditions and cumbersome work-up procedures. Thus, these compounds were synthesized by a new, one-step, easy synthetic method using N-(protected α-aminoacyl)benzotriazoles in this study.
General procedure for the synthesis of amino acid–coumarin conjugates, 1–6
A mixture of equivalent amounts of the appropriate N-protected aminoacylbenzotriazole and 6-amino-2H-chromen-2-one or 7-amino-4-methyl-2H-chromen-2-one was subjected to microwave irradiation (100 W, 70 °C) in anhydrous THF (5 mL) for 30 min. After completion of the reaction, all volatiles were removed by rotavapor and the obtained crude product was crystallized from methanol.
Benzyl (2-oxo-2-((2-oxo-2H-chromen-6-yl)amino)ethyl)carbamate, 1
Beige solid (95%); silica gel TLC Rf = 0.20 (MeOH/CH2Cl2 5% v/v); mp 160–161 °C; 1H NMR (DMSO-d6, 400 MHz) δ 10.23 (s, 1H, NH), 8.10 (d, 1H, CH = CH–CO), J = 12.0 Hz), 8.06 (s, 1H, Ar–H), 7.71 (m, 1H, Ar-H), 7.62 (t,1H, NH, J = 8.0 Hz), 7.40-7.35 (m, 6H, Ar–H), 6.52 (d, 1H, =CH–CO, J = 12.0 Hz), 5.10 (s, 2H, CH2Ph), 3.88 (d, 2H, CH2NH, J = 8,0 Hz). 13C NMR (DMSO-d6, 400 MHz) δ 169.1 (COCH2NH), 160.9 (O-C=O), 157.5 (COOCH2Ph), 150.3, 145.2, 137.9, 136.2, 129.3, 128.7, 128.6, 124.2, 119.6, 118.7, 117.6, 117.4 (Ar-C + C = C), 66.4 (CH2Ph), 45.0 (CH2NH). HRMS m/z for C19H16N2O5 [M + H]+ calcd. 353.1, found 353.1; [M + Na]+ calcd. 375.1, found 375.0.
tert-butyl (2-oxo-2-((2-oxo-2H-chromen-6-yl)amino)ethyl)carbamate, 2
Yellow solid (96%); silica gel TLC Rf = 0.77 (MeOH/CH2Cl2 5% v/v); mp 201–202 °C; 1H NMR (DMSO-d6, 400 MHz) δ 10.17 (s, 1H, NH), 8.12 (d, 1H, CH=CH–CO), J = 12.0 Hz), 8.07 (s, 1H, Ar–H), 7.71 (m, 1H, Ar–H), 7.11 (t,1H, NH, J = 4.0 Hz), 6.52 (d, 1H, =CH–CO, J = 12.0 Hz), 3.78 (d, 2H, CH2NH, J = 4,0 Hz). 1.44 (s, (CH3)3C, 9H). 13C NMR (DMSO-d6, 400 MHz) δ 169.3 (COCH2NH), 160.9 (O–C=O), 156.8 (COOCH2Ph), 145.3, 137.9, 136.3, 124.2, 119.6, 118.6, 117.6, 117.4 (Ar–C + C = C), 79.0 (C(CH3)3), 44.7 (CH2NH), 29.1 ((CH3)3C. HRMS m/z for C19H18N2O5 [M + H]+ calcd. 319.1, found 319.1; [M + Na]+ calcd. 341.1, found 341.1.
(S)-benzyl (1-oxo-1-((2-oxo-2H-chromen-6-yl)amino)propan-2-yl)carbamate, 3
Beige solid (92%); silica gel TLC Rf = 0.36 (MeOH/CH2Cl2 5% v/v); mp 87–88 °C; 1H NMR (DMSO-d6, 400 MHz) δ 10.24 (s, 1H, NH), 8.11 (d, 1H, CH=CH–CO), J = 8.0 Hz), 8.07 (s, 1H, Ar–H), 7.73 (m, 1H, Ar–H), 7.67 (t,1H, NH, J = 8.0 Hz), 7.42–7.40 (m, 6H, Ar–H), 6.52 (d, 1H, =CH–CO, J = 8.0 Hz), 5.75 (s, 2H, CH2Ph), 4.24 (t, 2H, CH2NH, J = 8,0 Hz), 1.35 (d, 3H, CH3, J = 8.0 Hz. 13C NMR (DMSO-d6, 400 MHz) δ 172.6 (COCH2NH), 160.9 (O–C=O), 157.2 (COOCH2Ph), 150.3, 145.3, 137.9, 136.3, 129.3, 128.7, 128.6, 124.4, 119.6, 118.9, 117.6, 117.4 (Ar–C + C = C), 66.4 (CH2Ph), 51.7 (CH2NH), 18.8 (CH3). HRMS m/z for C20H18N2O5 [M + H]+ calcd. 367.1, found 367.0; [M + Na]+ calcd. 389.1, found 389.1.
(S)-benzyl (1-oxo-1-((2-oxo-2H-chromen-6-yl)amino)-3-phenylpropan-2-yl)carbamate, 4
Brown solid (94%); silica gel TLC Rf = 0.33 (MeOH/CH2Cl2 5% v/v); mp 137–138 °C; 1H NMR (DMSO-d6, 400 MHz) δ 10.38 (s, 1H, NH), 8.11 (m, 2H, CH=CH–CO + Ar–H), 7.96 (t,1H, NH, J = 4.0 Hz), 7.95–7.94 (m, 2H, Ar–H), 7.80–7.71 (m, 10H, Ar–H), 6.52 (d, 1H, =CH–CO, J = 12.0 Hz), 5.02 (s, 2H, CH2Ph), 4.47 (m, 1H, CHNH), 3.11 (m, 1H, CH2Ph), 2.93 (m, 1H, CH2Ph). 13C NMR (DMSO-d6, 400 MHz) δ 171.6 (COCH2NH), 160.9 (O–C=O), 156.9 (COOCH2Ph), 150.4, 145.2, 138.6, 137.8, 136.1, 130.1, 129.2, 129.0, 128.6, 128.5, 127.3, 124.5, 119.6, 119.0, 117.6, 117.4 (Ar–C + C = C), 66.3 (OCH2Ph), 57.9 (CHNH), 38.3 (CH2Ph). HRMS m/z for C26H22N2O5 [M + H]+ calcd. 443.2, found 443.1.0; [M + Na]+ calcd. 465.2, found 465.1.
Benzyl (2-((4-methyl-2-oxo-2H-chromen-7-yl)amino)-2-oxoethyl)carbamate, 5
Yellow solid (87%); silica gel TLC Rf = 0.61 (MeOH/CH2Cl2 5% v/v); mp 234–235 °C; 1H NMR (DMSO-d6, 400 MHz) δ 10.46 (s, 1H, NH), 7.79–7.76 (m, 2H, Ar–H), 7.65 (t,1H, NH, J = 4.0 Hz), 7.52 (m, 1H, Ar–H), 7.44–7.36 (m, 5H, Ar–H), 6.30 (s, 1H, =CH–CO), 5.10 (s, 2H, CH2Ph), 3.90 (d, 2H, CH2NH, J = 4,0 Hz), 2.44 (s, 3H, CH3). 13C NMR (DMSO-d6, 400 MHz) δ 169.7 (COCH2NH), 160.9 (O-C=O), 157.6 (COOCH2Ph), 154.6, 154.0, 143.1, 137.9, 129.3, 128.8, 128.7, 126.9, 116.1, 116.0, 113.2, 106.5 (Ar-C + C = C), 66.5 (CH2Ph), 45.2 (CH2NH), 18.9 (CH3). HRMS m/z for C20H18N2O5 [M + H]+ calcd. 367.1, found 367.1; [M + Na]+ calcd. 389.1, found 389.1.
(S)-benzyl (1-((4-methyl-2-oxo-2H-chromen-7-yl)amino)-1-oxopropan-2-yl)carbamate, 6
Yellow solid (84%); silica gel TLC Rf = 0.65 (MeOH/CH2Cl2 5% v/v); mp 175–177 °C; 1H NMR (DMSO-d6, 400 MHz) δ 10.48 (s, 1H, NH), 7.81–7.73 (m, 3H, Ar–H + NH), 7.55 (d,1H, Ar–H, J = 8.0 Hz), 7.41–7.35 (m, 5H, Ar–H), 6.31 (s, 1H, =CH–CO), 5.08 (s, 2H, CH2Ph), 4.26 (t, 1H, CHNH, J = 8,0 Hz), 2.44 (s, 3H, CH3), 1.35 (d, 3H, CHCH3, J = 8.0 Hz). 13C NMR (DMSO-d6, 400 MHz) δ 173.2 (COCH2NH), 160.9 (O–C=O), 157.0 (COOCH2Ph), 154.5, 154.0, 143.2, 129.2, 128.7, 128.6, 126.8, 116.1, 116.0, 113.2, 106.4 (Ar–C + C = C), 66.4 (CH2Ph), 51.9 (CH2NH), 18.9 (CH3), 18.8 (CHCH3). HRMS m/z for C21H20N2O5 [M + H]+ calcd. 381.1, found 381.1; [M + Na]+ calcd. 403.1, found 403.1.
General method for the synthesis of amino acid-quinolin-2-one conjugates, 7–10
A mixture of equivalent amounts of the appropriate N-protected aminoacylbenzotriazole and 7-amino-1,2,3,4-tetrahydro-2-quinolinone was subjected to microwave irradiation (100 W, 70 °C) in anhydrous THF (5 mL) for 30 min. After completion of the reaction, all volatiles were removed by rotary evaporator and the obtained crude product was crystallized from methanol.
Benzyl (2-oxo-2-((2-oxo-1,2,3,4-tetrahydroquinolin-7-yl)amino)ethyl)carbamate, 7
White solid (97%); silica gel TLC Rf = 0.81 (MeOH/CH2Cl2 5% v/v); mp 224–225 °C; 1H NMR (DMSO-d6, 400 MHz) δ 10.15 (s, 1H, NHlactam), 9.94 (s, 1H, NH), 7.55 (t,1H, NH, J = 8.0 Hz), 7.42–7.25 (m, 4H, Ar–H), 7.24 (s, 1H, Ar–H), 7.12–7.11 (m, 2H, Ar–H), 5.09 (s, 2H, CH2Ph), 3.82 (d, 2H, CH2NH, J = 8,0 Hz), 2.82 (t, 2H, COCH2, J = 8.0 Hz), 2.44 (t, 2H, COCH2CH2, J = 8.0 Hz). 13C NMR (DMSO-d6, 400 MHz) δ 171.2 (COCH2NH), 168.6 (NH–C=O), 157.5 (COOCH2Ph), 139.4, 138.8, 138.0, 129.3, 128.7, 128.6, 119.3, 113.7, 107.2 (Ar–C), 66.4 (CH2Ph), 44.9 (CH2NH), 31.5 (COCH2), 25.2 (COCH2CH2). HRMS m/z for C19H19N3O4 [M + H]+ calcd. 354.1, found 354.1; [M + Na]+ calcd. 376.1, found 376.1.
tert-butyl (2-oxo-2-((2-oxo-1,2,3,4-tetrahydroquinolin-7-yl)amino)ethyl)carbamate, 8
Beige solid (94%); silica gel TLC Rf = 0.26 (Ethyl acetate/Hexane 30% v/v); mp 135–136 °C; 1H NMR (DMSO-d6, 400 MHz) δ 10.13 (s, 1H, NHlactam), 9.87 (s, 1H, NH), 7.24 (s, 1H, Ar–H), 7.10 (d, 2H, Ar–H, J = 4.0 Hz), 7.02 (t,1H, NH, J = 4.0 Hz), 3.72 (d, 2H, CH2NH, J = 4,0 Hz), 2.83 (t, 2H, COCH2, J = 4.0 Hz), 2.45 (t, 2H, COCH2CH2, J = 4.0 Hz), 1.43 (s, 9H, (CH3)3C). 13C NMR (DMSO-d6, 400 MHz) δ 171.2 (COCH2NH), 168.9 (NH–C=O), 156.8 (COOCH2Ph), 139.4, 138.8, 119.3, 113.7, 107.1,(Ar–C), 79.0 (CO(CH3)3), 44.6 (CH2NH), 31.6 (COCH2), 29.1 (COCH2CH2), 25.2 (C(CH3)3). HRMS m/z for C16H21N3O4 [M]+ calcd. 319.1, found 319.1; [M + Na]+ calcd. 342.1, found 342.1.
(S)-benzyl (1-oxo-1-((2-oxo-1,2,3,4-tetrahydroquinolin-7-yl)amino)propan-2-yl)carbamate, 9
White solid (96%); silica gel TLC Rf = 0.63 (MeOH/CH2Cl2 5% v/v); mp 220–221 °C; 1H NMR (DMSO-d6, 400 MHz) δ 10.14 (s, 1H, NHlactam), 9.94 (s, 1H, NH), 7.58 (d,1H, NH, J = 8.0 Hz), 7.40–7.36 (m, 5H, Ar–H), 7.26 (s, 1H, Ar–H), 7.13–7.09 (m, 2H, Ar–H), 5.07 (s, 2H, CH2Ph), 4.22 (t, 1H, CH2NH, J = 8,0 Hz), 2.84 (t, 2H, COCH2, J = 8.0 Hz), 2.45 (t, 2H, COCH2CH2, J = 8.0 Hz), 1.31 (d, 3H, CH3, J = 8.0 Hz). 13C NMR (DMSO-d6, 400 MHz) δ 171.3 (COCH2NH), 171.2 (NH–C=O), 156.7 (COOCH2Ph), 139.4, 138.9, 138.0, 129.3, 128.7, 128.6, 119.4, 113.8, 107.3, (Ar–C), 66.3 (CH2Ph), 51.6 (CH2NH), 31.6 (COCH2), 25.2 (COCH2CH2), 19.0 (CH3). HRMS m/z for C20H21N3O4 [M + H]+ calcd. 368.1, found 368.1; [M + Na]+ calcd. 390.1, found 390.1.
(S)-benzyl (1-oxo-1-((2-oxo-1,2,3,4-tetrahydroquinolin-7-yl)amino)-3-phenylpropan-2-yl)carbamate, 10
White solid (98%); silica gel TLC Rf = 0.58 (MeOH/CH2Cl2 5% v/v); mp 150–151 °C; 1H NMR (DMSO-d6, 400 MHz) δ 10.17 (s, 1H, NHlactam), 10.10 (s, 1H, NH), 7.95 (d,1H, NH, J = 4.0 Hz), 7.38–7.12 (m, 13H, Ar–H), 5.00 (s, 2H, CH2Ph), 4.22 (m, 1H, CHNH), 3.05 and 2.91 (m, 2H, CHCH2Ph), 2.86 (t, 2H, COCH2, J = 8.0 Hz), 2.46 (t, 2H, COCH2CH2, J = 8.0 Hz). 13C NMR (DMSO-d6, 400 MHz) δ 171.3 (COCH2NH), 171.2 (NH–C=O), 156.9 (COOCH2Ph), 139.4, 138.8, 138.7, 138.0, 130.2, 129.2, 129.0, 128.7, 128.6, 128.5, 127.3, 119.5, 114.0, 107.4 (Ar–C), 66.2 (CH2Ph), 57.8 (CHNH), 38.5 (CHCH2Ph, 31.6 (COCH2), 25.2 (COCH2CH2). HRMS m/z for C26H25N3O4 [M + H]+ calcd. 444.1, found 444.1; [M + Na]+ calcd. 466.1, found 466.1.
CA inhibition
An Applied Photophysics stopped-flow instrument was used for assaying the CA catalyzed CO2 hydration activityCitation27. Phenol red (at 0.2 mM) was used as indicator, working at the absorbance maximum of 557 nm with 10 mM HEPES (pH 7.5) as buffer and 0.1 M NaClO4 (for maintaining constant ionic strength), at 20 °C, following the CA-catalyzed CO2 hydration reaction for a period of 10–100 s (the uncatalyzed reaction needs around 60–100 s in the assay conditions, whereas the catalyzed ones are of around 6–10 s). The CO2 concentrations ranged from 1.7 to 17 mM for the determination of the kinetic parameters. The uncatalyzed rates were determined in the same manner and subtracted from the total observed rates. Enzyme and inhibitor were incubated together for 6 h prior to assay, as for all other couamrin/sulfocoumarin derivatives investigated earlierCitation1,5–10. Enzyme concentrations in the assay system were in the range of 8–16 nM for all the enzymes considered in this study. All CAs were recombinant proteins, obtained as reported earlier by our group Citation28–31.
Results and discussion
The new N-protected amino acid–coumarin conjugates (1–6) were synthesized by the treatment of the appropriate coumarin derivatives with N-protected aminoacylbenzotriazole under microwave heating at 70 °C for 30 min. They were obtained in good yields, of 84–96% (Scheme 1). New N-protected amino acid–quinolinone conjugates (7–10) were also synthesized by a similar method mentioned above for the coumarin conjugates, using appropriately N-protected aminoacylbenzotriazoles and 7-amino-1,2,3,4-tetrahydro-2-quinolinoneCitation32 under microwave heating at 70 °C for 30 min with good yields of 94–98%. The synthesis pathways of the N-protected amino acid–coumarin or –quinolinone derivatives (1–10) are summarized in Scheme 1.
Scheme 1. Synthesis pathways of the new coumarin and quinolinone conjugates of N-protected amino acids.
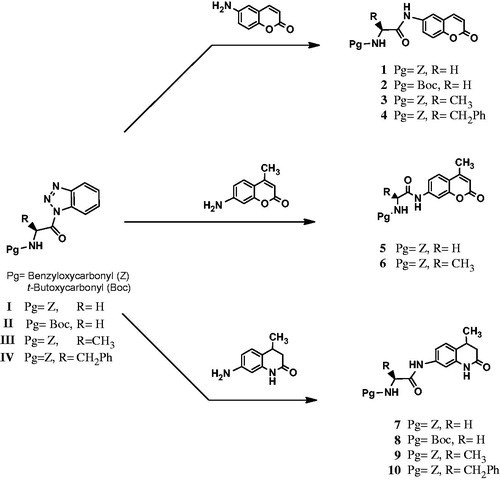
The structures of the N-protected amino acid–coumarin/quinolinone conjugates (1–10) were elucidated by 1H NMR, 13C NMR and mass spectrometry (MS) analyses. All spectral data were in accordance with the assumed structures (see “Materials and Methods” section). The characteristic NH resonances for the coumarin part of the conjugates were observed between 10.17 and 10.48 ppm. Other amino group resonances for the cuomarin conjugates were observed between 7.11 and 7.98 ppm. Carbonyl resonances of the amide carbonyl, lactone carbonyl and carba-mate carbonyl were observed around 169.1–173.2, 169.0 and 156.8–157.6 ppm, respectively. The characteristic NH resonances for carbamate and quinolinone part of the conjugates were observed between 10.13–10.17 and 9.87–10.10 ppm, respectively. Other amino group resonances for the quinolinone conjugates were observed between 7.02 and 7.95 ppm. Carbonyl resonances of the amide carbonyl, lactame carbonyl and carbamate carbonyl were observed around 171.2–173.3, 168.9–171.2 and 156.7–157.5 ppm, respectively. All other aliphatic and aromatic protons and carbons were observed at expected regions.
The hCA I, II, IV and XII enzyme inhibitory activity results of the coumarin/quinolinone amino acid conjugates are shown in
Table 1. hCA I, II, IV and XII inhibition with amino acid conjugates reported in the paper, by a stopped-flow, CO2 hydrase assay, after 6 h ioncubation period between enzyme and inhibitorCitation27.
Data of show that the quinolinone conjugates 7–10 were totally inactive as CAIs, although the parent compound, 7-amino-1,2,3,4-tetrahydro-2-quinolinoneCitation32 showed interesting such properties. On the other hand, the coumarin derivatives showed interesting enzyme inhibitory properties. As many other coumarinsCitation1,5–10, they did not inhibit the cytosolic, off-target isoforms hCA I and II (KIs > 50 μM) but showed submicromolar affinity for the membrane-associated (hCA IV) and transmembarne (hCA XII) isoforms. Indeed, against hCA IV apart 2 and 6 which was inactive, the other derivatives showed inhibition constants in the range of 92 nM–1.19 μM, with the best activity being shown by the benzyloxycarbonyl-protected (Cbz) Gly derivative 1. Interestingly, the corresponding Boc-protected derivative 2 was ineffective as an hCA IV inhibitor, whereas the inhibitory activity diminished for the remaining Cbz-protected Ala and Phe derivatives 3 and 4. Thus, bulkier amino acyl moieties than the Gly one were detrimental in this position of the coumarin ring for the hCA IV inhibitory activity.
Against hCA XII the new coumarin conjugates showed an effective inhibitory power, with KIs ranging between 0.11 and 0.79 μM (). The best inhibitor was 6 (KI of 0.11 μM) which incorporates the amino acyl moiety in position 7 of the coumarin ring, and contains a Cbz–Ala moiety. The corresponding Gly derivative was around 5 times less effective as a hCA XII inhibitor, which is very different from the hCA IV inhibition discussed above.
The 6-substituted coumarins showed on the other hand a rather similar behavior of submicromolar hCA XII inhibitors, with a relatively small variation of the inhibition constants with the scaffold they incorporate. Indeed, both Boc- and Cbz-protected compounds incorporating Gly, Ala and Phe moieties had KIs ranging only between 0.23 and 0.79 μM ().
Conclusions
Several N-Protected amino acids, such as Boc- or Cbz-protected Gly, Ala and Phe, were reacted with amino-substituted coumarin and quinolinone derivatives, leading to the corresponding N-protected amino acid–coumarin/quinolinone conjugates. The CA inhibitory activity of the new compounds was assessed against various isoforms, such as hCA I, hCA II, hCA IV and hCA XII. The quinolinone conjugates were inactive as enzyme inhibitors, whereas the coumarins were ineffective hCA I/II inhibitors (KIs > 50 μM) but were submicromolar hCA IV and XII inhibitors, with inhibition constants ranging between 92 nM and 1.19 μM for hCA IV, and between 0.11 and 0.79 μM for hCA XII. These coumarin derivatives, as many others reported earlier, thus show an interesting selective inhibitory profile for the membrane-bound over the cytosolic CA isoforms.
Acknowledgements
We thank İnönü University, Turkey (BAPB), and Università degli Studi di Firenze, Italy, for financial support.
Declaration of interest
The authors declare no conflict of interest.
References
- Maresca A, Temperini C, Vu H, et al. Non-zinc mediated inhibition of carbonic anhydrases: coumarins are a new class of suicide inhibitors. J Am Chem Soc 2009;131:3057–62
- Lei L, Xue Y, Liu Z, et al. Coumarin derivatives from Ainsliaea fragrans and their anticoagulant activity. Sci Rep 2015;5:13544
- Vats P, Hadjimitova V, Yoncheva K, et al. Chromenone and quinolinone derivatives as potent antioxidant agents. Med Chem Res 2014;23:4907–14
- Sharma A, Tiwari M, Supuran CT. Novel coumarins and benzocoumarins acting as isoform-selective inhibitors against the tumor-associated carbonic anhydrase IX. J Enzyme Inhib Med Chem 2014;29:292–6
- Maresca A, Temperini C, Pochet L, et al. Deciphering the mechanism of carbonic anhydrase inhibition with coumarins and thiocoumarins. J Med Chem 2010;53:335–44
- Maresca A, Supuran CT. Coumarins incorporating hydroxy- and chloro-moieties selectively inhibit the transmembrane, tumor-associated carbonic anhydrase isoforms IX and XII over the cytosolic ones I and II. Bioorg Med Chem Lett 2010;20:4511–14
- Maresca A, Scozzafava A, Supuran CT. 78-Disubstituted but not 6,7-disubstituted coumarins selectively inhibit the transmembrane, tumor-associated carbonic anhydrase isoforms IX and XII over the cytosolic ones I and II in the low nanomolar/subnanomolar range. Bioorg Med Chem Lett 2010;20:7255–8
- Touisni N, Maresca A, McDonald PC, et al. Glycosyl coumarin carbonic anhydrase IX and XII inhibitors strongly attenuate the growth of primary breast tumors. J Med Chem 2011;54:8271–7
- Lou Y, McDonald PC, Oloumi A, et al. Targeting tumor hypoxia: suppression of breast tumor growth and metastasis by novel carbonic anhydrase IX inhibitors. Cancer Res 2011;71:3364–76
- Bonneau A, Maresca A, Winum JY, Supuran CT. Metronidazole-coumarin conjugates and 3-cyano-7-hydroxy-coumarin act as isoform-selective carbonic anhydrase inhibitors. J Enzyme Inhib Med Chem 2013;28:397–401
- Supuran CT. Carbonic anhydrases: novel therapeutic applications for inhibitors and activators. Nat Rev Drug Discov 2008;7:168–81
- Supuran CT. Structure-based drug discovery of carbonic anhydrase inhibitors. J Enzyme Inhib Med Chem 2012;27:759–72
- Supuran CT. Carbonic anhydrases: from biomedical applications of the inhibitors and activators to biotechnological use for CO(2) capture. J Enzyme Inhib Med Chem 2013;28:229–30
- Capasso C, Supuran CT. Anti-infective carbonic anhydrase inhibitors: a patent and literature review. Expert Opin Ther Pat 2013;23:693–704
- Capasso C, Supuran CT. An overview of the alpha-, beta- and gamma-carbonic anhydrases from Bacteria: can bacterial carbonic anhydrases shed new light on evolution of bacteria? J Enzyme Inhib Med Chem 2015;30:325–32
- Supuran CT, Capasso C. The η-class carbonic anhydrases as drug targets for antimalarial agents. Expert Opin Ther Targets 2015;19:551–63
- Capasso C, Supuran CT. Sulfa and trimethoprim-like drugs — antimetabolites acting as carbonic anhydrase, dihydropteroate synthase and dihydrofolate reductase inhibitors. J Enzyme Inhib Med Chem 2014;29:379–87
- Supuran CT. Carbonic anhydrase inhibitors: an editorial. Expert Opin Ther Pat 2013;23:677–9
- Del Prete S, Vullo D, De Luca V, et al. Biochemical characterization of recombinant beta-carbonic anhydrase (PgiCAb) identified in the genome of the oral pathogenic bacterium Porphyromonas gingivalis. J Enzyme Inhib Med Chem 2015;30:366–70
- Del Prete S, Vullo D, De Luca V, et al. Biochemical characterization of the δ-carbonic anhydrase from the marine diatom Thalassiosira weissflogii, TweCA. J Enzyme Inhib Med Chem 2014;29:906–11
- Panda SS, Hall CD, Scriven E, Katritzky AR. Aminoacyl benzotriazolides: versatile reagents for the preparation of peptides and their mimetics and conjugates. Aldrichimica Acta 2013;46:43–5
- Panda SS, Ibrahim MA, Küçükbay H, et al. Synthesis and antimalarial bioassay of quinine – peptide conjugates. Chem Biol Drug Des 2013;82:361–6
- Khatib ME, Jauregui L, Tala SR, et al. Solution-phase synthesis of chiral O-acyl isodipeptides. MedChemComm 2011;2:1087–92
- Ibrahim MA, Panda SS, Oliferenko AA, et al. Macrocyclic peptidomimetics with antimicrobial activity: synthesis, bioassay, and molecular modeling studies. Org Biomol Chem 2015;13:9492–503
- Wu X, Chen Y, Aloysius H, Hu L. A novel high-yield synthesis of aminoacyl p-nitroanilines and aminoacyl 7-amino-4-methylcoumarins: important synthons for the synthesis of chromogenic/fluorogenic protease substrates. Beilstein J Org Chem 2011;7:1030–5
- Pozdnev VF. Activation of carboxylic acids by pyrocarbonates. Synthesis of arylamides of N-protected amino acids and small peptides using dialkyl pyrocarbonates as condensing reagents. Int J Pept Protein Res 1994;44:36–48
- Khalifah RG. The carbon dioxide hydration activity of carbonic anhydrase. I. Stop-flow kinetic studies on the native human isoenzymes B and C. J Biol Chem 1971;246:2561–73
- Carta F, Temperini C, Innocenti A, et al. Polyamines inhibit carbonic anhydrases by anchoring to the zinc-coordinated water molecule. J Med Chem 2010;53:5511–22
- Casini A, Scozzafava A, Mincione F, et al. Carbonic anhydrase inhibitors: water-soluble 4-sulfamoylphenylthioureas as topical intraocular pressure-lowering agents with long-lasting effects. J Med Chem 2000;43:4884–92
- Fabrizi F, Mincione F, Somma T, et al. A new approach to antiglaucoma drugs: carbonic anhydrase inhibitors with or without NO donating moieties. Mechanism of action and preliminary pharmacology. J Enzyme Inhib Med Chem 2012;27:138–47
- Supuran CT, Ilies MA, Scozzafava A. Carbonic anhydrase inhibitors. Part 29. Interaction of isozymes I, II and IV with benzolamide-like derivatives. Eur J Med Chem 1998;33:739–52
- Isik S, Vullo D, Bozdag M, et al. 7-Amino-3,4-dihydro-1H-quinolin-2-one, a compound similar to the substituted coumarins, inhibits α-carbonic anhydrases without hydrolysis of the lactam ring. J Enzyme Inhib Med Chem 2015;30:773–7