Abstract
Ischemia of brain areas is a global health problem, causing death or long-term disability. Current pharmacological options have limited impact on ischemic damages. Recently, a relationship between hypoxia and carbonic anhydrase (CA) over-expression has been highlighted suggesting CA inhibition as a possible target. This study aimed to evaluate the pharmacological profile of sulfonamide and coumarin CA inhibitors in rats underwent permanent middle cerebral artery occlusion (pMCAO). The neurological score of pMCAO rats was dramatically reduced 24 h after occlusion. Repeated subcutaneous injections of the CA inhibitors 4 and 7 (1 mg kg−1) were able to increase the neurological score by 40%. Compound 7 showed the tendency to reduce the volume of hemisphere infarction. The standard CA inhibitor acetazolamide was ineffective. The properties of novel CA inhibitors to improve neurological functionalities after cerebral ischemic insult are shown. The CA involvement in cerebral hypoxic phenomena deserves deeper investigations.
Introduction
Cerebral ischemia occurs when blood flow to the brain is insufficient to meet the metabolic demand. Occlusion of cerebral arteries interrupts blood flow, limits oxygen and glucose supply to the central nervous system (CNS) and causes infarction/ischemic strokeCitation1. Preclinical results individuate candidate targets for CNS protection: glutamate release, glutamate receptor, excitotoxicity, mitochondrial dysfunction, nitric oxide, inflammation, necrosis, apoptosis and redox unbalance. In spite of advances in the pathophysiology of cerebral ischemia, therapeutic options remain very limitedCitation2.
Up to now, only one pharmacological treatment for acute ischemic stroke was approved by FDA, the intravenous administration of recombinant tissue plasminogen activator (rt-PA)Citation3. rt-PA delivered within 3 h after the onset of symptoms, reduces neurological deficits and improves functional outcome in stroke patients. Unfortunately, the large majority of patients with ischemic stroke are not able to reach hospital within 3 h, as a result rt-PA can be exploitable by a very limited number of patients and most of them do not receive treatmentCitation4. Consequently, successful treatments of ischemic stroke remain a major challenge in clinical practice.
The evidence that hypoxic microenvironments elicit the expression of specific isoforms of carbonic anhydrase (CA) (in particular CAIX and CAXII) through the hypoxia inducible factorCitation5,Citation6 allows to hypothesize a possible CA relevance in ischemia.
The aim of this study was the evaluation of the pharmacological profile of a new class of CA inhibitors in a rat model of cerebral ischemia.
Materials and methods
Chemistry
Anhydrous solvents and all reagents were purchased from Sigma-Aldrich (Milan, Italy), Alfa Aesar (Milan, Italy) and TCI (Milan, Italy). All reactions involving air- or moisture-sensitive compounds were performed under a nitrogen atmosphere using dried glassware and syringes techniques to transfer solutions. Nuclear magnetic resonance (1H NMR, 13C NMR) spectra were recorded using a Bruker Advance III 400 MHz spectrometer in DMSO-d6 or CDCl3. Chemical shifts are reported in parts per million (ppm) and the coupling constants (J) are expressed in Hertz (Hz). Splitting patterns are designated as follows: s, singlet; d, doublet; sept, septet; t, triplet; q, quadruplet; m, multiplet; brs, broad singlet; dd, double of doublets. The assignment of exchangeable protons (OH and NH) was confirmed by the addition of D2O. Analytical thin-layer chromatography (TLC) was carried out on Merck silica gel F-254 plates. Flash chromatography purifications were performed on Merck Silica gel 60 (230–400 mesh ASTM) as the stationary phase and ethyl acetate/n-hexane were used as eluents. Melting points (mp) were measured in open capillary tubes with a Gallenkamp MPD350.BM3.5 apparatus and are uncorrected. All compounds reported here were >95% HPLC pure.
Synthesis of 7-(prop-2-ynyloxy)-2H-chromen-2-one 2Citation7
Hydroxy coumarin 1 (1.0 g, 1.0 eq), propargyl alcohol (1.0 eq) and triphenylphoshine (1.0 eq) were dissolved in dry THF (90 ml). Then the temperature was lowered to 0 °C and diisopropylazadicarboxylate (DIAD; 1.1 eq) was added drop-wise under sonication. The orange solution was sonicated at r.t. under a nitrogen atmosphere until starting material was consumed (TLC monitoring). Solvents were removed under vacuo to give a white solid that was recrystallized from MeOH to afford the titled compound as white solid.
7-(Prop-2-ynyloxy)-2H-chromen-2-one 2
65% yield; m.p. 118 °C (Lit.Citation6 120 °C); silica gel TLC Rf 0.53 (Ethyl acetate/n-hexane 50% v/v); νmax (KBr) cm−1, 3310 (C ≡ C–H), C2160 (C ≡ CH), 1765 (C = O), 1604 (Aromatic); δH (400 MHz, DMSO-d6) 3.69 (1H, t, J 2.4, 3′-H), 4.97 (2H, d, J 2.4, 1′-H2), 6.36 (1H, d, J 9.6, 3-H), 7.03 (1H, dd, J 8.5, 2.3, 6-H), 7.09 (1H, d, J 2.3, 8-H), 7.69 (1H, d, J 8.5, 5-H), 8.03 (1H, d, J 9.6, 4-H); δC (100 MHz, DMSO-d6) 161.1 (C-2), 161.0 (C-7), 156.0 (C-8a), 145.1 (C-4), 130.4 (C-5), 113.9 (C-3), 113.8 (C-4a), 113.7 (C-6), 102.7 (C-8), 79.8 (C-2′), 79.4 (C-3′) and 57.0. Experimental in agreement with reported dataCitation8.
Synthesis of 2-bromophenylazide 3Citation9
The commercially available 2-bromo aniline (0.5 g, 1.0 eq) was dissolved in a 2 -M aqueous hydrochloric acid solution (15 ml) at 0 °C and then was treated with NaNO2 (1.2 eq) and NaN3 (1.5 eq) dissolved in H2O (15 ml each). The reaction mixture was stirred at r.t. for 15 min and then extracted with ethyl acetate (2 × 15 ml) and the combined organic layers were dried over Na2SO4, filtered-off and the solvent evaporated in vacuo to afford the titled compound which was used without further purification.
2-Bromophenylazide 3
89% yield; δH (400 MHz, CDCl3) 7.55 (app d, J = 8.0 Hz, 1H), 7.35 (app t, J = 7.7 Hz, 1H), 7.17 (app d, J = 8.0 Hz, 1H), 7.01 (app t, J = 7.7 Hz, 1H); δC NMR (100 MHz, CDCl3) 138.6, 133.8, 128.5, 125.9, 119.4, 113.8. Experimental in agreement with reported dataCitation9.
Synthesis of 7-[(1′-(2-bromophenyl)-1H-1′,2′,3′-triazol-4′-yl]methoxy)-2H-chromen-2-one 4Citation10
Freshly prepared 1-azido-2-bromobenzene 3 (0.44 g, 1.1 eq) and 7-(prop-2-ynyloxy)-2H-chromen-2-one 2 (0.4 g, 1.0 eq) were dissolved in tert-ButOH/H2O 1/1 (2.0 ml) and then TMACl (0.4 g, 1.0 eq) and copper(0) nanosize (5% mol) were added. The suspension was stirred at 60 °C until starting materials were consumed (TLC monitoring), then quenched with H2O (10 ml) and the formed precipitate was filtered-off. The obtained residue was purified by silica gel column chromatography eluting with 33% ethyl acetate in n-hexane to afford 4 as a white solid.
7-[(1′-(2-Bromophenyl)-1H-1′,2′,3′-triazol-4′-yl]methoxy)-2H-chromen-2-one 4
Fifty-percent yield; m.p. 133–134 °C; silica gel TLC Rf 0.16 (Ethyl acetate/n-hexane 33% v/v); νmax (KBr) cm−1, 1770 (C = O), 1560 (aromatic); δH (400 MHz, DMSO-d6) 5.41 (2H, s, 1″-H2), 6.35 (1H, d, J 9.6, 3-H), 7.11 (1H, dd, J 8.8, 2.4, 6-H), 7.26 (1H, d, J 2.4, 8-H), 7.67 (4H, m, Ar-H), 7.96 (1H, dd, J 8.8, 2.4, 5-H), 8.04 (1H, d, J 9.6, 4-H), 8.78 (1H, s, 5′-H); δc (100 MHz, DMSO-d6) 162.0, 161.2, 156.2, 145.2, 142.9, 137.0, 134.5, 133.0, 130.5, 129.9, 129.7, 128.1, 119.8, 113.9, 113.7, 113.6, 102.6, 62.4.
CA inhibition
An Applied Photophysics stopped-flow instrument (Leatherhead, UK) has been used for assaying the CA catalyzed CO2 hydration activityCitation11. Phenol red (at a concentration of 0.2 mM) has been used as indicator, working at the absorbance maximum of 557 nm, with 20 mM Hepes (pH 7.5) as buffer, and 20 mM Na2SO4 (for maintaining constant the ionic strength), following the initial rates of the CA-catalyzed CO2 hydration reaction for a period of 10–100 s. The CO2 concentrations ranged from 1.7 to 17 mM for the determination of the kinetic parameters and inhibition constants. For each inhibitor at least six traces of the initial 5–10% of the reaction have been used for determining the initial velocity. The uncatalyzed rates were determined in the same manner and subtracted from the total observed rates. Stock solutions of inhibitor (0.1 mM) were prepared in distilled-deionized water and dilutions up to 0.01 nM were done thereafter with the assay buffer. Inhibitor and enzyme solutions were preincubated together for 15 min – 6 h at room temperature (15 min) or 4 °C (6 h) prior to assay, in order to allow for the formation of the E-I complex. Data from were obtained after 6 h incubation of enzyme and inhibitor. The inhibition constants were obtained by non-linear least-squares methods using PRISM 3 and the Cheng-Prusoff equation, as reported earlierCitation8,Citation11, and represent the mean from at least three different determinations. All CA isoforms were recombinant ones obtained in-house as reported earlierCitation12–16.
Table 1. hCA I, II, VII, IX and XII inhibition data of the newly synthesized compounds 4–7 by a stopped flow CO2 hydrase assayCitation11. 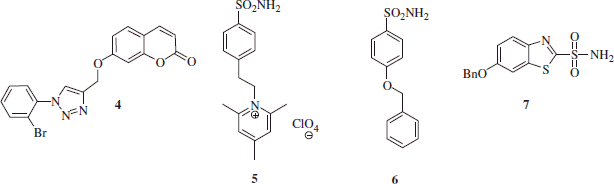
Animals
Male Sprague–Dawley rats (Harlan, Varese, Italy), weighing 200–250 g at the beginning of the experimental procedure, were used for all the experiments. Animals were housed in the Centro Stabulazione Animali da Laboratorio, University of Florence and used no earlier than 1 week after their arrival. Four rats were housed per cage (size 2 6 × 41 cm); animals were fed with standard laboratory diet and tap water ad libitum, and kept at 2 3 ± 1 °C with a 12-h light/dark cycle, light at 7a.m. All animal manipulations were carried out according to the European Community guidelines for animal care (DL 116/92), application of the European Communities Council Directive of 24 November 1986 (86/609/EEC). The ethical policy of the University of Florence complies with the Guide for the Care and Use of Laboratory Animals of the US National Institutes of Health (NIH Publication No. 85-23, revised 1996; University of Florence assurance number: A5278-01). Formal approval to conduct the experiments described was obtained from the Animal Subjects Review Board of the University of Florence. Experiments involving animals have been reported according to ARRIVE guidelinesCitation17. All efforts were made to minimize animal suffering and to reduce the number of animals used.
Permanent middle cerebral artery occlusion (pMCAO) in rats
pMCAO was induced by using a previously described, relatively non-invasive techniqueCitation18 in Sprague–Dawley rats. In these experiments, the animals were randomly picked up from the cages and allocated to the vehicle or treated groups. The researchers were aware of the treatment allocation. Anesthesia was induced with 2% isoflurane in air and maintained with the lowest active concentration of anesthetic. Body temperature was measured with a rectal probe and kept at 37 °C with a heating pad. The external and internal right carotid arteries were dissected under an operating microscope, and a silk suture was tied loosely around the external carotid stump. A silicone-coated nylon filament (diameter: 0.28 mm) was then inserted through the external into internal carotid artery up to the Willis circle to occlude the right middle cerebral artery. The silk suture was finally tightened around the intraluminal filament to prevent bleeding. To maintain the animals at standard temperature conditions, a heating pad was used for the entire duration of the surgery. In this pMCAO severe model, the nylon filament was not remove and the animals were killed 24 h after surgery.
Compound administration protocol
Acetazolamide (AAZ; 30–60 mg kg−1) was suspended in 1% carboxymethilcellulose or dissolved in 1% DMSO/1% PEG/0.2% TWEEN 80 and per os or subcutaneously (s.c.) administered, respectively. The AAZ injections were performed 30 min before, 15 and 120 min after pMCAO. Compounds (1 mg kg−1) were dissolved in 1% DMSO/1% PEG/0.2% TWEEN 80 and s.c. administered at 5 and 20 min after the induction of cerebral ischemia.
Neurological test
Neurological evaluation of motor sensory functions was carried out 24 h after pMCAO. The examiners were blind as to the procedure that the rat had undergone. Evaluations were always performed between 10 and 11 a.m. to exclude behavioral changes based on circadian rhythm. The neurological examination consisted of six testsCitation19,Citation20:
Spontaneous activity
Symmetry in the movement of four limbs
Forepaw outstretching
Climbing
Body proprioception
Response to vibrisse touch.
The score assigned to each rat at completion of the evaluation equals the sum of all six test scores. The final minimum score was 3, and the maximum was 18.
Evaluation of infarct volume
Twenty-four hour after injury, rats were killed and their brains were carefully removed. The brains were cut into five coronal slices of 2-mm thickness. Slices were incubated in 2% solution of 2,3,5-triphenyltetrazolium chloride (TTC) at 37 °C for 30 min and immersion fixed in 10% buffered formalin solution. TTC stains the viable brain tissue red, while infracted tissue remains unstainedCitation21,Citation22. For quantification of infracted area and volumes, the brain slices were photographed using a digital camera and the Image analysis was performed on a personal computer with an image analysis software program (using ImageJ for Windows)Citation23. To compensate the effect of brain edema which distorts and enlarges the infarcted tissue and surrounding white matter, infarct areas were calculated subtracting the area of intact tissue in the ipsilateral hemisphere from the area of the contralateral hemisphere. Infarct volumes were calculated multiplying the infarct area by the distance among sections (2 mm) as described previouslyCitation24.
Statistical analyses
Statistically significant differences in the neurological scores among vehicle-treated, CA inhibitors-treated and sham-operated rats were evaluated by one-way ANOVA followed by the post hoc Newman–Keuls multiple comparison test. Statistically significant differences in brain damage were evaluated by unpaired Student’s t-test. At least five rats were examined per group. A p value of <0.05 was considered significant.
Results
Three sulfonamide and one coumarin CA inhibitors (CAIs) were included in the study ()Citation25,Citation26. Except the coumarin 4, which is reported here for the first time (see “Materials and methods” section for details), the other compounds were reported earlier by our groupsCitation26.
The main differences between coumarins and sulfonamide CAIs is that the first class tends to show isoform selective inhibition profileCitation27–30, whereas the sulfonamides generally inhibit indiscriminately most CA isoformsCitation31,Citation32.
This is also observed for the data of : indeed the coumarine 4 is a hCA VII-selective inhibitor (with an inhibition constant of 15.3 nM against this brain-specific isoform), being an ineffective inhibitor of isoforms hCA I, II (cytosolic) as well as IX and XII (transmembrane CAs).
The positively charged sulfonamide 5 is a membrane-impermeant, pyridinium derivative which does not cross plasma membranesCitation33. This compound is a poor hCA I inhibitor but effectively inhibits CA II, VII, IX and XII (), with KIs ranging between 7 and 21 nM. The remaining two sulfonamides, 6 and 7, are lipophilic, membrane-permeant derivatives. They are effective inhibitors of all five CA isoforms investigated here (except compound 6 against hCA I), with inhibition constants between 0.89 and 48.1 nM. As all these five isoforms are presumably present in the brain, and two of them (CA IX and XII) may be over-expressed in hypoxic conditions, it may be rather difficult to assess the role of precise CA isoforms in ischemic processes, and this was also the reason why compounds with such a variable inhibition profile were included in this study. AAZ, the classical, standard sulfonamide CAI was also included in the study as a control compound and its inhibition profile are also shown in .
The neurological score was evaluated in sham operated and in pMCAO rat groups 24 h after occlusion (). The neurological score of pMCAO vehicle-treated rats was significantly reduced (9.5 ± 1.3) in comparison to sham-operated rats (17.5 ± 0.2). AAZ, administered per os (50 mg kg−1) or subcutaneously (30 mg kg−1) 30 min before, 15 and 120 min after occlusion did not improve the neurological deficit induced by cerebral stroke. Repeated subcutaneous injections, 5 and 20 min after surgery, of the CAIs 4 and 7 (both 1 mg kg−1) were able to significantly increase the neurological score up to 40%. The other two compounds tested (5 and 6) did not reduce the neurological damages induced by cerebral ischemia.
Figure 1. Evaluation of neurological score. Measurements were carried out 24 h after pMCAO. The protocol consisted of six tests in order to evaluate spontaneous activity, symmetry in the movement of four limbs, forepaw outstretching, climbing, body proprioception and response to vibrisse touch. The score assigned to each rat at completion of the evaluation equals the sum of all six test scores. The final minimum score was 3, and the maximum was 18. Each value represents the mean ± SEM of five rats per group. **p < 0.01 versus sham + vehicle-treated rats; ^p < 0.05 versus pMCAO + vehicle-treated rats.
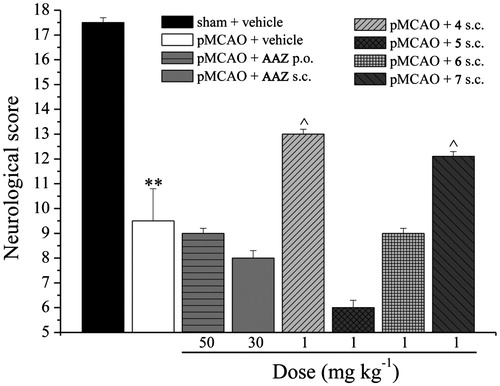
The cerebral infarction was examined on slices obtained from cerebrum 24 h after pMCAO (). pMCAO-vehicle-treated rats showed a large infarct volume in comparison to sham-operated rats (data not shown). The treatments with the analyzed compounds were not able to significantly reduce the volume of total hemisphere infarction 24 h after occlusion in comparison to pMCAO + vehicle group. Nevertheless, animals treated with 7 induced a tendency to infarct volume decrease ().
Figure 2. Effect of CA inhibitors on infarct volume after pMCAO. The evaluation was performed 24 h after surgery. Coronal brain slices (2 mm) were incubated with TTC and infarct volume was calculated multiplying the infarct area by the distance among sections (2 mm). Each value represents the mean ± SEM of five rats per group.
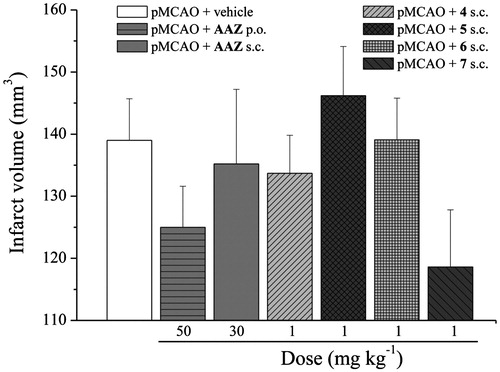
Discussion and conclusions
To the best of our knowledge, this study is the first preclinical evidence of CAI efficacy in cerebral ischemia treatment. Four synthetic CAIs 4–7 were compared with AAZ about the property to ameliorate the neurological score and the brain infarct volume after pMCAO. While AAZ administration was not active neither to increase neurological score nor to reduce brain damage, 4 and 7 (both 1 mg kg−1) were able to significantly improve motor and sensory functions 24 h after injury. Moreover, a tendency to decrease the infarct volume was highlighted after compound 7 treatment.
CA reversibly converts carbon dioxide to H+ and bicarbonate and contributes to several biological processes, including pH regulation, anion transport and water balance. It plays a pivotal role both in physiological and pathological conditions also concerning the CNSCitation34. In astroglia (CA high expressing cellsCitation35), both intracellularly and membrane-bound (acting interstitially) CA isoforms cooperatively couple intercellular CO2 shuttling to the acid/base transporter activitiesCitation36,Citation37. Glial CA converts neuron-derived CO2 to bicarbonate and protons which are driven out of the glial cell by a cotransporter and monocarboxylate transporters. In the extracellular space, CA catalyzes the reaction of bicarbonate with a proton to recycle CO2, which is instrumental for buffering extracellular pHCitation36. pH dysregulation has been related to a spectrum of pathological conditionsCitation38, in particular in vitro studies showed that hypoxia/anoxia decreases pH in neurons and glial cellsCitation38 possibly by alterations in the Na+/H+ exchange activityCitation39,Citation40. Moreover, the lack of oxygen caused by hypoxia leads to a switch from aerobic to anaerobic glucose metabolism, characterized by increased production of lactic acid and a lowered intracellular pHCitation41. CAIs intervene to reduce H+ concentration contributing to the pH homeostasisCitation42,Citation43.
Moreover, the CA ability to regulate the volume of body fluidsCitation42 offers a further opportunity to modulate ischemia-induced damages. The presence of edema is a key aggravating factor in ischemic stroke, brain volume is limited by the rigidity of the skull, so that even little volume increases lead to high intracranial pressure and compression of neural tissue and vasculatureCitation44. The reduction of edema can mitigate the brain damages induced by cerebral ischemia. Inhibitors of CA are currently used to reduce body fluid volume and edema in pathologies like glaucomaCitation45,Citation46, congestive heart failure induced- or drug-induced oedemaCitation47. Finally, CA participates in the regulation of the vascular tone by direct (on endothelial and smooth muscle cells) and indirect mechanismsCitation48. CA enzymatic activity inhibition has been related to an enhanced production of the vasodilator molecule nitric oxide (NO)Citation49,Citation50 even if in the cerebral circulation the vasodilating effect of AAZ is independent of NOCitation51. Accordingly, AAZ protected against renal ischemia–reperfusion (I/R) in mice and in human HK-2 cellsCitation52. The increase of renal perfusion rates after I/R insult has been correlated to the vessel dilatation induced by AAZ in both small and medium caliber vesselsCitation53–56. Moreover, AAZ efficiently protected steatotic liver against cold I/R injuryCitation50. Although we observed interesting effects in ischemia after treatment with sulfonamide/coumarin CAIs, our data cannot allow us to elucidate which CA isoforms are involved in the process. This is due on one hand to the fact that many such CAs are present in the brain (CA I, II, VA, VII, IX, XII and XIV among others)Citation42 and on the other one to the rather complex pharmacology of sulfonamides/coumarins, which do not easily arrive to the brain. Thus, this preliminary study opens a new and fascinating field of CAI research, which surely needs to be investigated in deeper detail.
In conclusion, the present data show the properties of a sulfonamide/coumarin CAIs to improve neurological functionalities after cerebral ischemic insult. The theoretical bases of CA involvement in cerebral hypoxic phenomena are thus individuated without however knowing at this point which isoforms are involved in these complex processes.
Acknowledgements
This research was funded by the Italian Ministry of Instruction, University and Research and the University of Florence.
Declaration of interest
The authors declare no conflict of interest.
References
- Thompson BJ, Ronaldson PT. Drug delivery to the ischemic brain. Adv Pharmacol 2014;71:165–202
- Kaur H, Prakash A, Medhi B. Drug therapy in stroke: from preclinical to clinical studies. Pharmacology 2013;92:324–34
- Jahan R, Vinuela F. Treatment of acute ischemic stroke: intravenous and endovascular therapies. Expert Rev Cardiovasc Ther 2009;7:375–87
- Kleindorfer D, Kissela B, Schneider A, et al. Eligibility for recombinant tissue plasminogen activator in acute ischemic stroke: a population-based study. Stroke 2004;35:e27–9
- Wykoff CC, Beasley NJ, Watson PH, et al. Hypoxia-inducible expression of tumor-associated carbonic anhydrases. Cancer Res 2000;60:7075–83
- Stiehl DP, Wirthner R, Köditz J, et al. Increased prolyl 4-hydroxylase domain proteins compensate for decreased oxygen levels. Evidence for an autoregulatory oxygen-sensing system. J Biol Chem 2006;281:23482–91
- Cravotto G, Balliano G, Tagliapietra S, et al. Umbelliferone aminoalkyl derivatives, a new class of squalene-hopene cyclase inhibitors. Eur J Med Chem 2004;39:917–24
- Kosiova I, Kovackova S, Kois P. Synthesis of coumarin–nucleoside conjugates via Huisgen 1,3-dipolar cycloaddition. Tetrahedron 2007;63:312–20
- Kwok S, Fotsing J, Fraser R, et al. Transition-metal-free catalytic synthesis of 1,5-diaryl-1,2,3-triazoles. Org Lett 2010;12:4217–19
- Caddick S, Delisser VM, Doyle VE, et al. Studies toward the synthesis of natural and unnatural dienediynes 1. Approaches to a functionalised bicyclic ring system. Tetrahedron 1999;55:2737–54
- Khalifah RG. The carbon dioxide hydration activity of carbonic anhydrase. I. Stop-flow kinetic studies on the native human isoenzymes B and C. J Biol Chem 1971;246:2561–73
- Liu F, Martin-Mingot A, Lecornué F, et al. Carbonic anhydrases inhibitory effects of new benzenesulfonamides synthesized by using superacid chemistry. J Enzyme Inhib Med Chem 2012;27:886–91
- Ekinci D, Kurbanoglu NI, Salamci E, et al. Carbonic anhydrase inhibitors: inhibition of human and bovine isoenzymes by benzenesulphonamides, cyclitols and phenolic compounds. J Enzyme Inhib Med Chem 2012;27:845–8
- Hilvo M, Baranauskiene L, Salzano A, et al. Biochemical characterization of CA IX, one of the most active carbonic anhydrase isozymes. J Biol Chem 2008;283:27799–809
- Nocentini A, Carta F, Ceruso M, et al. Click-tailed coumarins with potent and selective inhibitory action against the tumor-associated carbonic anhydrases IX and XII. Bioorg Med Chem 2015;23:6955–66
- Casini A, Scozzafava A, Mincione F, et al. Carbonic anhydrase inhibitors: water-soluble 4-sulfamoylphenylthioureas as topical intraocular pressure-lowering agents with long-lasting effects. J Med Chem 2000;43:4884–92
- Kilkenny C, Browne WJ, Cuthill IC, et al. Improving bioscience research reporting: the ARRIVE guidelines for reporting animal research. J Pharmacol Pharmacother 2010;1:94–9
- Longa EZ, Weinstein PR, Carlson S, Cummins R. Reversible middle cerebral artery occlusion without craniectomy in rats. Stroke 1989;20:84–91
- Garcia JH, Wagner S, Liu KF, Hu XJ. Neurological deficit and extent of neuronal necrosis attributable to middle cerebral artery occlusion in rats. Statistical validation. Stroke 1995;26:627–34
- Melani A, Pantoni L, Bordoni F, et al. The selective A2A receptor antagonist SCH 58261 reduces striatal transmitter outflow, turning behavior and ischemic brain damage induced by permanent focal ischemia in the rat. Brain Res 2003;959:243–50
- Bederson JB, Pitts LH, Germano SM, et al. Evaluation of 2,3,5-triphenyltetrazolium chloride as a stain for detection and quantification of experimental cerebral infarction in rats. Stroke 1986;17:1304–8
- Schomacher M, Müller HD, Sommer C, et al. Endocannabinoids mediate neuroprotection after transient focal cerebral ischemia. Brain Res 2008;1240:213–20
- Hara H, Friedlamder RM, Gagliardini V, et al. Inhibition of interleukin 1beta converting enzyme family proteases reduces ischemic and excitotoxic neuronal damage. Proc Natl Acad Sci USA 1997;94:2007–12
- Swanson RA, Sharp FR. Infarct measurement methodology. J Cereb Blood Flow Metab 1994;14:697–8
- Supuran CT, Dedhar S, Carta F, et al. Carbonic anhydrase inhibitors with antimetastatic activity. WO2012070024
- Carta F, Di Cesare Mannelli L, Pinard M, et al. A class of sulfonamide carbonic anhydrase inhibitors with neuropathic pain modulating effects. Bioorg Med Chem 2015;23:1828–40
- Supuran CT. Carbonic anhydrases: from biomedical applications of the inhibitors and activators to biotechnological use for CO(2) capture. J Enzyme Inhib Med Chem 2013;28:229–30
- Supuran CT. Structure-based drug discovery of carbonic anhydrase inhibitors. J Enzyme Inhib Med Chem 2012;27:759–72
- Bonneau A, Maresca A, Winum JY. Metronidazole-coumarin conjugates and 3-cyano-7-hydroxy-coumarin act as isoform-selective carbonicanhydrase inhibitors. J Enzyme Inhib Med Chem 2013;28:397–401
- Maresca A, Temperini C, Vu H, et al. Non-zinc mediated inhibition of carbonic anhydrases: coumarins are a new class of suicide inhibitors. J Am Chem Soc 2009;131:3057–62
- Supuran CT, Ilies MA, Scozzafava A. Carbonic anhydrase inhibitors. Part 29. Interaction of isozymes I, II and IV with benzolamide-like derivatives. Eur J Med Chem 1998;33:739–52
- Supuran CT, Maresca A, Gregáň F, et al. Three new aromatic sulfonamide inhibitors of carbonic anhydrases I, II, IV and XII. J Enzyme Inhib Med Chem 2013;28:289–93
- Scozzafava A, Briganti F, Ilies MA, Supuran CT. Carbonic anhydrase inhibitors: synthesis of membrane-impermeant low molecular weight sulfonamides possessing in vivo selectivity for the membrane-bound versus cytosolic isozymes. J Med Chem 2000;43:292–300
- Matthews TA, Abel A, Demme C, et al. Expression of the CHOP-inducible carbonic anhydrase CAVI-b is required for BDNF-mediated protection from hypoxia. Brain Res 2014;1543:28–37
- Agnati LF, Tinner B, Staines WA, et al. On the cellular localization and distribution of carbonic anhydrase II immunoreactivity in the rat brain. Brain Res 1995;676:10–24
- Tong CK, Brion LP, Suarez C, Chesler M. Interstitial carbonic anhydrase (CA) activity in brain is attributable to membrane-bound CA type IV. J Neurosci 2000;20:8247–53
- Svichar N, Esquenazi S, Waheed A, et al. Functional demonstration of surface carbonic anhydrase IV activity on rat astrocytes. Glia 2006;53:241–7
- Obara M, Szeliga M, Albrecht J. Regulation of pH in the mammalian central nervous system under normal and pathological conditions: facts and hypotheses. Neurochem Int 2008;52:905–19
- Diarra A, Sheldon C, Brett CL, et al. Anoxia-evoked intracellular pH and Ca2+ concentration changes in cultured postnatal rat hippocampal neurons. Neuroscience 1999;93:1003–16
- Sheldon C, Diarra A, Cheng YM, Church J. Sodium influx pathways during and after anoxia in rat hippocampal neurons. J Neurosci 2004;24:11057–69
- Swietach P, Vaughan-Jones RD, Harris AL. Regulation of tumor pH and the role of carbonic anhydrase 9. Cancer Metastasis Rev 2007;26:299–310
- Supuran CT. Carbonic anhydrases: novel therapeutic applications for inhibitors and activators. Nat Rev Drug Discov 2008;7:168–81
- Pettersen EO, Ebbesen P, Gieling RG, et al. Targeting tumour hypoxia to prevent cancer metastasis. From biology, biosensing and technology to drugdevelopment: the METOXIA consortium. J Enzyme Inhib Med Chem 2015;30:689–721
- Klatzo I. Brain oedema following brain ischaemia and the influence of therapy. Br J Anaesth 1985;57:18–22
- Masini E, Carta F, Scozzafava A, Supuran CT. Antiglaucoma carbonic anhydrase inhibitors: a patent review. Expert Opin Ther Pat 2013;23:705–16
- Scozzafava A, Supuran CT. Glaucoma and the applications of carbonic anhydrase inhibitors. Subcell Biochem 2014;75:349–59
- Jacson EK. Diuretics. In: Brunton L, Lazo J, Parker K, eds. Goodman and Giltman’s the pharmacological basis of therapeutics. 9th ed. New York: Pergamon Press Ltd; 1995;690–5
- Swenson ER. New insights into carbonic anhydrase inhibition, vasodilation, and treatment of hypertensive-related diseases. Curr Hypertens Rep 2014;16:467
- Tuettenberg J, Heimann A, Kempski O. Nitric oxide modulates cerebral blood flow stimulation by acetazolamide in the rat cortex: a laser Doppler scanning study. Neurosci Lett 2001;315:65–8
- Bejaoui M, Pantazi E, De Luca V, et al. Acetazolamide protects steatotic liver grafts against cold ischemia reperfusion injury. J Pharmacol Exp Ther 2015;355:191–8
- Kiss B, Dallinger S, Findl O, et al. Acetazolamide-induced cerebral and ocular vasodilation in humans is independent of nitric oxide. Am J Physiol 1999;276:R1661–7
- An Y, Zhang JZ, Han J, et al. Hypoxia-inducible factor-1α dependent pathways mediate the renoprotective role of acetazolamide against renal ischemia-reperfusion injury. Cell Physiol Biochem 2013;32:1151–66
- Taki K, Oogushi K, Hirahara K, et al. Preferential acetazolamide-induced vasodilation based on vessel size and organ: confirmation of peripheral vasodilation with use of colored microspheres. Angiology 2001;52:483–8
- Horita Y, Yakabe K, Tadokoro M, et al. Renal circulatory effects of acetazolamide in patients with essential hypertension. Am J Hypertens 2006;19:282–5
- Teppema LJ, Bijl H, Romberg RR, Dahan A. Antioxidants reverse depression of the hypoxic ventilatory response by acetazolamide in man. J Physiol (Lond.) 2006;572:849–56
- Iida H, Nakagawara J, Hayashida K, et al. Multicenter evaluation of a standardized protocol for rest and acetazolamide cerebral blood flow assessment using a quantitative spect reconstruction program and split-dose 123i-iodoamphetamine. J Nucl Med 2010;51:1624–31