Abstract
Melatonin (MLT) is a well-known free-radical scavenger, involving in the prevention of cellular damage that can lead to cancer, ageing and a variety of neurodegenerative diseases. Research on MLT-related compounds has been required to optimise the maximum pharmaceutical activity with the lowest side effects. In our ongoing research, we have synthesized new indole-based MLT analogues as potential antioxidant agents by modifying the MLT molecule. In this study, we build on previous findings, through the synthesis, characterization and in vitro antioxidant profiling of a series of new indole-based MLT analogues which possess triazole, thiadiazole and carbothioamides on the third position on the indole ring. In vitro antioxidant activity was investigated by evaluating their reducing effect against oxidation of a redox sensitive fluorescent probe and their radical scavenging activity was assessed via the DPPH assay. In addition, in vitro cytotoxic effects of newly synthesized compounds were investigated in CHO-K1 cells using the MTT assay.
Introduction
Reactive oxygen species (ROS), and reactive nitrogen species (RNS), are products of cellular metabolism, and are well known for having both a harmful and beneficial roleCitation1,Citation2. Overproduction of reactive species causes oxidative stressCitation3 which may lead to serious damage to vital cell structures like proteins and DNACitation4,Citation5. Oxidative stress has been associated with various diseases such as cancerCitation6, heart diseasesCitation7, neurological disorders such as Alzheimer’s diseaseCitation8, Huntington’s diseaseCitation9,Citation10 and ageingCitation11. Melatonin (MLT) is a hormone primarily secreted by the pineal gland along with many other parts of the human bodyCitation11–13 and has a significant anti-oxidant propertyCitation14. MLT () and its metabolites are able to function as endogenous free-radical scavengers and broad-spectrum antioxidantsCitation15,Citation16. In addition, MLT and its derivatives have been shown to exhibit the regulation of circadian rhythm and immune functions in many physiological processes and therapeutic functionsCitation17,Citation18. In recent years, the many physiological properties of MLT have stimulated much interest in the development of synthetic compounds possessing the indole ring. In addition MLT is a highly lipophilic compound that easily passes through cell membranes to reach intracellular compartments, especially mitochondria which are well-known for having high concentrations of ROS. Also MLT acts in the fixation of oxidant-antioxidant balance in mitochondriaCitation19,Citation20. However, MLT has very short half-life in the human body, of approximately 1 h in blood following oral administration. Therefore, the synthesis of MLT analogues with longer half-lives is an interesting topic for researchersCitation20,Citation21.
Recent research has shown that compounds with an indole ring such as indol-3-propionic acidCitation22, indole amine triazoleCitation23 and indolehydrazide/hydrazone derivativesCitation24,Citation25 have significant antioxidant effects. The indole ring of these compounds is the reactive centre dealing with oxidants due to its high resonance stability and low activation energy barrier towards free radical reactionsCitation24,Citation26. In addition to these compounds, there are some studies that demonstrate the antioxidant effect of 1,2,4-triazoles and 1,3,4-thiadiazolesCitation27–29. The triazole molecule is one of the most significant and well recognized heterocycles which is a common feature of a selection of natural products as well as pharmaceutical agents. Triazoles and their derivatives were investigated in many studies and established to be associated with a variety of biological activities like antibacterialCitation30, antifungalsCitation31, antitumor, anti-inflammatory, analgesicCitation32 and antioxidantCitation33. In addition this 1,3,4-thiadiazoles have also been found to be key group of molecules with biological activity particularly antibacterial and antioxidantCitation34. The broad spectrum activities of 1,2,4-triazoles derivatives have attracted the attention of researchers in recent years. Until now, studies showed that modifications of the triazole derivatives have proven highly efficient with better potency and lesser toxicity. Based on the above findings it was planed and synthesized some indole derivatives with 1,2,4-triazole and 1,3,4-thiadiazole with different side chains to improve biological activity of indole derivatives.
In the present study, some modifications were made on the MLT molecule to improve its antioxidant effect. The acetylaminoethyl side chain in the third position of the indole ring was removed and substituted with hydrazinecarbothioamide, triazole and thiadiazole groups. As a part of our ongoing study 31 new compounds were synthesized using four different routes. Synthesis of 1a–h and 3a–h compounds demonstrated high yield with average of 87%. However, synthesis of 4a–g and 2a–h compounds showed low yield, respectively, with 28% and 37%. Biological activity of new synthesis compounds was investigated in vitro. The MTT assay was performed to evaluate their cytotoxic profiles, the DCFH-DA (2′,7′-dichlorodihydrofluorescein diacetate) assay was carried out for revealing the antioxidant activity of the newly synthesized compounds, and the DPPH (2,2-diphenyl-1-picrylhydrazyl) free radical scavenging activity test. All the synthesized compounds except those formerly synthesized (2dCitation35, 2hCitation36, 3cCitation36, 3dCitation36) were characterized on the basis of 1H and 13C NMR, mass spectra and elemental analysis.
Materials and methods
The present study aimed to synthesize, characterize and investigate the potential antioxidant and cytotoxic effects of indole-based MLT analogues containing hydrazinecarbothioamide, triazole and thiadiazoles as side chains attached to the 3-position of the indole ring. Two parts of the MLT molecule were modified to develop new indole-based MLT analogue compounds as shown in . These modifications were done mainly on the MLT acylamino group (.
These modulations of the lead structures were made at two different points: the methoxy group was replaced with hydrogen at the 5-position of the indole ring as modification 1 and the acetylaminoethyl side chain was replaced by hydrazinecarbothioamide (1a–h), thiadiazole (2a–h) and triazole (3a–h) groups as modification 2. Finally, S-alkylation on the compounds (3a–h) provided analogues (4a–g) (). These modifications resulted in a new series of compounds having different heterocyclic groups as side chain in the indole nucleus. These modifications helped to investigate the effect of substituents with different electronic and lipophilic properties of heterocyclic groups on the antioxidant activity and biological half-life of new indole derivatives. Synthetic routes to new MLT analogues are outlined in Scheme 1. Reaction of indole 3-methylhydrazine with isothiocyanates in ethanol under reflux gave the corresponding hydrazinecarbothioamides (1a–h) in high yields. Treatment of 1a–h under strongly acidic conditions proceeded with full regiochemical control to gives the corresponding 2-aminothiadiazoles (2a–h), albeit in low to moderate yields. Conversely, treatment of 1a–h under basic conditions (aq. NaOH) with heating produced the 2-thiotriazoles (3a–h) in high yields with full control of cyclization regiochemistry. Triazoles (3a–h) could be further alkylated under basic conditions to produce substituted triazole 4a–g in low to moderate yield.
Chemistry – experimental
Uncorrected melting points were determined with a Büchi melting point B-540 apparatus (Buchi Labortechnik, Flawil, Switzerland). The 1H and 13C NMR spectra were measured with a Varian 400 MHz instrument (Palo Alto, CA) using TMS as an internal standard and DMSO-d6 as solvent. ESI mass spectra were determined on a Waters Micromass ZQ. Elemental analyses were performed using a CHNS-932 instrument (Leco Corporation, St. Joseph, MI). All spectral analysis was performed at Central Laboratory of the Faculty of Pharmacy, Ankara University. Chromatography was carried out using Merck silica gel 60 (230–400 mesh ASTM). The chemical reagents used in synthesis were purchased from Sigma (Germany) and Aldrich (USA).
General procedure for the synthesis of hydrazinecarbothioamide compounds 1a–h
Equal amounts of 2-(1H-indol-3-yl) acetohydrazide (5 mmol) and aryl or alkylisothiocyanates (5 mmol) were dissolved in absolute ethanol (20 ml). The mixture was heated under reflux for 4–5 h at 80–85 °C. After completion of the reaction, the reaction mixture was concentrated on the rotary evaporator under reduced pressure and kept overnight at room temperature. The crystals thus obtained were purified by washing with petroleum etherCitation35.
2-(2-(1H-Indol-3-yl)acetyl)-N-ethylhydrazincarbothioamide (1a). Yield 95%, m.p. 132 °C; 1H NMR: δ 1.01 (t, 3H, J = 7.6 Hz, CH3); 3.42 (q2H, CH2); 3.55 (s, 2H, Ar–CH2); 6.96 (m, 1H, H-5); 7.05 (m, 1H, H-6); 7.21 (d, 1H, J = 2.4 Hz, H-2); 7.32 (d, 1H, J = 8 Hz, H-7); 7.55 (d, 1H, J = 7.6 Hz, H-4); 7.78, 9.16, 9.84, 10.88 (s, 4H, NH–NH–CS–NH and NH indole); 13C NMR: δ 14.87, 31.07, 38.85, 108.44, 110.00, 111.73, 118.76, 119.19, 121.42, 124.38, 127.65, 136.46, 170.84 (C=O); ESI MS m/z 277 (M + H, 100%); Anal. calcd. for C13H16N4OS: C, 55.59%; H, 5.92%; N, 19.95%. Found: C, 55.21%; H, 6.24%; N, 19.66%.
2-(2-(1H-Indol-3-yl)acetyl)-N-propylhydrazincarbothioamide (1b). Yield 86%, m.p. 143.5 °C; 1H NMR: δ 0.80 (t, 3H, J = 7.2 Hz, CH3); 1.46 (m, 2H, CH2); 3.37 (t, 2H, J = 7.6 Hz, NH–CH2); 3.57 (s, 2H, COCH2); 6.98 (t, 1H, J = 7.6 Hz, H-5); 7.07 (t, 1H, J = 8.4 Hz, H-6); 7.23 (d, 1H, J = 2.4 Hz, H-2); 7.34 (d, 1H, J = 8 Hz, H-7); 7.57 (d, 1H, J = 7.6 Hz, H-4); 7.75; 9.18; 9.87; 10.90; (s, 4H, NH-NH-CS-NH and NH-indole); 13C NMR: δ 11.60, 22.40, 31.10 45.72 (NH–C); 108.46, 111.75, 118.77, 119.19, 121.44, 124.40, 127.65, 136.49, 170.86 (C=O), 175.28 (C=S); ESI MS m/z 291 (M + H, 100%); Anal. calcd. for C14H18N4OS: C, 57.91%; H, 6.24%; N, 19.29%. Found: C, 58.19%; H, 6.75%; N, 19.14%.
2-(2-(1H-Indol-3-yl)acetyl)-N-benzylhydrazincarbothioamide (1c). Yield 78%, m.p. 142.5 °C; 1H NMR: δ 3.58 (s, 2H, COCH2); 4.74 (d, 2H, J = 5.6 Hz, benzyl-CH2); 6.96 (m, 1H, H-5); 7.06 (m, 1H, H-6); 7.23 (d, 1H, J = 2.4 Hz, H-2); 7.21–7.35 (m, 6H, Ar–H); 7.56 (d, 1H, J = 8 Hz, H-4); 8.44, 9.35, 9.94, 10.88 (s, 4H, NH–NH–CS–NH and NH-indole); 13C NMR: δ 30.50; 46.54 (NH-C); 107.84; 111.15; 118.17; 118.68; 120.84; 123.80; 126.50; 126.88, 127.15, 127.94, 135.91, 139.20, 170.36 (C=O) ESI MS m/z 339 (M + H, 100%); Anal. calcd. for C18H18N4OS: C, 62.55%; H, 5.49; N, 16.21%. Found: C, 63.07%; H, 6.01%; N, 16.68%.
2-(2-(1H-Indol-3-yl)acetyl)-N-phenylhydrazincarbothioamide (1d). Yield 90%, m.p. 178.5 °C; 1H NMR: δ 3.63 (s, 2H, COCH2); 6.98 (m, 1H, H-5); 7.07 (m, 1H, H-6); 7.26 (d, 1H, J = 2.4 Hz, H-2); 7.60 (d, 1H, J = 7.6, H-4); 7.14–7.41 (m, 6H, Ar–H); 9.54, 9.64, 10.11, 10.91 (s, 4H, NH–NH–CS–NH and NH indole); 13C NMR: δ 31.17, 108.45, 110.00, 111.75, 118.80, 119.27, 121.44, 124.45, 125.44, 126.04, 127.71, 128.55, 136.50, 139.57, 167.71, 171.01 (C=O), 181.33 (S=C); ESI MS m/z 325 (M + H, 100%); Anal. calcd. for C17H16N4OS: C, 62.94%; H, 4.97%; N, 17.27%. Found: C, 63.05%; H, 5.33%; N, 17.05%.
2-(2-(1H-Indol-3-yl)acetyl)-N-(2,4-difluorophenyl)hydrazincarbothioamide (1e). Yield 81%, m.p. 169 °C; 1H NMR: δ 3.64 (s, 2H, Ar–CH2); 6.98–7.61 (m, 8H, Ar–H); 9.60 (s, 1H, NH indole); ESI MS m/z 361 (M + H, 100%); Anal. calcd. for C17H14F2N4OS: C, 56.66%, H, 3.92%, N, 15.55%. Found: C, 56.68%, H, 4.14%, N, 15.66%.
2-(2-(1H-Indol-3-yl)acetyl)-N-(3-fluorophenyl)hydrazincarbothioamide (1f). Yield 92%, m.p. 183.5 °C; 1H NMR: δ 3.63 (s, 2H, COCH2); 6.97 (t, 1H, J = 6.8 Hz, H-5); 7.07 (t, 1H, J = 7.2 Hz, H-6); 7.23–7.61 (m, 7H, Ar-H); 9.60, 9.78, 10.13, 10.90, (s, 4H, NH–NH–CS–1NH and NH indole); 13C NMR: δ 31.15, 108.38, 111.76, 118.75, 119.25, 121.41, 124.46, 127.68, 130.10, 136.50, 141.33, 141.44, 160.76, 163.15, 164.568, 165.87, 167.30, 168.76, 170.22 (C=O); 175.49, 181.17 (C=S); ESI MS m/z 343 (M + H, 100%); Anal. calcd. for C17H15FN4OS: C, 59.63%; H, 4.42%; N, 16.36%. Found: C, 59.91%; H, 4.51%; N, 16.51%.
2-(2-(1H-Indol-3-yl)acetyl)-N-(2,4-dichlorophenyl)hydrazincarbothioamide (1g). Yield 91%, m.p. 172.5 °C; 1H NMR: δ 3.62 (s, 2H, COCH2); 6.97 (t, 1H, J = 7.2 Hz, H-5); 7.07 (t, 1H, J = 6.8 Hz, H-6); 7.59 (d, 1H, J = 8 Hz, H-4); 7.25–7.68 (m, 5H, Ar–H); 9.47, 9.87, 10.20, 10.90 (s, 4H, NH–NH–CS–NH and NH indole); 13C NMR: δ 31.01, 108.31, 111.71, 118.72, 119.29, 121.41, 124.45, 127.73, 129.23, 132.34, 136.46, 171.07 (C=O); 182.14 (C=S); ESI MS m/z (M+, 100%); 395 (M + 2, 85%); 397 (M + 4, 38%); Anal. calcd. for C17H14Cl2N4OS: C, 51.92%; H, 3.59%; N, 14.25%. Found: C, 52.30%; H, 3.92%; N, 14.51%.
2-(2-(1H-Indol-3-yl)acetyl)-N-(3-chlorophenyl)hydrazincarbothioamide (1h). Yield 84%, m.p. 168 °C; 1H NMR: δ 3.64 (s, 2H, COCH2); 6.98 (t, 1H, J = 6.8 Hz, H-5); 7.07 (t, 1H, J = 7.2 Hz, H-6); 7.21 (d, 1H, J = 7.2 Hz, H-2); 7.60 (d, 1H, J = 1.6 Hz, H-4); 7.26–7.44 (m, 5H, Ar–H); 9.63, 9.81, 10.13, 10.91 (s, 4H, NH–NH–CS–NH and NH indole); 13C NMR: δ 25.81, 31.15, 108.35, 111.74, 118.78, 119.24, 121.44, 124.45, 125.18, 127.68, 130.13, 136.49, 141.12, ESI MS m/z 359.5 (M + H, 72%), 361 (M + H + 2, 28%); Anal. calcd. for C17H15N4SOCl: C, 56.90%; H, 4.21%; H, 15.61%. Found: C, 57.26%; H, 4.41%; N, 15.31%.
General procedure for the synthesis of compounds 2a–h
Conc. sulfuric acid (3 ml) was placed in a conical flask, and 2-(1H-Indol-3-yl-acetyl)-N-(aryl or alkyl)hydrazincarbothioamide (1 mmol) was added in small portions over a period of 2 h under stirring while maintaining the temperature at 0–5 °C. When the reaction was completed the mixture was poured into crushed ice and neutralized with 2 N NH4OH dropwise until the pH was adjusted to 7. The formed precipitate was filtered, washed with water, dried at room temperature and recrystallized from absolute ethanolCitation35.
5-((1H-Indol-3-yl)methyl)-2-ethylamino-1,3,4-thiadiazole (2a). Yield 42%, m.p. 125 °C; 1H NMR: δ 1.12 (t, 3H, J = 6.8 Hz, CH3); 3.21 (q, 2H, CH2); 4.26 (s, 2H, Ar–CH2);6.98 (m, 1H, H-5); 7.09 (m, 1H, H-6); 7.30 (d, 1H, J = 2.4, H-2); 7.36 (d, 1H, J = 8 Hz, H-7); 7.46 (d, 1H, J = 8 Hz, H-4); 7.73 (s, 1H, NH); 11.01 (s, 1H, NH indole); 13C NMR: δ 14.62, 26.51, 39.31(NH–C); 110.94, 112.02, 118.76, 119.11, 121.73, 124.25, 126.99, 136.75, 159.29, 168.89 (S–C–N); ESI MS m/z 259 (M + H, 100%), 300 (M + H + CH3CN, 76%); Anal. calcd. for C13H14N4S: C, 57.43%, H, 5.75%, N, 20.61%. Found: C, 57.55%, H, 5.66%, N, 20.23%.
5-((1H-Indol-3-yl)methyl)-2-propylamino-1,3,4-thiadiazole (2b). Yield 44%, m.p. 148 °C; 1H NMR: δ 0,86 (t, 3H, J = 7.6 Hz, CH3); 1.52 (m, 2H, CH2); 3.15 (q, 2H, NH–CH2); 4.26 (s, 2H, Ar–CH2); 6.98 (t, 1H, J = 8 Hz, H-5); 7.09 (t, 1H, J = 8 Hz, H-6); 7.31 (d, 1H, J = 2.4 Hz, H-2); 7.36 (d, 1H, J = 8 Hz, H-7); 7.46 (d, 1H, J = 8.4 Hz, H-4); 7,82 (s, 1H, NH); 11.00 (s, 1H, NH-indole); 13C NMR: δ 11.78, 22.16, 26.51, 47.05 (NH–C); 110.91, 112.03, 118.77, 119.12, 121.74, 124.29, 127.01, 136.76, 159.22, 169.06 (N–C–S); ESI MS m/z (M + H, 100%), 314 (M + H + CH3CN, 100%); Anal. calcd. for C14H16N4S: C, 51.51%; H, 6.79%; N, 17.16%. Found: C, 51.23%; H, 5.57%; N, 16.78%.
5-((1H-Indol-3-yl)methyl)-2-amino-1,3,4-thiadiazole (2c). Yield 39%, m.p. 177 °C; 1H NMR: δ 4.22 (s, 2H, Ar–CH2); 6.94 (bs, 2H, NH2); 6.97 (dt, 1H, J1 = 8.4, J2 = 1.2 Hz, H-5); 7.07 (dt, 1H, J1 = 8.4, J2 = 1.2 Hz H-6); 7.27 (d,1H, J = 2.4 Hz, H-2); 7.35 (d, 1H, J = 8 Hz, H-7); 7.45 (d, 1H, J = 8 Hz, H-4); 10.97 (s, 1H, NH-indole); 13C NMR: δ 25.98, 110.98, 111.42, 118.24, 118.48, 121.11, 123.52, 126.47, 136.18, 159.21, 168.41 (N–C–S); ESI MS m/z (M + H, 37%), 272 (M + H + CH3CN, 100%); Anal. calcd. for C11H10N4S: C, 55.21%, H, 4.63%, N, 23.41%. Found: C, 54.90%, H, 4.58%, N, 23.35%.
5-((1H-Indol-3-yl)methyl)-2-(2,4-difluorophenyl)amino-1,3,4-thiadiazole (2e). Yield 26%, m.p. 141 °C; 1H NMR: δ 4.34 (s, 2H, Ar–CH2); 6.70–8.33 (m,8H, Ar–H); 9.89 (s, 1H, NH); 11.01 (s, 1H, NH indole); ESI MS m/z 343 (M + H, 100%), 384 (M + H + CH3CN, 44%); Anal. calcd. for C17H12 F2N4S: C, 58.11%, H, 3.73%, N, 15.94%. Found: C, 57.98%, H, 3.63%, N, 15.80%.
5-((1H-Indol-3-yl)methyl)-2-(3-fluorophenyl)amino-1,3,4-thiadiazole (2f). Yield 22%, m.p. 183 °C; 1H NMR: δ 4.36 (s, 2H, Ar-CH2); 6.73–7.63 (m, 9H, Ar-H); 10.36 (s, 1H, NH); 11.01 (s, 1H, NH indole); 13C NMR: δ 26.36; 104.38; 104.64; 108.28; 110.94; 112.06; 113.51; 118.76; 119.18; 121.77; 124.34; 126.97; 131.02; 136.77; 124.75; 161.88; 164.25 (S–C–N); ESI MS m/z 325 (M + H, 100%), 366 (M + H + CH3CN, 33%); Anal. calcd. for C17H13FN4S: C, 61.24%, H, 4.23%, N, 16.81%. Found: C, 61.16%, H, 4.07%, N, 16.69%.
5-((1H-Indol-3-yl)methyl)-2-(2,4-dichlorophenyl)amino-1,3,4-thiadiazole (2g). Yield 50%, m.p. 112 °C; 1H NMR: δ 4.35 (s, 2H, Ar–CH2); 6.97 (m, 1H, H-5); 7.07 (m, 1H, H-6); 7.39 (d, 1H, J = 2.4 Hz, H-7); 7.48 (d, 1H, J = 7.6 Hz, H-4); 7.21–7.59 (m, 4H, Ar-H); 8.33 (d, 1H, J = 9.2 Hz, NH); 11.02 (s, 1H, NH indole);13C NMR: δ 25.78; 110.38; 111.51; 118.22; 118.61; 121.21; 121.89; 122.74; 123.81; 126.08; 126.38; 127.74; 128.76; 136.21; 136.48; 162.66; 164.11 (S–C–N); ESI MS m/z 375 (M+, 100%), 377 (M + 2, 68%), 379 (M + 4, 13%), 416 (M++CH3CN, 77%); Anal. calcd. for C17H12Cl2N4S: C, 49.43%, H, 3.95%, N, 13.56%. Found: C, 49.03%, H, 3.54%, N, 14.03%.
General procedure for the synthesis of compounds 3a–h
2-(1H-Indol-3-yl-acetyl)-N-(aryl or alkyl)hydrazincarbothioamide (2 mmol) and 2 N NaOH solution (25 ml) were placed in conical flask. The mixture was heated under reflux for 4–5 h. After completion of the reaction, the reaction mixture was neutralized with 2 N HCl dropwise till pH was adjusted to 7. The mixture was kept aside for a few minutes. The precipitate thus obtained was filtered, washed with water and recrystallized from a mixture of ethanol/water (4:1)Citation35.
5-((1H-Indol-3-yl)methyl)-4-ethyl-4H-1,2,4-triazole-3-thiol (3a). Yield 91%, m.p. 191.5 °C; 1H NMR: δ 0.92 (t, 3H, J = 7.6 Hz, CH3); 3.90 (q, 2H, CH2); 4.20 (s, 2H, Ar–CH2); 6.98 (dt, 1H, J1 = 7.2, J2 = 0.8 Hz, H-5); 7.09 (dt, 1H, J1 = 7.2, J2 = 1.2 Hz H-6); 7.33 (d, 1H, J = 2.4 Hz, H-2); 7.37 (d, 1H, J = 8 Hz, H-7); 7.49 (d, 1H, J = 7.6 Hz, H-4); 11.05 (s, 1H, NH indole); 13.54 (s, 1H, SH); 13C NMR: δ 13.31; 22.34; 38.72; 107.70; 112.057; 118.73; 119.16; 121.76; 124.61; 127.14; 136.70; 151.70; 166.67 (N–C–S); ESI MS m/z 259 (M + H, 100%), 300 (M + H + CH3CN, 8%); Anal. calcd. for C13H14N4S: C, 57.63%, H, 5.73%, N, 20.68%. Found: C, 57.93%, H, 5.96%, N, 20.51%.
5-((1H-Indol-3-yl)methyl)-4-propyl-4H-1,2,4-triazole-3-thiol (3b). Yield 76%, m.p. 151.5 °C; 1H NMR: δ 0.73 (t, 3H, J = 7.6 Hz, CH3); 1.35 (m, 2H, CH2); 3.78 (t, 2H, J = 7.6 Hz, NH–CH2); 4.19 (s, 2H, Ar–CH2); 6.98 (t, 1H, J = 7.2 Hz, H-5); 7.09 (t, 1H, J = 7.2 Hz, H-5); 7.33 (d, 1H, J = 2 Hz, H-2); 7.37 (d, 1H, J = 8 Hz, H-7); 7.47 (d, 1H, J = 7.6 Hz, H-7); 11.047 (s, 1H, NH indole); 13.54 (s, 1H, SH); 13C NMR: δ 10.59; 20.51; 21.77; 44.42 (N–C); 107.12; 111.50; 118.14; 118.58; 121.20; 124.00; 126.57; 136.13; 151.27; 166.40 (N–C–S); ESI MS m/z 273 (M + H, 100%); Anal. calcd. for C14H16N4S: C, 60.73% H, 6.01%, N, 20.23%. Found: C, 60.25%, H, 5.79%, N, 19.77%.
5-((1H-Indol-3-yl)methyl)-4-(2,4-difluorophenyl-4H-1,2,4-triazole-3-thiol (3e). Yield 85%, m.p. 242.5 °C; 1H NMR: δ 3.96 (q, 2H, Ar–CH2); 6.70–7.48 (m, 8H, Ar–H); 10.86 (s, 1H, NH indole); 13.85 (s, 1H, SH); ESI MS m/z 343 (M + H, 100%); Anal. calcd. for C17H12 F2N4S: C, 59.63%, H, 3.53%, N, 16.36%. Found: C, 59.50%, H, 3.77%, N, 16.21%.
5-((1H-Indol-3-yl)methyl)-4-(3-fluorophenyl)-4H-1,2,4-triazole-3-thiol (3f). Yield 94%, m.p. 134 °C; 1H NMR: δ 3.96 (s, 2H, Ar–CH2); 6.67–7.49 (m, 9H, Ar-H); 10.85 (bs, 1H, NH indole); 13C NMR: δ 22.76; 107.56; 111.84; 116.08; 116.24; 116.32; 116.45; 118.58; 118.89; 121.51; 124.07; 124.98; 127.04; 130.92; 131.02; 136.28; 136.43; 151.38; 160.98; 163.41; 167.94 (N–C–S); ESI MS m/z 325 (M + H, 100%); Anal. calcd. For C17H13FN4S: C, 55.54%, H, 4.88%, N, 15.24%. Found: C, 55.03%, H, 3.96%, N, 15.04%.
5-((1H-Indol-3-yl)methyl)-4-(2,4-dichlorophenyl)-4H-1,2,4-triazole-3-thiol (3g). Yield 89%, m.p. 254.5 °C; 1H NMR: δ 3.91 (q, 2H, Ar–CH2); 6.92 (t, 1H, J = 8 Hz, H-5); 7.05 (t, 1H, J = 8 Hz, H-6); 7.497 (d, 1H, J = 6.4 Hz, H-7); 7.52 (d, 1H, J = 2.4 Hz, H-4); 6.77–7.78 (m, 4H, Ar-H); 10.91 (s, 1H, NH indole); 13.86 (s, 1H, SH); 13C NMR: δ 22.01; 105.87; 111.30; 117.80; 118.39; 120.99; 123.90; 126.52; 128.28; 129.60; 130.13; 132.05; 133.22; 135.23; 135.91; 151.11; 167.735 (N–C–S); ESI MS m/z 375 (M+, 100%), 377 (M + 2, 58%), 379 (M + 4, 13%), 426 (M + CH3CN, 11%); Anal. calcd. for C17H12Cl2N4S: C, 53.76%, H, 3.32%, N, 14.75%. Found: C, 53.57%, H, 3.29%, N, 14.69%.
5-((1H-Indol-3-yl)methyl)-4-(3-chlorophenyl)-4H-1,2,4-triazole-3-thiol (3h). Yield 83%, m.p. 140 °C; 1H NMR: δ 3.96 (s, 2H, Ar–CH2); 6.91 (t, 1H, J = 7.2 Hz, H-5); 7.028 (t, 1H, J = 7.6 Hz, H-6); 7.19 (d, 1H, J = 7.6 Hz, H-2); 6.71–7.58 (m, 6H, Ar–H); 10.86 (s,1H, NH indole); 13.77 (s, 1H, SH); 13C NMR: δ 22.73; 107.03; 111.88; 118.50; 118.97; 121.57; 124.26; 126.99; 127.56; 128.74; 129.78; 131.09; 133.57; 135.56; 136.43; 151.72;168.14 (S–C–N); ESI MS m/z 341.2 (M+, 100%); 343.3 (M + 2, 30%), 382 (M + CH3CN, 20%); Anal. calcd. for C17H13ClN4S: C, 58.36%, H, 4.03%, N, 16.01%. Found: C, 58.54%, H, 4.01%, N, 15.70%.
General procedure for the synthesis of compounds 4a–g
5-((1H-Indol-3-yl) methyl)-4-(substituted aryl/alkyl)-4H-1,2,4-triazole-3-thiol (1 mmol) was dissolved in a solution of 2 N KOH (2 ml) and absolute ethanol (20 ml) in a conical flask. R-X (X = Cl) (1 mmol) was added in to the mixture and stirred at room temperature for 2–3 h. After completion of the reaction, the reaction mixture was poured onto crushed ice. The formed precipitate was filtered, washed with water, dried at room temperature and recrystallized from absolute ethanolCitation33.
2-(5-((1H-Indol-3-yl)methyl)-4-ethyl-4H-1,2,4-triazole-3-ylthio)-N-phenylacetamide (4a). Yield 17%, m.p. 120 °C; 1H NMR: δ 0.96 (t, 3H, J = 6.8 Hz, CH3); 3.91 (q, 2H, CH2); 4.09 (s, 2H, Ar–CH2); 4.25 (s, 2H, S–CH2–CO); 6.92–7.55 (10H, m, Ar–H); 10.32 (s, 1H, amide-NH); 10.97 (s,1H, NH indole); 13C NMR: δ 14.55; 21.43; 28.90; 37.51; 108.30; 111.37; 118.40; 118.45; 119.01; 121.11; 123.42; 123.61; 126.65; 128.69; 136.16; 138.66; 148.32; 154.44 (N–C–S); 165.62 (C=O); ESI MS m/z 392 (M + H, 100%); Anal. calcd. for C21H21N5OS: C, 56.04%, H, 6.15%, N, 15.56%. Found: C, 55.68%, H, 5.60%, N, 15.22%.
2-(5-((1H-Indol-3-yl)methyl)-4-propyl-4H-1,2,4-triazole-3-ylthio)-N-propylacetamide (4b). Yield 32%, m.p. 79 °C; 1H NMR: δ 0.71 (t, 3H, CH3); 0.79 (t, 3H, J = 7.2 Hz, CH3); 1.34 (m, 2H, CH2); 1.43 (m, 2H, CH2); 2.97 (q, 2H, CO–NH–CH2); 3.78 (t, 2H, J = 7.6, triazole–NH–CH2); 3.82 (s, 2H, Ar–CH2); 4.23 (s, 2H, S–CH2–CO); 6.94 (t,1H, J = 8 Hz, H-5); 7.06 (t,1H, J = 8.4 Hz, H-6); 7.25 (d, 1H, J = 2 Hz, H-2); 7.34 (d, 1H, J = 8.4 Hz, H-7); 7.50 (d, 1H, J = 8 Hz, H-4); 8.16 (bs, 1H, amide–NH); 10.960 (s,1H, NH indole); 13C NMR: δ 11.08; 11.72; 22.06; 22.59; 22.99; 37.29; 43.12; 45.26; 79.63; 108.95; 111.90; 118.95; 121.65; 124.07; 127.21; 136.71; 149.14; 155.04; 167.12 (C=O); ESI MS m/z 372.3 (M + H, 100%); Anal. calcd. for C19H25N5OS: C, 58.18%, H, 7.02%, N, 17.85%. Found: C, 58.77%, H, 6.96%, N, 17.13%.
2-(5-((1H-Indol-3-yl)methyl)-4-benzyl-4H-1,2,4-triazole-3-ylthio)-N-(2-fluorophenyl)acetamide (4c). Yield 30%, m.p. 182.5 °C; 1H NMR: δ 4.13(s, 2H, indole-CH2); 4.20 (s, 2H, benzyl-CH2); 5.18 (s, 2H, amide-CH2); 6.92–7.88 (m, 14H, Ar-H); 10.16 (s, 1H, amide-NH); 10.91 (s, 1H, NH-indole); 13C NMR: δ 22.20; 37.64 (S–C); 47.02 (N–C); 108.48; 111.89; 115.87; 116.07; 118.98; 121.67; 124.09; 124.25; 124.87; 125.86; 126.27; 126.38; 127.02; 127.22; 128.20; 129.08; 135.832; 136.76; 149.86; 152.56; 155.00; 155.46; 166.82 (C=O); ESI MS m/z 472.2 (M + H, 100%); Anal. calcd. for C26H22 FN5OS: C, 66.22%, H, 4.70%, N, 14.85%. Found: C, 65.73%, H, 5.08% N, 14.36%.
2-(5-((1H-İndol-3-yl)methyl)-4-phenyl-4H-1,2,4-triazole-3-ylthio)-N-propylacetamide (4d). Yield 17%, m.p. 165.5 °C; 1H NMR: δ 0.89 (t, 3H, J = 7.6 Hz, CH3); 1.53 (m, 2H, CH2); 3.20 (q, 2H, CO–NH–CH2); 3.73 (s, 2H, Ar–CH2); 4.16 (s, 2H, S–CH2–CO); 6.61–7.50 (m, 10H, Ar–H); 7.96 (bs, 1H, amide-NH); 8.10 (s, 1H, NH indole); 13C NMR: δ 11.39; 22.05; 22.56; 34.56; 41.56; 109.34; 110.00; 111.10; 118.78; 119.71; 122.28; 122.69; 126.63; 127.02; 129.78; 130.19;132.72; 136.02; 152.17; 155.39 (N–C–S); 168.68 (C=O); ESI MS m/z 406 (M + H, 100%); Anal. calcd. for C22H23 N5OS: C, 64.44%, H, 5.78%, N, 17.08%. Found: C, 64.21%, H, 5.88%, N, 16.85%.
2-(5-((1H-Indol-3-yl)methyl)-4-(2,4-difluorophenyl)-4H-1,2,4-triazole-3-ylthio)-N-propylacetamide (4e). Yield 45%, m.p. 140 °C; 1H NMR: δ 0.81 (t, 3H, J = 7.6 Hz, CH3); 1.37 (m, 2H, CH2); 2.99 (q, 2H, CO-NH-CH2); 3.85 (s, 2H, Ar–CH2); 4.08 (s, 2H, S–CH2–CO); 6.69–7.51 (m, 8H, Ar–H); 8.16 (t, 1H, J = 5.2 Hz, amide-NH); 10.81 (s, 1H, NH indole); ESI MS m/z 442 (M + H, 100%); Anal. calcd. for C22H21F2N5OS: C, 59.85%, H, 4.79%, N, 15.86%. Found: C, 59.70%, H, 4.97%, N, 15.66%.
2-(5-((1H-Indol-3-yl)methyl)-4-(3-fluorophenyl)-4H-1,2,4-triazole-3-ylthio)-N-propylacetamide (4f). Yield 40%, m.p. 145 °C; 1H NMR: δ 0.81 (t, 3H, J = 7.6 Hz, CH3); 1.37 (m, 2H, CH2); 2.98 (q, 2H, CO-NH-CH2); 3.84 (s, 2H, Ar–CH2); 4.11 (s, 2H, S-CH2-CO); 6.69–7.55 (m, 9H, Ar-H); 8.16 (t, 1H, J = 5.2 Hz, amide-NH); 10.80 (s, 1H, NH indole); 13C NMR: δ 11.75; 22.025; 22.61; 36.69; 41.16; 108.36; 111.77; 115.19; 115.43; 117.18; 117.38; 118.63; 118.87; 121.53; 123.84; 124.03; 124.06; 127.02; 131.66; 131.75; 134.87; 134.97; 136.45; 150.10; 155.134; 161.13; 163.58 (N–C–S); 166.85 (C=O); ESI MS m/z 424 (M + H, 100%); Anal. calcd. for C22H22FN5OS: C, 62.39%, H, 5.24%, N, 16.54%. Found: C, 62.32%, H, 5.28%, N, 16.35%.
2-(5-((1H-Indol-3-yl)methyl)-4-(2,4-dichlorophenyl)-4H-1,2,4-triazole-3-ylthio)-N-phenylacetamide (4g). Yield 15%, m.p. 219 °C; 1H NMR: δ 4.03 (s, 2H, Ar–CH2); 4.12(s, 2H, S–CH2–CO); 6.72–7.80 (m, 13H, Ar–H); 10.27 (s,1H, amide-NH); 10.82 (s, 1H, NH indole); 13C NMR: δ 21.47; 37.30; 107.15; 111.19; 117.96; 118.28; 118.99; 120.93; 123.43; 123.47; 126.56; 128.51; 128.69; 129.36; 129.76; 131.13; 132.53; 135.68; 135.90; 138.61; 149.66; 154.80 (N–C–S); 165.26 (C=O); ESI MS m/z 508 (M+, 100%), 510 (M + 2, 75%), 512 (M + 4, 16%); Anal. calcd. for C25H19Cl2N5OS: C, 59.06%, H, 3.77%, N, 13.77%. Found: C, 59.60%, H, 4.27%. N, 13.47%.
Experimental – biological activity
Cell culture and reagents
CHO-K1 cells were purchased from ATCC and maintained at 37 °C in 5% CO2 atmosphere. Dulbecco’s modified Eagle’s medium/F12 (Sigma, St. Louis, MO) (1:1) nutrient mixture medium supplemented with 10% foetal bovine serum (FBS), 1% of a 100 U/ml penicillin–streptomycin solution, 2 mM (final concentration) l-glutamine and 1 mM (final concentration) sodium pyruvate was used in all cell incubations. Hyclone characterized FBS is purchased from Thermo Scientific (Waltham, MA). 2′,7′-Dichlorofluorescin diacetate (DCFH-DA), DPPH, sodium pyruvate and l-glutamine were obtained from Sigma (St. Louis, MO). 3-(4,5-dimethyl-2-thiazolyl)-2,5-diphenyl-2H-tetrazolium bromide (MTT) was purchased from Invitrogen (Waltham, MA). Test materials were dissolved in dimethyl sulfoxide (DMSO) and the final concentration of DMSO never exceeded 0.1% v/v in cell culture medium.
Cytotoxic effects via the MTT assay
CHO-K1 cells were seeded in 96-well plates (5 × 103 cells/well) and incubated at 37 °C in a humid atmosphere containing 5% CO2 for 24 h. Cells were treated with the newly synthesized compounds (10 μM) for 24 h. Control (medium only), vehicle control and positive control (15 μM Triton X-100) were included in every experiment. Following the exposure period the medium was removed, cells were washed with phosphate buffered saline (PBS) and then incubated with MTT (1 mg/ml) for 4 h at 37 °C. MTT solution was removed and formazan crystals were dissolved in DMSO. The absorbance was recorded at 550 nm on a microplate reader. The ratio of the absorbance of treated samples to the absorbance of control (taken as 100%) was expressed as % cell viability.
Measurement of intracellular ROS (antioxidant activity on ROS-induced DCFH-DA oxidation)
For estimation of intracellular ROS, DCFH-DA was used as a probe. In cellular systems, the non-fluorescent probe DCFH-DA readily crosses the cell membrane and undergoes hydrolysis by intracellular esterases to non-fluorescent 2′,7′-dichlorofluorescin (DCFH). In the presence of ROS, DCFH is oxidized to highly fluorescent dichlorofluorescein (DCF)Citation37 which can be detected by a fluorescent microplate reader. The DCF fluorescence intensity is believed to be parallel to the amount of ROS formed intracellularly.
Cells were seeded in black 96-well plates at a density of 5 × 103 cells/well and incubated at 37 °C in a humid atmosphere containing 5% CO2 for 24 h for cell attachment. The medium was removed and then cells were incubated with DCFH-DA (20 μM) containing medium for 30 min. Cells were washed with PBS to remove excess DCFH-DA. Ten micromolar synthesized compounds and 10 μM cumene hydroperoxide (CMHP) were added into medium. The production of fluorescent DCF was evaluated by monitoring fluorescence intensity at 488 nm excitation, 530 nm emission wavelengths for 60 min.
DPPH free radical scavenging activity
The free radical scavenging activities of newly synthesized compounds were tested by their ability to bleach the stable radical DPPHCitation38. DPPH presents a maximum of absorbance at 515 nm; when DPPH reacts with an antioxidant compound, which can donate hydrogen, this absorbance diminishes and can be measured on a visible spectrophotometer.
Twenty microlitres of the samples (100 μM) was added to 180 μl of DPPH solution (150 μM) in methanol–water (80:20, v/v) in 96-well plates, incubated for 30 min at room temperature then DPPH reduction was estimated at 517 nm. Percentage inhibition by the sample treatment was determined by comparison with a DMSO-treated control group. All experiments were carried out in triplicate. MLT and BHT were used as reference compounds. The radical scavenging activities were expressed as the inhibition percentage and were calculated using the formula: Radical scavenging activity (%) = [(A0−A1/A0) × 100], where A0 is the absorbance of the control (blank, without compound) and A1 is the absorbance of the compound.
Results and discussion
Cytotoxic effects of the synthesized compounds
The cytotoxic effect of the synthesized compounds in CHO-K1 cells was evaluated by the MTT assay (. CHO-K1 cells are used because many studies as well as our previous study suggested them as a relevant model for in vitro antioxidant screening studiesCitation39. Furthermore, CHO cells are advantageous since they have active CYP enzymes that make them a good in vitro model for detecting the toxicity of chemicals as well as some of their metabolites without adding S9 fraction.
Antioxidant effects of the synthesized compounds
The protective effect of newly synthesized MLT analogues against CMHP induced DCFH-DA oxidation was determined in CHO-K1 cells that were preloaded with the fluorescent probe. Oxidation of the probe that locates in the cytosol was screened at various time intervals up to 60 min (. Several compounds were found to have antioxidant activity, whereas 1b and 2e were found to be the most potent two compounds among all. Therefore, their reducing activity against DCFH oxidation was further investigated at their various concentrations (
Figure 3. DCFH oxidation in CHO cells after 60 min incubation with compounds (10 μM) in the presence of CMHP. Bars represent “medium ± standard deviation” values obtained from five individual experiments. Statistical comparisons of samples with CMHP were compared to CMHP alone control group. *p < 0.05, **p < 0.005 (AA, ascorbic acid).
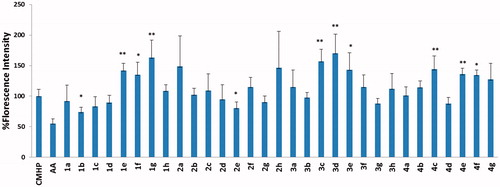
Figure 4. DCFH oxidation in CHO cells after 60 min incubation with MLT, compounds 1b and 2e in the presence of CMHP. Bars represent “medium ± standard deviation” values from five individual experiments. The values above the bars represent percentage of the absorbance values comparing with CMHP group used as control. All the statistical analysis of samples with CMHP was performed by comparing to the CMHP alone group. *p < 0.05, **p < 0.005, ***p < 0.0005 (MLT, melatonin).
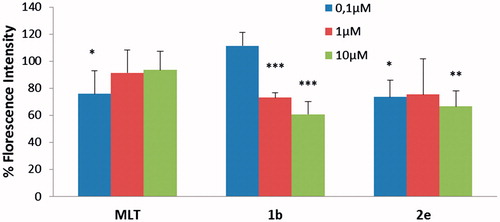
DPPH free radical scavenging activity
Free radical scavenging activity of the newly synthesized compounds was further investigated in a cell free in vitro model where an active radical, DPPH was used. The radical scavenging activity of a compound can be detected via decrease in the absorbance of DPPH. As can be seen in compounds which named as 1a–h and 3a–h are more potent radical scavengers then 2 and 4 coded compounds.
Conclusion
In this study, 31 new indole-based MLT analogues were synthesized and their antioxidant and cytotoxic effects were tested by three different assays. The MTT assay was used for determining the cytotoxic profiles in CHO-K1 cells, and the DCFH-DA and DPPH assays were preformed to reveal the antioxidant activity. According to the results of the MTT assay 1a, 1d, 2b, 2h and 4c (10 μM) showed significant proliferative effect on CHO cells. However, other compounds have demonstrated cytotoxic effects at their 10 μM concentrations. Compounds with cytotoxic effect can be tested for a possible anticancer activity in further studies due to the similarity of chemical structures to sunitinib which is a receptor tyrosine kinase inhibitorCitation40 and was approved by the FDA for the treatment of renal cell carcinoma. According to the DCFH-DA assay in the presence of CMHP with p < 0.05 degree of significance, 1b and 2e were observed with the highest antioxidant effect, respectively, with values of 74% and 81%. This was followed by 1c, 3 g, 4d, 1d, respectively, with the rate of 83%, 88% and 89%. The concentration dependence effect of the most active compounds 1b and 2e was investigated against MLT at the same concentration. Depending on the dose of 1b and 2e when the concentration is increasing the antioxidant effect is also increasing and this effect is more potent than MLT at concentrations of 1 and 10 μM.
The DPPH assay is a cell-free in vitro screening test for the radical scavenging activity. Among newly synthesized compounds generally hydrazinecarbothioamide (1a–h) and 1,2,4-triazole-3-thiol (3a–h) derivatives were found to have high scavenging activity whereas (4a–g) derivatives were found to have the least scavenging activity. Compound 1b which was found to have the highest antioxidant effect in the cell based in vitro DCFH assay was found to have high scavenging activity in DPPH assay too. This finding indicates that the antioxidant effect of 1b is probably is a result of its radical scavenging activity. However, compound 2e which was also found to have antioxidant activity according to the DCFH assay, and was found to have very low scavenging activity in the DPPH assay which suggests an alternative mechanism for its antioxidant activity. However, almost all hydrazincarbothioamide and 1,2,4-triazole-3-thiol derivatives were found to have radical scavenging activity in the DPPH assay where no antioxidant activity was observed in the cell based DCFH assay. This result could be the result of limited availability of the compounds in cell cytosol because of the possible membrane passage. This speculation needs to be further verified experimentally.
Declaration of interest
The authors report no conflicts of interest. The chemical synthesis and characterization section of this work were supported by The Scientific and Technological Research Council of Turkey (TÜBİTAK) Research and Development Grant 112S599. The cell culture facility was established by the support of a TUBITAK 108S202 Grant.
References
- Valko M, Rhodes CJ, Moncol J, et al. Free radicals, metals and antioxidants in oxidative stress-induced cancer. Chem Biol Interact 2006;160:1–40
- Wu JQ, Kosten TR, Zhang XY. Free radicals, antioxidant defense systems, and schizophrenia. Progr Neuro-Psychopharmacol Biol Psychiatr 2013;46:200–6
- Bonnefont-Rousselot D, Collin F. Melatonin: action as antioxidant and potential applications in human disease and aging. Toxicology 2010;278:55–67
- Valko M, Leibfritz D, Moncol J, et al. Free radicals and antioxidants in normal physiological functions and human disease. Int J Biochem Cell Biol 2007;39:44–84
- Yamashita T, Sakamoto K, Yamanishi H, et al. Effect of a free radical scavenger on nitric oxide release in microvessels. Vasc Pharmacol 2013;58:134–9
- Poeggeler B, Pappolla MA, Hardeland R, et al. Indole-3-propionate: a potent hydroxyl radical scavenger in rat brain. Brain Res 1999;815:382–8
- Fernandez D, Bonilla E, Phillips P, et al. Signaling abnormalities in systemic lupus erythematosus as potential drug targets. Endocr Metab Immune Disord Drug Targets 2006;6:305–11
- Guglielmotto M, Tamagno E, Danni O. Oxidative stress and hypoxia contribute to Alzheimer's disease pathogenesis: two sides of the same coin. Sci World J 2009;9:781–91
- Dong XX, Wang Y, Qin ZH. Molecular mechanisms of excitotoxicity and their relevance to pathogenesis of neurodegenerative diseases. Acta Pharmacol Sin 2009;30:379–87
- Yilmaz AD, Coban T, Suzen S. Synthesis and antioxidant activity evaluations of melatonin-based analogue indole-hydrazide/hydrazone derivatives. J Enzyme Inhib Med Chem 2012;27:428–36
- Militante J, Lombardini JB. Age-related retinal degeneration in animal models of aging: possible involvement of taurine deficiency and oxidative stress. Neurochem Res 2004;29:151–60
- Pieri C, Moroni F, Marra M, et al. Melatonin is an efficient antioxidant. Arch Gerontol Geriatr 1995;20:159–65
- Ressmeyer AR, Mayo JC, Zelosko V, et al. Antioxidant properties of the melatonin metaboliteN1-acetyl-5-methoxykynuramine (AMK): scavenging of free radicals and prevention of protein destruction. Redox Report 2003;8:205–13
- Süzen S. Antioxidant activities of synthetic indole derivatives and possible activity mechanisms. Top Heterocycl Chem 2007:11:145–78
- Allegra M, Reiter RJ, Tan DX, et al. The chemistry of melatonin’s interaction with reactive species. J Pineal Res 2003;34:1–10
- Reiter RJ, Tan DX, Cabrera J, et al. The oxidant/antioxidant network: role of melatonin. Biol Signals Recept 1999;8:56–63
- Gürkök G, Coban T, Süzen S. Melatonin analogue new indole hydrazide/hydrazone derivatives with antioxidant behavior: synthesis and structure–activity relationships. J Enzyme Inhib Med Chem 2009;24:506–15
- Korkmaz A, Reiter RJ, Tan DX, et al. Melatonin; from pineal gland to healthy foods. Spatula DD 2011;1:33–6
- Mallo C, Zaidan R, Galy G, et al. Pharmacokinetics of melatonin in man after intravenous infusion and bolus injection. Eur J Clin Pharmacol 1990;38:297–301
- Vakkuri O, Leppäluoto J, Kauppila A. Oral administration and distribution of melatonin in human serum, saliva and urine. Life Sci 1985;37:489–95
- Favero G, Rodella LF, Reiter RJ, et al. Melatonin and its atheroprotective effects: a review. Mol Cell Endocrinol 2014;382:926–37
- Cano A, Alcaraz O, Arnao M. Free radical-scavenging activity of indolic compounds in aqueous and ethanolic media. Anal Bioanal Chem 2003;376:33–7
- Andreadou I, Tsantili-Kakoulidou A, Spyropoulou E, et al. Reactions of indole derivatives with cardioprotective activity with reactive oxygen species. Comparison with melatonin. Chem Pharm Bull (Tokyo) 2003;51:1128–31
- Shirinzadeh H, Yilmaza AD, Gumustasb M, et al. Electrochemical behavior of indole-3-carboxaldehyde isonicotinoyl hydrazones: discussion on possible biological behavior. Comb Chem High Throughput Screen 2010;13:619–27
- Suzen S, Tekiner-Gulbas B, Shirinzadeh H, et al. Antioxidant activity of indole-based melatonin analogues in erythrocytes and their voltammetric characterization. J Enzyme Inhib Med Chem 2013;28:1143–55
- Suzen S, Ates-Alagoz Z, Demircigil BT, et al. Synthesis and analytical evaluation by voltammetric studies of some new indole-3-propionamide derivatives. Farmaco 2001;56:835–40
- Khan I, Ali S, Hameed S, et al. Synthesis, antioxidant activities and urease inhibition of some new 1,2,4-triazole and 1,3,4-thiadiazole derivatives. Eur J Med Chem 2010;45:5200–7
- Koparir M, Orek C, Koparir P, et al. Synthesis, experimental, theoretical characterization and biological activities of 4-ethyl-5-(2-hydroxyphenyl)-2H-1,2,4-triazole-3(4H)-thione. Spectrochim Acta A Mol Biomol Spectrosc 2013;105:522–31
- Kus C, Ayhan-Kilcigil G, Ozbey S, et al. Synthesis and antioxidant properties of novel N-methyl-1,3,4-thiadiazol-2-amine and 4-methyl-2H-1,2,4-triazole-3(4H)-thione derivatives of benzimidazole class. Bioorg Med Chem 2008;16:4294–303
- Varvaresou A, Tsantılı-Kakoulıdou A, Sıatra-Papastaıkoudı T, et al. Synthesis and biological evaluation of indole containing derivatives of thiosemicarbazide and their cyclic 1,2,4-triazole and 1,3,4-thiadiazole analogs. Arzneimittelforschung 2000;50:48–54
- Chen H, Li Z, Han Y. Synthesis and fungicidal activity against Rhizoctonia solani of 2-alkyl (alkylthio)-5-pyrazolyl-1,3,4-oxadiazoles (thiadiazoles). J Agric Food Chem 2000;48:5312–15
- Mathew V, Gıles D, Keshavayya J, et al. Studies on synthesis and pharmacological activities of 1,2,4-triazolo[3,4-b]1,3,4-thiadiazoles and their dihydro analogues. Arch Pharm (Weinheim) 2009;342:210–22
- Kharadı GJ. Antioxidant, tautomerism and antibacterial studies of Fe(III)-1,2,4-triazole based complexes. Spectrochim Acta A Mol Biomol Spectrosc 2013;110:311–16
- Bansod S, Kamble R. Synthesis of novel 2-(3′-aryl-sydnon-4′-ylidene)-5′-substituted-[1,3,4]-thiadiazolylamines and [1,3,4]-thiadiazol-2′-yl-3-oxo-[1,2,4]-triazoles as antimicrobial agents. Med Chem Res 2012;21:867–73
- Siddiqui N, Alam MS, Ahsan W. Synthesis, anticonvulsant and toxicity evaluation of 2-(1H-indol-3-yl)acetyl-N-(substituted phenyl)hydrazine carbothioamides and their related heterocyclic derivatives. Acta Pharm (Zagreb, Croatia) 2008;58:445–54
- Siddiqui N, Alam MS. 5[(1H-indol-3-yl)methyl]-N-(phenyl)-1,2,4-thiadiazol-2-amine derivatives: synthesis and biological screening. Biosci Biotechnol Res Asia 2009;6:261–4
- Lautraite S, Bigot-Lasserre D, Bars R, et al. Optimisation of cell-based assays for medium throughput screening of oxidative stress. Toxicol In Vitro 2003;17:207–20
- Blois MS. Antioxidant determination by the use of stable free radical. Nature 1958;181:1199–200
- Orhan H, Gurer-Orhan H, Vriese E, et al. Application of lipid peroxidation and protein oxidation biomarkers for oxidative damage in mammalian cells. A comparison with two fluorescent probes. Toxicology in Vitro 2006;20:1005–13
- Papaetis GS, Syrigos KN. Sunitinib: a multitargeted receptor tyrosine kinase inhibitor in the era of molecular cancer therapies. BioDrugs 2009;23:377–89