Abstract
In this study, the extracellular thermostable alkaline protease out of A10 strain was purified 1.38-fold with 9.44% efficiency through the ammonium sulfate precipitation-dialysis and DE52 anion exchange chromatography methods. The molecular weight of the enzyme in question along with sodium dodecyl sulfate-polyacrylamide gel electrophoresis was determined to be approximately 40.55 kDa, whereas the optimum pH and temperature ratings were identified as 9.0 and 70 °C, respectively. It was seen that the enzyme had remained stable between pH 7.5–10.5 range, protecting more than 90% of its activity in the wake of 1 h incubation at 60–70 °C. It was also observed that the enzyme enhanced its activity in the presence of Mg2+, Mn2+, K+, while Fe2+, Ni2+, Zn2+, Ag+ and Co2+ decreased the activity. Ca2+, however, did not cause any change in the activity. The enzyme was seen to have been totally inhibited by phenylmethylsulfonyl fluoride, therefore, proved to be a serine alkaline protease.
Introduction
Proteases (EC: 3.4.21–24 and 99), which comprise one of the enzyme groups that has the greatest market share among industrial enzymes, catalyze the hydrolysis of the peptide bonds within proteinsCitation1. Proteases are classified as acidic, alkaline and neutral proteases according to the pH and they exhibit maximum efficacy within a specific pH rangeCitation2.
Alkaline proteases have a high activity and stability at the alkaline pH range, comprising approximately 30% of the total enzyme production throughout the worldCitation3. The alkaline proteases which are significant in terms of industrial potential, notably the detergent industry, are often used in a number of fields, such as leather, textile, food and pharmaceutical industriesCitation3–5. The alkaline proteases, particularly those with 8.5–10.0 pH range, are suitable for the detergent formulationCitation6.
The majority of the commercial alkaline proteases are isolated from the type of bacteria referred to as Bacillus speciesCitation6. These are regarded as conspicuous industrial organisms due to the fact that most of the strains of this species are non-pathogenic, that their isolations are effortless, that they discharge the protein they synthesize to the outer environment and that they are produced in large amounts in a short time through the fermentation methodCitation7,Citation8. Many alkaline proteases have been purified and characterized from Bacillus sp., which are Bacillus licheniformisCitation9–15; B. clausiiCitation16,Citation17; B. pumilusCitation18–20; B. subtilisCitation21; B. laterosporusCitation22; B. mojavensisCitation23 and B. koreensisCitation24. The alkaline proteases obtained from B. licheniformis, in particular, comprise the majority of the detergent marketCitation25.
Since several important chemical processes industrially take place under tough conditions like high temperature and pressure, the microorganisms living under extreme conditions have attracted the attention of researchers. Microbial diversity is an important source for biotechnological products and processesCitation3. It is generally required that the thermostability of the alkaline proteases used commercially be high, which provides an advantage in accordance with the intended purposes. The alkaline proteases produced by thermophilic and alkaliphilic microorganisms are able to endure high temperature and a broad pH rangeCitation21. These characteristics of theirs make them conspicuous strains for the discovery of new alkaline proteases due to their biotechnological potentials.
In this study, the purification and characterization of the alkaline protease enzyme from B. licheniformis A10, a thermophilic bacterium, are aimed at.
Materials and methods
Microorganism
In this study, the production of the alkaline protease from B. licheniformis A10 strain, which was isolated from Erzurum Ilıca hot springs by Yanmis et al.Citation26, was used as the enzyme source.
Enzyme and protein assay
The protease activity was determined through the modification of the method by means of which casein identified by Takami et al.Citation27 was used as a substrate. A 0.5 ml of enzyme solution was mixed with 0.65% of casein solution prepared in 2.5 ml, 100 mm pH 8.5 Tris-HCl buffer solutions, and the reaction solution was incubated at 37 °C for 10 min. Instead of a blank enzyme solution, the reaction mixture into which 100 mm pH 8.5 Tris-HCl buffer solutions had been added was used in the process. At the end of the incubation, 2.5 ml, 110 mm trichloroacetic acid solution was added into the reaction mixtures, after which it was kept at room temperature for 30 min and the reaction was completed. At the deadline, the reaction mixture was centrifuged at 10 000× g for 10 min, and then the 2.5 ml 0.5 M Na2CO3 solution was added into 1 ml of supernatant liquid. Afterwards, 0.5 ml 0.5 M Folin–Ciocalteu reagent was added over the reaction mixture, and after the mixture was kept at room temperature for 30 min, the absorbance was measured spectrophotometrically at 660 nm. One unit of protease activity was expressed as the enzyme amount that formed l μg/min tyrosine from 0.65% of casein in the hydrolysis reaction that took place at pH 8.5 and at 37 °C. The protein concentration was determined according to Bradford method in which bovine serum albumin (BSA) was used as the standardCitation28.
Purification of protease
The cell isolates of B. licheniformis A10 were centrifuged at 10 000× g for 10 min, after which the supernatant fluid was filtered through Whatman I filter paper, and then, the homogenate was obtained. Afterward, the mixture precipitated with the ammonium sulfate at 0–90% range was centrifuged at 5000× g for 15 min. The precipitate obtained was dissolved with 100 mm Tris-HCl (pH = 8.5) sufficiently was dialyzed toward the same buffer. An increasing linear gradient elusion with an ionic strength along with the same buffer containing 50–500 mm NaCl was applied by loading a diethylaminoethyl (DEAE)-cellulose (DE52) column, the enzyme solution of which had been equilibrated with a 50 mm Tris-HCl buffer (pH 8.5). The determinations of protein and activity were performed in the collected fractions.
Sodium dodecyl sulfate-polyacrylamide gel electrophoresis and zymography
After the enzyme was purified, a 3–8% of batch sodium dodecyl sulfate polyacrylamide gel electrophoresis (SDS-PAGE) was performed according to Laemmli methodCitation29, and the purity grade and molecular mass of the enzyme were determinedCitation30. The protein bands were identified through silver staining. For the zymogram of the alkaline protease enzyme, the gel, after the electrophoresis process, was kept within 2.5% of Triton X-100 solution prepared with 100 mm pH 8.5 Tris-HCl in order to remove SDS, after which the washing process was performed three times with Tris-HCl buffer. The gel was incubated within 0.65% casein solution at 37 °C for 30 min, and finally, it was stained with Coomassie Brilliant BlueCitation31. The clear zone formed within the gel suggested the presence of a protease activity.
Enzyme kinetics
The Km and Vmax values of the protease enzyme were determined through the use of Lineweaver–Burk graphic by measuring their activities at different concentrations and at 0.02–0.6% range of the casein substrate. Later on, a quantitative protein determination was performed in the enzyme solution performed to calculate the Km and Vmax values, and the total enzyme amount was identified, and then kcat and the specificity constant (V0) values were calculated through the obtained valuesCitation29,Citation32.
The effect of pH and temperature on the activity of the alkaline protease enzyme
In order to determine the optimum pH, the determination of the activity was performed by using sodium phosphate (pH 6.0–8.0), Tris-HCl (pH 8.0–9.0) and glycine-NaOH (pH 9.0–11.0) buffer solutions with 0.1 M different pH values, which contained 0.65% casein. To ascertain the pH, it was stable at, the enzyme was incubated at the same buffer solution range (pH 6.0–11.0) for 2 h at 37 °C, after which the remaining activity percentage (%) was determined.
The optimum temperature of the enzyme was ascertained by measuring the enzyme activity during the 10-min-incubation period at the temperature range of 20–80 °C. To determine the temperature stability of the enzyme, the activity was measured by leaving the enzyme solution to the incubation process for 15, 30 and 60 min-durations at the temperature range of 20–80 °C. The activity amount prior to the incubation process was accepted as 100, and the values obtained after the incubation were expressed as the remaining activity percentage (%)Citation23,Citation33.
The effect of some inhibitors and metal ions on the activity of the alkaline protease enzyme
The effects of the inhibitors (1 and 5 mm) on the enzyme activity were examined by using phenylmethylsulfonyl fluoride (PMSF), ethylenediaminetetraacetic acid (EDTA), β-mercaptoethanol and dithio-bis-nitrobenzoic acid (DTNB). The enzyme was incubated along with the incubator for 30 min at 37 °C, and the activity amount of the enzyme solution containing no inhibitor was accepted as 100%, and then the remaining enzyme activity was calculated. The effects of various metal ions (1, 5 and 10 mm) on the enzyme activity were investigated by adding univalent (K+, Ag+) and bivalent metal ions (Co2+, Mg2+, Fe2+, Mn2+, Ca2+) into the enzyme-substrate reaction mixture. The activity amount of the enzyme solution containing no metal ion was accepted as 100%, and then the activity of the samples containing metal ion was determined as the remaining activity.
The effect of the organic solvents on the alkaline protease activity
The protease enzyme was incubated for 1 and 24 h by getting mixed with organic solvents, such as methanol, ethanol, chloroform, isopropanol, butanol, acetone, dimethyl sulfoxide (DMSO) and formamide at different concentrations (15%, 25%, and 50%). The activity amount of the enzyme solution containing no organic solvent was accepted as 100%, and then the remaining enzyme activity was calculated.
The effect of surface active agents and oxidant agent on the alkaline protease activity
The effects of some surfactants (SDS, Triton X-100, Tween-20, Tween-80) and the oxidant agent (H2O2) on the enzyme activity were examined by being subjected to the incubation process along with the enzyme for 30 min at 37 °C. The enzyme activity in which there was no additive was received as 100%, and the remaining enzyme activity was calculated.
Substrate specificity – determining the specificity of the alkaline protease enzyme over natural substrates
The substrate specificity of the enzyme was investigated by using the substrates, such as casein, azocasein, hemoglobin, BSA and gelatin. To that end, the determination of the activity was performed by mixing 0.5 ml enzyme solution with 2.5 ml substrate solutions. The activity of the enzyme over the substrate in which it showed the highest activity was accepted as 100%, and then the relative enzyme activities were calculated.
Results and discussion
In this study, an extracellular alkaline protease enzyme taken from B. licheniformis A10, which was isolated from Erzurum Ilıca hot springs by Yanmis et al.Citation26, was purified and characterized.
Purification of B. licheniformis A10 protease
Protease enzyme obtained from B. licheniformis A10 was purified 1.38 times with 9.44% efficiency through ammonium sulfate precipitation (90% w/v) and DE52 anion exchange chromatography. The purification results of the enzyme have been summarized in . The molecular weight of the enzyme was determined as almost 40.55 kDa through SDS-PAGE (). In the former studies, alkaline proteases were obtained from the types of Bacilli with different molecular weights, such as B. licheniformis RSP-09-37 (55 kDa)Citation34, Bacillus sp. SM2014 (71 kDa)Citation35, B. cereus MCM B-326 (45 kDa)Citation36, B. subtilis DM-04 (16.9 kDa)Citation21, B. licheniformis (32 kDa)Citation37, Bacillus sp. RKY3 (38 kDa)Citation38, B. strain HUTBS71 (49 kDa)Citation39.The activity band of the protease enzyme was observed through the zymogram analysis in the form of a clear casein hydrolysis zone within the gel, which verified the fact that the purified enzyme was the protease ().
Figure 1. SDS-PAGE of the purified protease from B. licheniformis A10. Lane 1: Bio-Rad Precision Plus molecular weight markers (Bio-Rad, Hercules, CA); lane 2: purified protease, lane 3: zymography of the purified protease.
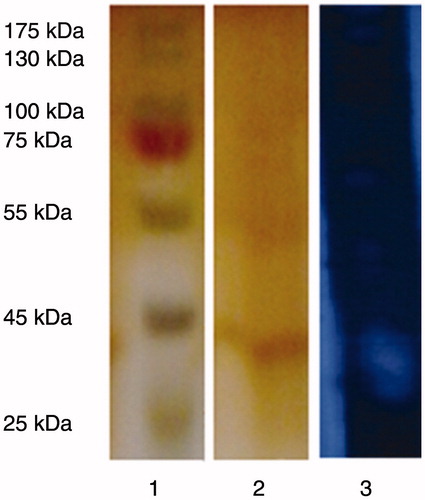
Table 1. Summary of the alkaline protease from B. licheniformis A10 purification procedure.
Kinetic parameters
The Km and Vmax values of the protease enzyme purified from B. licheniformis A10 were determined as 0.033 mg/ml and 8.17 μmol. ml−1.min−1, respectively, for the casein substrate. By using the Km and Vmax values, kcat and V0 values were calculated as 1.392 s−1 and 42.18 μM−1.s−1, respectively. It was determined that Km value of B. licheniformis A10 protease for the casein substrate proved to be lower when compared with other Bacillus sp.Citation15,Citation32,Citation35 alkaline proteases, and as a result, the enzyme was found to have had a high affinity for the casein.
The effect of pH and temperature on protease activity and stability
The optimum pH of the enzyme isolated from B. licheniformis A10 was determined as 9.0, and this outcome suggests that the enzyme could have been an alkaline protease. These findings show concordance with the proteases, such as B. pumilus TMS55Citation30, B. horikoshiiCitation16, B. licheniformis YP1ACitation40, B. subtilis DM-04Citation21 and Bacillus sp. KSM-K16Citation22, the formerly reported optimum pH of which was 9.0–9.5. In general, significant commercial proteases obtained from microorganisms have the highest activity at 8.0–12.0 alkaline pH rangeCitation41. The results obtained from this study also showed that the enzyme in question had the highest activity at pH 7.0–10.0 range. When the pH stability profile of the enzyme is analyzed, it was seen that it had maintained more than 70% of its activity in the wake of the 2-h incubation at pH 7.5–9.5 range. However, it was observed that this rate dropped down to 45% and 62% at pH 6.0 and 7.0, whereas at pH 10.0–10.5 range, it dropped down to 55% on the average and to 28.97% at pH 11.0 (). The protease enzyme purified from B. licheniformis A10 was determined to have showed stability at pH 7.5–10.5 range and there were also alkaline proteases reported to have been produced from Bacillus sp. with a similar pH stability rangeCitation14,Citation39,Citation42,Citation43. The pH stability is an important factor, particularly, for industrial practicesCitation38,Citation41.
Figure 2. Effect of pH on activity (a) and stability (b) of the purified protease from B. licheniformis A10.
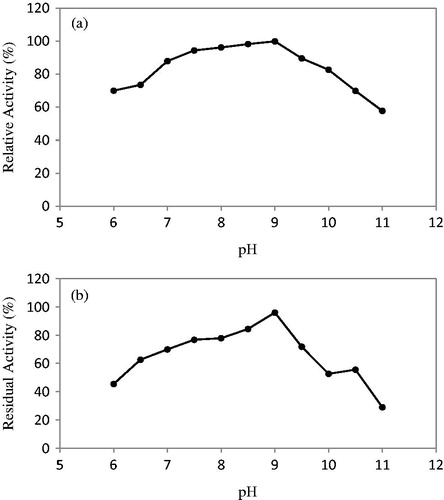
When the temperature profile of the protease enzyme obtained from B. licheniformis A10 was examined, the optimum temperature value was determined to be 70 °C. The enzyme was ascertained to have had quite high activity through the range of 50–80 °C (). It is also seen that the protease produced by B. licheniformis A10 has a higher optimum temperature value than those reported formerly. For instance, the proteases with the optimum temperature of 65 °C are Bacillus HS08Citation44, B. licheniformis 3C5Citation37, Bacillus strain HUTBS71Citation39; whereas those with 60 °C-optimum temperature are B. licheniformis ATCC 21424Citation12, B. licheniformis AP-1Citation43, Bacillus sp. HR-08Citation45, B. mojavensis A21Citation23 and Bacillus sp. SM2014Citation35; and the protease with 50 °C-optimum temperature is B. licheniformis P0031Citation4. Despite these, there were also other proteases reported, such as B. laterosporus-AK1 and B. cereus and B. stearothermophilus, the optimum temperatures of which were 70–75 °CCitation22,Citation46. The proteases active at high temperature and at alkaline pH have great advantages in terms of their use in detergent formulations and in other biotechnological practicesCitation21. When compared with the data in the literature; the fact that the protease enzyme obtained from B. licheniformis A10 has quite a high optimum temperature values suggests that the applicability of this enzyme in the detergent industry, in particular, is likely to be quite high. When the temperature stability profile of the enzyme was examined, it was seen that it preserved more than 90% of its activity at 60 °C and 70 °C, and when compared with the other temperature ratings, it was seen to have remained stable for a long time. It was determined that it preserved more than 50% of its activity at the temperature range of 20–80 °C at the end of 15- and 30-min-incubation periods; whereas, at the end of a 60-min-incubation, it preserved 70.74%, 54.22%, 94.82% and 98.47% of its activity at 40 °C, 50 °C, 60 °C and 70 °C, respectively (). Sarker et al.Citation14 showed that B. licheniformis P003 protease enzyme gained 87% and 70% residual activity, respectively, in the wake of a 60-min-incubation at 50 °C and 60 °C. The protease enzyme from B. licheniformis MH31 was reported to have remained stable by 75% at the end of 60 min at 60 °CCitation47. It was reported that half of the activity of B. licheniformis AP-1 protease had remained at the end of 2-h incubation at 60 °C and a 15-min-incubation at 65 °CCitation43. It was also stated by Rachadech et al.Citation37 that more than 75% of the activity of B. licheniformis 3C5 protease had remained at the end of 12 and 24 h incubations at 30–45 °C, whereas its activity decreased at higher temperatures and even became inactive at 80 °C.
The effect of some inhibitors and metal ions on the alkaline protease enzyme activity
When the effects of different enzyme inhibitors on the protease enzyme purified from B. licheniformis A10 was examined, it was seen that the enzyme had been totally inhibited in the presence of PMSF, whereas in the presence of EDTA and urea, it was inhibited strongly. Separately, a weak inhibition was identified in the presence of DTNB. In the presence of β-mercaptoethanol, on the other hand, an increase in the enzyme activity was observed (). The fact that it becomes totally inhibited by the serine-specific inhibitor, PMSF and the increase in its activity in the presence of β-mercaptoethanol suggests that the enzyme in question can be classified as a thiol-dependent serine proteaseCitation45,Citation48. The majority of the alkaline proteases of Bacillus origin have been reported to belong to serine protease classCitation49. Separately, the fact that it was also strongly inhibited in the presence of a metal chelator, EDTA, brought about the result that the enzyme had a metal binding regionCitation37,Citation50.
Table 2. The effect of inhibitors on B. licheniformis A10 alkaline protease activity.
The effects of various metal ions on the protease enzyme purified from B. licheniformis A10 at 1, 5 and 10 mm-concentrations were examined, and the results were given in . Although it was reported that most of Bacillus sp. proteases were induced by Ca2+ ionCitation23, it was determined that Ca2+ metal did not perform any change in B. licheniformis A10 protease enzyme activity. It was ascertained that K+, Mn2+ and Mg2+ activated the enzyme, while Fe2+, Ni2+, Zn2+, Ag+ and Co2+ metals inhibited it rather weakly. Similarly, Sarker et al.Citation14, in a study they conducted, saw that the activity of the alkaline protease enzyme isolated from B. licheniformis P003 was enhanced by Mn2+ and Mg2+ metals, while being reduced by Fe2+ metal; yet, Ca2+, on the other hand, did not perform any significant change in the enzyme activity. While B. cereus 1173900 protease activity was also inhibited by Zn2+, Cu2+ and Fe2+, Ca2+, Mg2+ and Mn2+ were reported to have increased the activity, as wellCitation33. It was reported that the divalent cations like Ca2+, Mn2+ and Mg2+ increased the thermal stability of Bacillus alkaline proteasesCitation45. Metal ions play a vital role in sustaining the active conformations of the enzymes at high temperatures by also protecting them against thermal inactivationCitation41.
Table 3. The effect of metal ions on B. licheniformis A10 alkaline protease activity.
The effect of organic solvents on the alkaline protease activity
When the stability of the protease enzyme purified from B. licheniformis A10 in the presence of organic solvents is analyzed, it was observed that the enzyme had preserved its activity by more than 50% as the result of the 1 h incubation at 15% and 25% – concentrations of ethanol, methanol, butanol, isopropanol, chloroform and DMSO, and at 50% concentration of butanol, isopropanol, chloroform and DMSO. In addition, in the presence of formaldehyde, the enzyme was determined to have increased its activity. On the other hand, as the result of the 24 h incubation, the enzyme was determined to have preserved its activity by more than 50% in the presence of butanol (15%), isopropanol (25–50%), formaldehyde (15–25–50%) and DMSO (15–25%) (). As seen from the results, as well, while the protease purified from B. licheniformis A10 showed stability against organic solvents as the result of 1 h incubation, it showed lower stability as the result of 24 h incubation. Enzymes easily lose their activities in the presence of organic solvents. In the literature, the proteases inactive in the presence of organic solvents, such as benzene, toluene, xylene, ethanol, acetone and DMSO, are reported, as well. Besides, the proteases stable in the presence of organic solvents are rarely found, and these enzymes have fields of use for synthetic reactions in the industryCitation51. It was observed that the protease purified from B. licheniformis A10 had showed better stability in the presence of DMSO when compared with the protease purified from B. licheniformis NCIM2042; yet, it exhibited lower stability in the presence of ethanol, acetone and butanolCitation13. Rachadech et al.Citation37 reported that B. licheniformis 3C5 protease had preserved more than 80% of its initial activity after 1 h incubation in the presence of various organic solvents; yet, its activity decreased following the next 6 h.
Table 4. The effect of organic solvents on B. licheniformis A10 alkaline protease activity.
The effect of surface active agents and the oxidant agent on the alkaline protease activity
The effect of surface active agents and the oxidant agent on the protease enzyme purified from B. licheniformis A10 has been summarized in . While it was determined that the enzyme had preserved 61% and 44.38% of its activity, respectively, in the presence of 1% and 5% of Triton X-100, it was seen that the enzyme had been strongly inhibited in the presence of SDS. On the other hand, it was observed that the enzyme activity had decreased in the presence of Tween-20, Tween-80 and H2O2. Similarly, it was reported in the former studies that Bacillus sp. protease activity was inhibited by SDSCitation14,Citation23 and H2O2Citation23,Citation35; yet, it preserved its activity in the presence of Triton X-100Citation13,Citation23,Citation52.
Table 5. The effect of surface active agents and the oxidant agent on B. licheniformis A10 alkaline protease activity.
Substrate specificity
When the effect of some of the natural substrates on the protease purified from B. licheniformis A10 was examined, it was ascertained that the enzyme had showed its highest activity in the presence of casein (100%) and azocasein (97.36%). It was also determined that the enzyme in question, when gelatin, hemoglobin and BSA substrates were used, had relative activities at the rate of 60.4%, 28.73% and 17.74%, respectively (). Similarly, also in the former studies, the alkaline protease was reported to have shown the highest activity toward caseinCitation12,Citation22,Citation42.
Table 6. The substrate specificity of B. licheniformis A10 alkaline protease.
Conclusion
In this study, the alkaline protease enzyme produced by B. licheniformis A10 was characterized and purified. The protease activity was inhibited by PMSF and EDTA, known as serine-metalloproteinase inhibitors. It can be considered that B. licheniformis A10 protease enzyme exhibiting high activity and stability at high temperatures and at pH will likely to have a potential of use with such characteristics in various industrial practices, notably the detergent industry.
Declaration of interest
This work was funded by grants from the Scientific Research Project of Ataturk University of Turkey (project number: 2013/297). The authors declared that there is no conflict of interests.
References
- Pihlanto A. Antioxidative peptides derived from milk proteins. Int Dairy J 2006;16:1306–14.
- Rao MB, Tanksale AM, Ghatge MS, Deshpande VV. Molecular and biotechnological aspects of microbial proteases. Microbiol Mol Biol Rev 1998;62:597–635.
- Gupta R, Beg QK, Lorenz P. Bacterial alkaline proteases: molecular approaches and industrial applications. Appl Microbiol Biotechnol 2002;59:15–32.
- Chandel AK, Rudravaram R, Rao LV, et al. Industrial enzymes in bioindustrial sector development: an Indian perspective. J Commerc Biotechnol 2007;13:283–91.
- Asokon S, Jayanthi C. Alkaline protease production by Bacillus licheniformis and Bacillus coagulans. J Cell Tissue Res 2010;10:2119–23.
- Maurer KH. Detergent proteases. Curr Opin Biotechnol 2004;15:330–4.
- Divakar K, Priya JDA, Gautam P. Purification and characterization of thermostable organic solvent-stable protease from Aeromonas veronii PG01. J Mol Catal B Enzym 2010;66:311–18.
- Boyce A, Walsh G. Production, purification and application-relevant characterisation of an endo-1,3(4)-beta-glucanase from Rhizomucor miehei. Appl Microbiol Biotechnol 2007;76:835–41.
- Mabrouk SS, Hashem AM, El-Shayeb NMA, et al. Optimization of alkaline productivity by Bacillus licheniformis ATCC 21415. Bioresour Technol 1999;69:155–9.
- Olajuyigbe FM, Ajel JO. Some properties of extracellular protease from Bacillus licheniformis Lbbl-11 isolated from “iru”, a traditionally fermented African locust bean condiment. Global J Biotechnol Biochem 2008;3:42–6.
- Toyokawa Y, Takahara H, Reungsang A, et al. Purification and characterization of a halotolerant serine proteinase from thermotolerant Bacillus licheniformis RKK-04 isolated from Thai fish sauce. Appl Microbiol Biotechnol 2010;86:1867–75.
- Bezawada J, Yan S, John RP, et al. Recovery of Bacillus licheniformis alkaline protease from supernatant of fermented wastewater sludge using ultrafiltration and its characterization. Biotechnol Res Int 2011;2011:238549.
- Bhunia B, Dey A. Statistical approach for optimization of physiochemical requirements on alkaline protease production from Bacillus licheniformis NCIM 2042. Enzyme Res 2012;2012:905804. doi:10.1155/2012/905804.
- Sarker PK, Talukdar SA, Deb P, et al. Optimization and partial characterization of culture conditions for the production of alkaline protease from Bacillus licheniformis P003. SpringerPlus 2013;2:506. doi:10.1186/2193-1801-2-506.
- Nadeem M, Qazi JI, Syed Q, Gulsher M. Purification and characterization of an alkaline protease from Bacillus licheniformis UV-9 for detergent formulations. Songklanakarin J Sci Technol 2013;35:187–95.
- Joo HS, Kumar CG, Park GC, et al. Optimization of the production of an extracellular alkaline protease from Bacillus horikoshi. Process Biochem 2002;38:155–9.
- Joo HS, Chang CS. Oxidant and SDS-stable alkaline protease from a halo-tolerant Bacillus clausii I-52: enhanced production and simple purification. J Appl Microbiol 2004;98:491–7.
- Jaouadi B, Ellouz-Chaabouni S, Rhimi M, Bejar S. Biochemical and molecular characterization of a detergent-stable serine alkaline protease from Bacillus pumilus CBS with high catalytic efficiency. Biochimie 2008;90:1291–305.
- Ibrahim KS, Muniyandi J, Karutha Pandian S. Purification and characterization of manganese-dependent alkaline serine protease from Bacillus pumilus TMS55. J Microbiol Biotechnol 2011;1:20–7.
- Jayakumar R, Jayashree S, Annapurna B, Seshadri S. Characterization of thermostable serine alkaline protease from an alkaliphilic strain Bacillus pumilus MCAS8 and its applications. Appl Biochem Biotechnol 2012;168:1849–66.
- Rai SK, Mukherjee AK. Statistical optimization of production, purification and industrial application of a laundry detergent and organic solvent-stable subtilisin-like serine protease (Alzwiprase) from Bacillus subtilis DM-04. Biochem Eng J 2010;48:173–80.
- Arulmani M, Aparanjini K, Vasanthi K, et al. Purification and partial characterization of serine protease from thermostable alkalophilic Bacillus laterosporus-AK1. World J Microbiol Biotechnol 2007;23:475–81.
- Haddar A, Bougatef A, Agrebi R, et al. A novel surfactant-stable alkaline serine-protease from a newly isolated Bacillus mojavensis A21. Purification and characterization. Process Biochem 2009;44:29–35.
- Anbu P. Characterization of solvent stable extracellular protease from Bacillus koreensis (BK-P21A). Int J Biol Macromol 2013;56:162–8.
- Godfrey T, West S, eds. Introduction to industrial enzymology. In: Industrial enzymology. 2nd ed. New-York: Stockton Press; 1996:1–8.
- Yanmis D, Baltaci MO, Gulluce M, Adiguzel A. Identification of thermophilic strains from geothermal areas in Turkey by using conventional and molecular techniques. Res J Biotechnol 2015;10:39–45.
- Takami H, Akiba T, Horikosh K. Production of extremely thermostable alkaline protease from Bacillus sp. AH-101. Appl Microbiol Biotechnol 1989;30:120–4.
- Bradford MM. A rapid and sensitive method for the quantitation of microgram quantities of protein utilizing the principle of protein-dye binding. Anal Biochem 1976;72:248–51.
- Laemmli UK. Cleavage of structural proteins during the assembly of the head of bacteriophage T4. Nature 1970;227:680–5.
- Güllçin I, Küfrevioğlu OI, Oktay M. Purification and characterization of polyphenol oxidase from nettle (Urtica dioica L.) and inhibitory effects of some chemicals on enzyme activity. J Enzyme Inhib Med Chem 2005;20:297–302.
- Kazan D, Denizci AA, Oner MNK, Erarslan A. Purification and characterization of a serine alkaline protease from Bacillus clausii GMBAE 42. J Ind Microbiol Biotechnol 2005;32:335–44.
- Rao S, Sathish T, Ravichandra P, Prakasham R. Characterization of thermo- and detergent stable serine protease from isolated Bacillus circulans and evaluation of eco-friendly applications. Process Biochem 2009;44:262–8.
- Ravindran B, Ganesh Kumar A, Aruna Bhavani PS, Sekaran G. Solid-state fermentation for the production of alkaline protease by Bacillus cereus 1173900 using proteinaceous tannery solid waste. Curr Sci 2011;100:726–30.
- Sareen R, Mishra P. Purification and characterization of organic solvent stable protease from Bacillus licheniformis RSP-09-37. Appl Microbiol Biotechnol 2008;79:399–405.
- Jain D, Pancha I, Mishra SK, et al. Purification and characterization of haloalkaline thermoactive, solvent stable and SDS-induced protease from Bacillus sp.: a potential additive for laundry detergents. Bioresour Technol 2012;115:228–36.
- Zambare VP, Nilegaonkar SS, Kanekar PP. Production of an alkaline protease by Bacillus cereus MCM B-326 and its application as a dehairing agent. World J Microbiol Biotechnol 2007;23:1569–74.
- Rachadech W, Navacharoen A, Ruangsit W, et al. An organic solvent-, detergent-, and thermostable alkaline protease from the mesophilic, organic solvent-tolerant Bacillus licheniformis 3C5. Microbiology 2010;79:620–9.
- Reddy LVA, Wee YJ, Ryu HW. Purification and characterization of an organic solvent and detergent-tolerant novel protease produced by Bacillus sp. RKY3. J Chem Technol Biotechnol 2008;83:1526–33.
- Akel H, Al-Quadan F, Yousef TK. Characterization of a purified thermostable protease from hyperthermophilic Bacillus strain HUTBS71. Eur J Sci Res 2009;31:280–8.
- Li S, He B, Bai Z, Ouyang P. A novel organic solvent-stable alkaline protease from organic solvent-tolerant Bacillus licheniformis YP1A. J Mol Catal B Enzym 2009;56:85–8.
- Kumar CG, Takagi H. Microbial alkaline proteases: from a bioindustrial viewpoint. Biotechnol Adv 1999;17:561–94.
- Adinarayana K, Ellaiah P, Prasad DS. Purification and partial characterization of thermostable serine alkaline protease from a newly isolated Bacillus subtilis PE-11. AAPS PharmSciTech 2003;4:440–8.
- Tang XM, Lakay FM, Shen W, et al. Purification and characterisation of an alkaline protease used in tannery industry from Bacillus licheniformis. Biotechnol Lett 2004;26:1421–4.
- Guangrong H, Tiejing Y, Po H, Jiaxing J. Purification and characterization of a protease from thermophilic Bacillus strain HS08. Afr J Biotechnol 2006;5:2433–8.
- Moradian F, Khajeh K, Naderi-Manesh H, Sadeghizadeh M. Isolation, purification and characterization of a surfactants-, laundry detergents- and organic solvents-resistant alkaline protease from Bacillus sp. HR-08. Appl Biochem Biotechnol 2009;159:33–45.
- Rahman RNZ, Razak CN, Ampon K, et al. Purification and characterization of a heat-stable alkaline protease from Bacillus stearothermophilus F1. Appl Microbiol Biotechnol 1994;40:822–7.
- Yu J, Jin H, Choi W, Yoon M. Production and characterization of an alkaline protease from Bacillus licheniformis MH31. Agric Chem Biotechnol 2006;49:135–9.
- Beg QK, Gupta R. Purification and characterization of an oxidant stable, thiol dependent serine alkaline protease from Bacillus mojavensis. Enzyme Microb Technol 2003;32:294–304.
- Gessesse A. The use of nug meal as a low-cost substrate for the production of alkaline protease by the alkaliphilic Bacillus sp. AR-009 and some properties of the enzyme. Bioresour Technol 1997;62:59–61.
- Yao D, Qu J, Chang P, et al. Production and characterization of alkaline protease from hemoglobin-degrading Bacillus pumilus NJM4 to produce fermented blood meal. J Food Sci Technol 2012;49:626–31.
- Rahman RNZA, Geok LP, Basri M, Salleh AB. An organic solvent-stable alkaline protease from Pseudomonas aeruginosa strain K: enzyme purification and characterization. Enzyme Microb Technol 2006;39:1484–91.
- Sellami-Kamoun A, Haddar A, Ali Nel H, et al. Stability of thermostable alkaline protease from Bacillus licheniformis RP1 in commercial solid laundry detergent formulations. Microbiol Res 2008;163:299–306.