Abstract
Cancer cells reprogram their metabolic machineries to enter into permanent glycolytic pathways. The full reason for such reprogramming takes place is unclear. However, this metabolic switch is not made in vain for the lactate that is generated and exported outside cells is reused by other cells. This results in the generation of a pH gradient between the low extracellular pH that is acidic (pHe) and the higher cytosolic alkaline or near neutral pH (pHi) environments that are tightly regulated by the overexpression of several pumps and ion channels (e.g. NHE-1, MCT-1, V-ATPase, CA9, and CA12). The generation of this unique pH gradient serves as a determining factor in defining “tumor fitness”. Tumor fitness is the capacity of the tumor to invade and metastasize due to its ability to reduce the efficiency of the immune system and confer resistance to chemotherapy. In this article, we highlight the importance of tumor microenvironment in mediating the failure of chemotherapeutic agents.
Introduction
In contrast to normal eukaryotic cells that generate energy by the breakdown of pyruvate, cancer cells generate energy by the nonoxidative breakdown of glucose with tumor cells displaying glycolytic rates up to 200 times higher than normal tissues. In the early 1920s, a technician at Otto Warburg’s lab spilled NaHCO3 by accident and created an opportunity for Otto Warburg to observe that those cancer cells became highly fermentative. This has led to the development of the Warburg hypothesis that states that the driver for tumor development is a mitochondrial insult that leads to insufficient cellular respiration, explaining why even in the presence of adequate oxygen tumor cells preferentially turn to fermentation of lactic acid for an energy supply “Warburg Effect”Citation1. While in yeast the fermentation results in the formation of ethanol, in eukaryotic cells glycolysis results in the formation of lactate. Cancer cells are unique in the way by which they switch to anaerobic glycolysis in the presence of a plentiful supply of oxygenCitation1,Citation2. Glycolysis occurs through the glycolysis pathway or the pentose phosphate pathway (PPP; ).
Figure 1. A model that describes the cytoplasmic utilization of glucose through either glycolysis or PPPs.
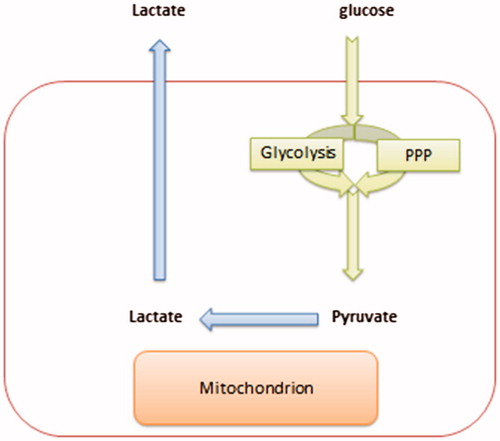
Glycolysis is an energy-rich process in that it requires two molecules of ATP to produce four ATP molecules. In contrast, PPP requires less energy as it requires only one ATP to produce four ATP molecules. Therefore, PPP has a lower metabolic cost in comparison to glycolysis. Furthermore, PPP maintains cellular redox status by providing a reduced form of glutathione which removes reactive oxygen species (ROS). Although the generation of ROS is an indication of cell death or induction of gene variation (mutation), ROS are also signaling molecules that are useful in the manipulation of cellular migrationCitation3–6. The generation of lactic acid is very helpful in allowing the progression of metastasis.
Warburg effect
Warburg effect is a cellular behavior rather than a disease that has been exploited clinically as diagnostic tool with Positron Emission Tomography (PET) scanCitation7–9 to detect a tumor and to some extent for therapeutic purposesCitation10–12. Since the 1920s the mitochondrion–cancer correlation plays a critical part of tumor biology. Otto Warburg and recent scientists have considered cancer as mitochondrial injuryCitation13–15. In particular, several studies have shown a decrease in mitochondrion number within cellsCitation16–19. Decreasing the efficiency of Krebs’ cycle pushes tumor cells to utilize the glucose in the cytoplasm either through glycolysis or the PPPCitation20–22. In this context, it is suggested that the Warburg effect reveals that some cancer undergoes a loss of mitochondrial activity (i.e. loss of endosymbiotic relationship between mitochondrion and the cell), and so most probably are returning to a primitive state (primitive microorganisms)Citation1. Diminishing the mitochondrial activity has evolutionarily advantaged such as keeping cancer cell survival through inhibition of apoptosisCitation23–25. Mitochondrial dynamic (fission/fusion processes) perturbations have been detected in cancerCitation26–28.
Causes of metabolic transformation
Numerous studies have identified the changes that occur leading to the metabolic transformation of tumor cells. In 2000, Reshkin et al. found that the first step in carcinogenesis was the development of cytoplasmic alkalinity due to Sodium-Hydrogen Exchanger-1 (NHE1) overexpression following human papilloma virus (HPV) transfectionCitation29. Later in 2007, Gatenby suggested that the Warburg effect is an adaptive strategy used by tumor cells to enable them to survive during periods of intermittent hypoxiaCitation29,Citation30.
Sodium–hydrogen exchanger1 (NHE1)
The sodium-hydrogen antiporter is a housekeeping protein found in the plasma membraneCitation31. It is an integral membrane protein having isoforms (NHE-1–9)Citation32,Citation33. These isoforms are expressed differently in tissues and localized differently sub-cellularly (). The physiological role of NHE is to maintain the pH of the cell and its organelles. Also, NHE1 acts as an anchor for actin filaments to control the integrity of the cortical cytoskeletonCitation34. This occurs through a previously unrecognized structural link between NHE1 and the actin-binding proteins ezrin, radixin, and moesin (ERM)Citation34. Therefore, it plays a role in the regulation of the cytoskeleton. NHE1 is the most extensively studied of the four isoforms. It has been implicated in painCitation35, several diseases, e.g. myocardial hypertrophyCitation36 and cancer. In 2000, Reshkin et al. observed that NHE1 stimulation by HPV is a core event in malignant transformation and inhibition of NHE1 delayed such transformationCitation29. NHE1 plays a critical role in tumor progression and invasionCitation37. NHE-1 could be considered as a key regulator in tumor cell migration due to its role in the formation of lamellipodium, which are needed for migrationCitation38 and formation of invadopodium that degrades the extracellular matrixCitation39. NHE-1 may also confer resistance to cancer chemotherapy either physiologically or biochemically. Physiologically NHE1 confers resistance to chemotherapy by inhibiting the diffusion of weakly basic drugs due to extracellular acidification, or by increasing the solubility of weakly acidic drugs when reaching cytoplasm and so preventing weakly acidic drugs from reaching their target. This type of resistance is known as ion trappingCitation40–42. Biochemically, cancer cells develop antiacidifying mechanisms such as hyperactivity of the group of membrane-bound proton extrusion transporters, inactivation of Bcl-2, Bcl-xI, and/or a pH-dependent destabilization of p53Citation43. These concerted dynamic changes work as an anti-chemotherapeutic shield involved in multiple drug resistance (MDR) and in the development of newly resistant subpopulations of tumor cellsCitation43. The final therapeutic aim is to target the selective acid–base disruption of cancer cell metabolism based on the H+-dependent thermodynamic advantages that malignant cells possess for their evolutionary survival as compared to their normal counterparts. Currently, NHE1 inhibitors such as amiloride and cariporide are being investigated as potential novel chemotherapeutic agentsCitation43,Citation44. NHE1 inhibitors could be used directly due to their tumoricidal activity or they may function as chemosensitizers as in adjuvant therapyCitation45.
Table 1. The level of expression of NHE isoforms in different tissues.
Monocarboxylate transporter 1 (MCT-1)
Monocarboxylate transporters (MCTs) belong to the SLC16 gene family and are composed of 14 membersCitation46,Citation47. MCTs handle lactate and pyruvate transport across the plasma membraneCitation48,Citation49. MCT is a symporter that transports H+ and L-lactate or pyruvate bidirectionallyCitation48. Lactate is extruded continuously from transformed cells due to a switch from the Pasteur effect to the Warburg effectCitation1. The presence of lactate extracellularly preserves a favorable habitat for malignant cells and an unfavorable environment for normal cells, so it offers and evolutionary advantage to tumor cellsCitation50. Tumor cells are highly adaptable, and it has been noted that individual tumors are composed of cells of different metabolic phenotypes. As the outer edge of the tumor cells may be in contact with blood vessels and thus have adequate oxygen and nutrients to allow glycolysis and oxidative phosphorylation to occur. In contrast, those cells in the hypoxic center of the tumor will utilize this switch to anaerobic glycolysis as dictated by their microenvironment. The lactate produced by oxidative tumor cells on the outer edge of the tumor is also thought to be an important signaling molecule, also known as “lactormone” ()Citation51 and, therefore, the presence of lactate transporters is very useful in cancer growthCitation52,Citation53. MCT-1 is expressed on the plasma membrane of the cellCitation54. According to the endosymbiotic theory, mitochondria originated from bacteria that were engulfed by the plasma membraneCitation55–57. Therefore, it is not surprising that MCT-1 is localized at both the mitochondrial and peroxisomal membranes (while evolution peroxisomes originated from mitochondria)Citation58,Citation59. One of the great evolutionary advantages is that MCT-1 plays a critical role in maintaining the redox status of the cell through inter-organelle lactate shuttling within the cytoplasm of the cellCitation60. For example, NADH is regenerated from NAD+ in the cytoplasm by lactate that has been generated from pyruvate inside a peroxisome and is subsequently translocated into the cytoplasm via MCTCitation60. The presence of this shuttle is crucial to maintaining beta-oxidation of free fatty acids. Upregulation of this oxidation process has been correlated with cancer and its progressionCitation61. MCT1 also interacts with CD147/basigin and promotes cellular migrationCitation62,Citation63. Moreover, MCT1 overexpression is correlated with metastasisCitation64. MCT1 has been used as a prognostic factorCitation65, and it is thought to contribute to tumor recurrence (relapse)Citation66 and may mediate chemotherapy resistance tooCitation67. MCT1 is thought to be as a selective target in cancerCitation68,Citation69.
Figure 2. A tumor colony consists of a heterogeneous group of cell population that clusters around the blood vessel.
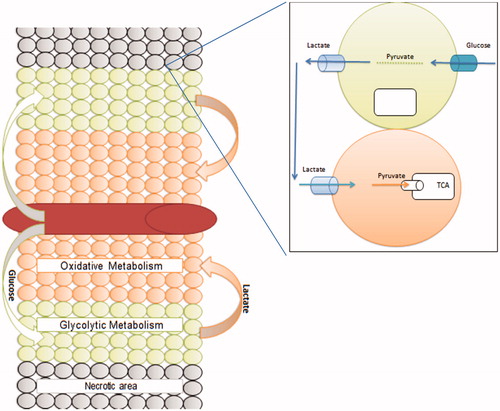
Box 1. Lactate Paradox in Cancer:
Vacuolar ATPase (V-ATPase)
In 1981, Anraku and Ohsumi discovered Vacuolar–ATPase (V-ATPase) at the membrane of Saccharomyces cerevisiaeCitation70. V-ATPase is an evolutionarily conserved transmembrane enzyme that might originate from archaebacteriaCitation71. It consists of two major domains with each domain being composed of several subunitsCitation72. V-ATPase translocates protons (H+) to maintain the intracellular cytosol and organelles at optimal pHCitation73. Organelles that contain V-ATPases are clathrin-coated vesicles, the Golgi complex, lysosomes, synaptic vesicles, and chromaffin granules. Examples of plasma membranes that contain V-ATPases are kidney proximal and distal tubule cells, the mitochondria-rich cells of the epididymis, macrophages, and osteoclastsCitation74. V-ATPase is overexpressed in cancer. It works to increase extracellular acidity and maintains cytoplasmic alkalinity tooCitation75. It has been shown that metastatic phenotypes have higher expression of V-ATPase compared to NHE1Citation76. Overexpression of V-ATPase is associated with a drug-resistant phenotypeCitation77,Citation78. Weakly basic drugs such as doxorubicin are ionized extracellularly. Moreover, the small amount of weakly basic drugs that can enter the cells becomes trapped within acidic vesiclesCitation75 (). Inhibition of V-ATPase serves to re-sensitize tumor cells to such weakly basic drugsCitation79–81. V-ATPase inhibitors include proton-pump inhibitor (e.g. lansoprazole), bafilomycin, concanamycin, the benzolactoneenamides salicylihalamides, and lobotamides, and, more recently, the macrolide lactams chondropsin and poecillastrinCitation76,80,82–84.
Carbonic anhydrases
Carbonic anhydrases 9 (CAIX)
Carbonic anhydrases (CAs) are a large family of zinc metalloenzymes that catalyze the reversible hydration of carbon dioxide. They participate in a variety of biological processes, including respiration, calcification, acid–base balance, bone resorption, and the formation of aqueous humor, cerebrospinal fluid, saliva, and gastric acid.
Carbonic anhydrase 9 (CA9/CAIX) is an enzyme that in human is encoded by the CA9 geneCitation85. It has also been linked to malignant transformation and hypoxia in various cancersCitation86,Citation87. CA9 also supports tumor growth and survival under normoxia tooCitation88. CA9 is a cancer-associated transmembrane enzyme, involving in pH regulation and ion transportCitation89. The CA9 expression is driven by hypoxiaCitation90, and it is overexpressed preferentially at the edge of the tumorCitation90 while other did observe it at the core of tumor (necrotic region)Citation91. CA9 is correlated with tumor cell invasion/migrationCitation90,Citation92; it is associated with poor prognosis of the tumorCitation93,Citation94. Also, CA9 is associated with resistance to chemotherapyCitation95–99.
Carbonic anhydrase 12 (CAXI)
Carbonic anhydrase 12 is an enzyme that in humans is encoded by the CA12 geneCitation100. CA12 is associated with poor prognosisCitation101 also responsible for tumor invasionCitation102 and associated with poor prognosisCitation103 while other data suggested CA12 is related to good prognosisCitation104,Citation105. These data collectively reveal that CA12 overexpressed preferentially at the edge of the tumor (distally from necrotic regions). Our data demonstrate that CA9 is correlated with P-gp overexpression (unpublished DATA), and hence, it is correlated with cancer chemotherapy resistance.
Carbonic anhydrase inhibition to alter tumor pH gradient becomes a promising strategy for treating cancerCitation89,Citation106. Carbonic anhydrase inhibitors are divided into four groups: inorganic anions, sulfonamide-based compounds (sulphonamides, sulfanilamides, sulphamates, and their derivatives), phenols, and coumarinsCitation105. A sulfonamide CA9 inhibitor (SLC-0111) is presently in Phase I clinical trialsCitation107. It has been shown that simultaneous inhibition of both CA9 and CA12 has a greater impact than inhibition of each individually or even inhibition of HIF-αCitation105. Indisulam is an example of nonselective CAs inhibitor where it inhibits both CA9 and CA12Citation108.
Impact of these channels in creating the tumor microenvironment
The tumor microenvironment consists of blood vesselsCitation109, immune cellsCitation110,Citation111, fibroblastsCitation112,Citation113 other cells, signaling moleculesCitation114–116, and the extracellular matrix (ECM)Citation117. In tumorigenesis, all of these components are working together in this unusual acidic mediumCitation50. Although some studies suggest that these ion channels mediate carcinogenesis by creating a hostile and sanctuary environmentCitation29,Citation39,Citation118, other suggests that these ion channels are an adaptation to the intolerable acidity created within cancer cellsCitation119. Nevertheless, the presence of this microenvironment has evolutionary advantages by reducing the stress e.g. defeating chemotherapy i.e. chemotherapy resistance.
Resistance
The prevailing medical dogma of cancer chemotherapy resistance is the overexpression of P-gp (MDR)Citation120–124 which is a very narrow perspective. Cancer chemotherapy resistance is a multifactorial phenomenon that can occur at multiple levels (local, regional, and systemic)Citation125. The tumor microenvironment is considered at a regional level that alters efficacy of cancer chemotherapy by:
Tumor vascularization: The tumor is like a fetus that grows outside the uterus. The placenta provides the fetus with nutrients and eliminates wastes; tumor vascularization serves as the “tumor placenta” supporting tumor growth by supplying nutrientsCitation126 and removing waste metabolitesCitation127. However, tumor vascularization differs from the physiology of the placenta in that tumor vascularization occurs as a response to hypoxia that may be regarded as detrimental to tumor progression. Inhibition of tumor angiogenesis would lead to the selective survival of hypoxic cancer cells, and it is these hypoxic cells at the center of tumor masses that are the most difficult to target with chemotherapeutic drugs and thus the larger the number of hypoxic tumor cells the worse the prognosis. Limiting the vascularization that occurs within tumors also restricts the perfusion of the cytotoxic agents as these are unable to perfuse the hypoxic central region of the tumor and can only penetrate those cells at the periphery of the tumor near to a blood supply atCitation128,Citation129.
Physicochemical properties of drugs: The tumor microenvironment alters the physicochemical properties of proton transporterCitation130,Citation131. Extrusion of protons into the extracellular milieu results in the generation of an acidic pHCitation1,Citation50. This extracellular acidity leads to protonation of weakly basic drugs as doxorubicin, decreasing their partitioning inside cellsCitation42 (). Thus, the tumor microenvironment creates another equilibrium as far as drug uptake is involved. Finally, it was also suggested that membrane stiffness increases due to the cytosolic alkaline pH reducing the ability of any chemotherapeutic agent to enter cancer cellsCitation130–132. Finally, the changes in membrane stiffness increase membrane recycling augmenting drug trapping inside acidified compartmentsCitation133–135.
Conclusion
Malignant transformation is a highly organized process accompanied by (i) reprogramming of the cellular metabolism that creates a unique pH gradient and (ii) the overexpression of several proton transporters and ion channels (e.g. MCTs, NHE1, V-ATPase, CA9, and CA12) that maintain this pH gradient as well as promote cancer cell survival. This acidic tumor microenvironment suppresses the growth of normal cells, supports cancer cell growth and migration, and blunts the response of the immune system. The acidic tumor environment also mediates microenvironmental resistance by either affecting drug permeability or altering the physicochemical properties of the chemotherapeutic agent at the site of the tumor. The cell transporters and ion channels represent the core of this complex and coordinated system. Therefore, the targeting of these transporters and ion channels will represent a new class of potential anticancer treatments and combination strategies that contributes to the war against cancer by controlling the progression of the disease, improving the tumor prognosis, improving the quality of life as well as increasing the survival rate of the patients, and finally decreasing the medicines cost.
Acknowledgements
The author thank Professors Salvador Harguindey, Stephan Joel Reshkin, Cyril Rauch, and Megan Walsh for the excellent discussion on the current topic.
Declaration of interest
The author reports no conflicts of interest. The author alone is responsible for the content and writing of this article.
References
- Alfarouk KO, Shayoub MEAA, Muddathir AK, et al Evolution of tumor metabolism might reflect carcinogenesis as a reverse evolution process (dismantling of multicellularity). Cancers (Basel) 2011;3:3002–17
- Alfarouk KO, Verduzco D, Rauch C, et al Glycolysis, tumor metabolism, cancer growth and dissemination. A new pH-based etiopathogenic perspective and therapeutic approach to an old cancer question. Oncoscience 2014;1:777–802
- Moldovan L, Mythreye K, Goldschmidt-Clermont PJ, Satterwhite LL. Reactive oxygen species in vascular endothelial cell motility. Roles of NAD(P)H oxidase and Rac1. Cardiovasc Res 2006;71:236–46
- Kim J-S, Huang TY, Bokoch GM. Reactive oxygen species regulate a slingshot-cofilin activation pathway. Mol Biol Cell 2009;20:2650–60
- Luanpitpong S, Talbott SJ, Rojanasakul Y, et al Regulation of lung cancer cell migration and invasion by reactive oxygen species and caveolin-1. J Biol Chem 2010;285:38832–40
- Hurd TR, DeGennaro M, Lehmann R. Redox regulation of cell migration and adhesion. Trends Cell Biol 2012;22:107–15
- Gatenby RA, Gillies RJ. Why do cancers have high aerobic glycolysis? Nat Rev Cancer 2004;4:891–9
- Kelloff GJ, Hoffman JM, Johnson B, et al Progress and promise of FDG-PET imaging for cancer patient management and oncologic drug development. Clin Cancer Res 2005;11:2785–808
- Nijsten MWN, van Dam GM. Hypothesis: using the Warburg effect against cancer by reducing glucose and providing lactate. Med Hypotheses 2009;73:48–51
- Bailey KM, Wojtkowiak JW, Hashim AI, Gillies RJ. Targeting the metabolic microenvironment of tumors. Adv Pharmacol 2012;65:63–107
- Silva AS, Yunes JA, Gillies RJ, et al The potential role of systemic buffers in reducing intratumoral extracellular pH and acid-mediated invasion. Cancer Res 2009;69:2677–84
- Martin NK, Robey IF, Gaffney EA, et al Predicting the safety and efficacy of buffer therapy to raise tumour pH: an integrative modelling study. Br J Cancer 2012;106:1280–7
- Putignani L, Raffa S, Pescosolido R, et al Mitochondrion Preliminary evidences on mitochondrial injury and impaired oxidative metabolism in breast cancer. Mitochondrion 2012;12:363–9
- Cza as rnecka AM, Gammazza AM, Felice VD, et al Cancer as a “mitochondriopathy”. J Cancer Mol 2007;3:71–9
- Czarnecka AM, Czarnecki JS, Kukwa W, et al Molecular oncology focus – is carcinogenesis a “mitochondriopathy”? J Biomed Sci 2010;17:31
- Higuchi M. Regulation of mitochondrial DNA content and cancer. Mitochondrion 2007;7:53–7
- Wen S, Zhang F, Feng S. Decreased copy number of mitochondrial DNA: a potential diagnostic criterion for gastric cancer. Oncol Lett 2013;6:1098–102
- Thyagarajan B, Wang R, Nelson H, et al Mitochondrial DNA copy number is associated with breast cancer risk. PLoS One 2013;8:e65968
- Cui H, Huang P, Wang Z, et al Association of decreased mitochondrial DNA content with the progression of colorectal cancer. BMC Cancer 2013;13:110
- Garber K. Energy boost: the Warburg effect returns in a new theory of cancer. J Natl Cancer Inst 2004;96:1805–6
- Vander Heiden MG, Cantley LC, Thompson CB. Understanding the Warburg effect: the metabolic requirements of cell proliferation. Science 2009;324:1029–33
- DeBerardinis RJ, Thompson CB. Cellular metabolism and disease: what do metabolic outliers teach us? Cell 2012;148:1132–44
- Reed JC, Jurgensmeier JM, Matsuyama S. Bcl-2 family proteins and mitochondria. Biochim Biophys Acta 1998;1366:127–37
- Green DR, Reed JC. Mitochondria and apoptosis. Science 1998;281:1309–12
- Wang C, Youle RJ. The role of mitochondria in apoptosis. Annu Rev Genet 2009;43:95–118
- Rehman J, Zhang HJ, Toth PT, et al Inhibition of mitochondrial fission prevents cell cycle progression in lung cancer. FASEB J 2012;26:2175–86
- Westermann B. Mitochondrial fusion and fission in cell life and death. Nat Rev Mol Cell Biol 2010;11:872–84
- Chen H, Chan DC. Mitochondrial dynamics – fusion, fission, movement, and mitophagy – in neurodegenerative diseases. Hum Mol Genet 2009;18:R169–76
- Reshkin SJ, Bellizzi A, Caldeira S, et al Na+/H + exchanger-dependent intracellular alkalinization is an early event in malignant transformation and plays an essential role in the development of subsequent transformation-associated phenotypes. FASEB J 2000;14:2185–97
- Gatenby RA, Smallbone K, Maini PK, et al Cellular adaptations to hypoxia and acidosis during somatic evolution of breast cancer. Br J Cancer 2007;97:646–53
- Tse CM, Ma AI, Yang VW, et al Molecular cloning and expression of a cDNA encoding the rabbit ileal villus cell basolateral membrane Na+/H + exchanger. EMBO J 1991;10:1957–67
- Tse CM, Levine SA, Yun CH, et al Cloning and expression of a rabbit cDNA encoding a serum-activated ethylisopropylamiloride-resistant epithelial Na+/H + exchanger isoform (NHE-2). J Biol Chem 1993;268:11917–24
- Fliegel L, Dyck JR, Wang H, et al Cloning and analysis of the human myocardial Na+/H + exchanger. Mol Cell Biochem 1993;125:137–43
- Denker SP, Huang DC, Orlowski J, et al Direct binding of the Na-H exchanger NHE1 to ERM proteins regulates the cortical cytoskeleton and cell shape independently of H(+) translocation. Mol Cell 2000;6:1425–36
- Torres-López JE, Guzmán-Priego CG, Rocha-González HI, Granados-Soto V. Role of NHE1 in nociception. Pain Res Treat 2013;2013:217864
- Fliegel L. The Na+/H + exchanger isoform 1. Int J Biochem Cell Biol 2005;37:33–7
- Provost JJ, Wallert Ma. Inside out: targeting NHE1 as an intracellular and extracellular regulator of cancer progression. Chem Biol Drug Des 2013;81:85–101
- Schwab A. Function and spatial distribution of ion channels and transporters in cell migration. Am J Physiol Renal Physiol 2001;280:F739–47
- Reshkin SJ, Cardone RA, Harguindey S. Na+-H + exchanger, pH regulation and cancer. Recent Pat Anticancer Drug Discov 2013;8:85–99
- Raghunand N, He X, van Sluis R, et al Enhancement of chemotherapy by manipulation of tumour pH. Br J Cancer 1999;80:1005–11
- Raghunand N, Mahoney BP, Gillies RJ. Tumor acidity, ion trapping and chemotherapeutics. II. pH-dependent partition coefficients predict importance of ion trapping on pharmacokinetics of weakly basic chemotherapeutic agents. Biochem Pharmacol 2003;66:1219–29
- Mahoney BP, Raghunand N, Baggett B, Gillies RJ. Tumor acidity, ion trapping and chemotherapeutics. I. Acid pH affects the distribution of chemotherapeutic agents in vitro. Biochem Pharmacol 2003;66:1207–18
- Harguindey S, Arranz JL, Polo Orozco JD, et al Cariporide and other new and powerful NHE1 inhibitors as potentially selective anticancer drugs-an integral molecular/biochemical/metabolic/clinical approach after one hundred years of cancer research. J Transl Med 2013;11:282
- Harguindey S, Orive G, Luis Pedraz J, et al The role of pH dynamics and the Na+/H + antiporter in the etiopathogenesis and treatment of cancer. Two faces of the same coin-one single nature. Biochim Biophys Acta 2005;1756:1–24
- Harguindey S, Arranz JL, Wahl ML, et al Proton transport inhibitors as potentially selective anticancer drugs. Anticancer Res 2009;29:2127–36
- Pinheiro C, Longatto-Filho A, Azevedo-Silva J, et al Role of monocarboxylate transporters in human cancers: state of the art. J Bioenerg Biomembr 2012;44:127–39
- Adijanto J, Philp NJ. The SLC16A family of monocarboxylate transporters (MCTs)-physiology and function in cellular metabolism, pH homeostasis, and fluid transport. Curr Top Membr 2012;70:275–311
- Poole RC, Halestrap AP. Transport of lactate and other monocarboxylates across mammalian plasma membranes. Am J Physiol 1993;264:C761–82
- Garcia CK, Goldstein JL, Pathak RK, et al Molecular characterization of a membrane transporter for lactate, pyruvate, and other monocarboxylates: implications for the Cori cycle. Cell 1994;76:865–73
- Alfarouk KO, Muddathir AK, Shayoub MEA. Tumor acidity as evolutionary spite. Cancers (Basel) 2011;3:408–14
- Brooks GA. Cell-cell and intracellular lactate shuttles. J Physiol (Lond) 2009;587:5591–600
- Hirschhaeuser F, Sattler UGA, Mueller-Klieser W. Lactate: a metabolic key player in cancer. Cancer Res 2011;71:6921–5
- Dhup S, Dadhich RK, Porporato PE, Sonveaux P. Multiple biological activities of lactic acid in cancer: influences on tumor growth, angiogenesis and metastasis. Curr Pharm Des 2012;18:1319–30
- Jóhannsson E, Nagelhus EA, McCullagh KJ, et al Cellular and subcellular expression of the monocarboxylate transporter MCT1 in rat heart. A high-resolution immunogold analysis. Circ Res 1997;80:400–7
- Gray MW, Burger G, Lang BF. Mitochondrial evolution. Science 1999;283:1476–81
- Andersson SGE, Karlberg O, Canbäck B, Kurland CG. On the origin of mitochondria: a genomics perspective. Philos Trans R Soc Lond B Biol Sci 2003;358:165–77
- Gray MW, Burger G, Lang BF. The origin and early evolution of mitochondria. Genome Biol 2001;2:reviews1018.1–reviews1018.5
- Hashimoto T, Hussien R, Brooks GA. Colocalization of MCT1, CD147, and LDH in mitochondrial inner membrane of L6 muscle cells: evidence of a mitochondrial lactate oxidation complex. Am J Physiol Endocrinol Metab 2006;290:E1237–44
- Gabaldón T, Snel B, van Zimmeren F, et al Origin and evolution of the peroxisomal proteome. Biol Direct 2006;1:8
- McClelland GB, Khanna S, González GF, et al Peroxisomal membrane monocarboxylate transporters: evidence for a redox shuttle system? Biochem Biophys Res Commun 2003;304:130–5
- Zha S, Ferdinandusse S, Hicks JL, et al Peroxisomal branched chain fatty acid beta-oxidation pathway is upregulated in prostate cancer. Prostate 2005;63:316–23
- Izumi H, Torigoe T, Ishiguchi H, et al Cellular pH regulators: potentially promising molecular targets for cancer chemotherapy. Cancer Treat Rev 2003;29:541–9
- De Saedeleer CJ, Porporato PE, Copetti T, et al Glucose deprivation increases monocarboxylate transporter 1 (MCT1) expression and MCT1-dependent tumor cell migration. Oncogene 2014;33:4060–8
- Zhao Z, Wu M-S, Zou C, et al Downregulation of MCT1 inhibits tumor growth, metastasis and enhances chemotherapeutic efficacy in osteosarcoma through regulation of the NF-κB pathway. Cancer Lett 2014;342:150–8
- Pinheiro C, Sousa B, Albergaria A, et al GLUT1 and CAIX expression profiles in breast cancer correlate with adverse prognostic factors and MCT1 overexpression. Histol Histopathol 2011;26:1279–86
- Curry JM, Tuluc M, Whitaker-Menezes D, et al Cancer metabolism, stemness and tumor recurrence: MCT1 and MCT4 are functional biomarkers of metabolic symbiosis in head and neck cancer. Cell Cycle 2013;12:1371–84
- Shih H-J, Chen H-H, Chen Y-A, et al Targeting MCT-1 oncogene inhibits Shc pathway and xenograft tumorigenicity. Oncotarget 2012;3:1401–15
- Birsoy K, Wang T, Possemato R, et al MCT1-mediated transport of a toxic molecule is an effective strategy for targeting glycolytic tumors. Nat Genet 2013;45:104–8
- Sonveaux P, Copetti T, De Saedeleer CJ, et al Targeting the lactate transporter MCT1 in endothelial cells inhibits lactate-induced HIF-1 activation and tumor angiogenesis. PLoS One 2012;7:e33418
- Ohsumi Y, Anraku Y. Active transport of basic amino acids driven by a proton motive force in vacuolar membrane vesicles of Saccharomyces cerevisiae. J Biol Chem 1981;256:2079–82
- Nelson N. Evolution of organellar proton-ATPases. Biochim Biophys Acta 1992;1100:109–24
- Nelson N. Organellar proton-ATPases. Curr Opin Cell Biol 1992;4:654–60
- Nishi T, Forgac M. The vacuolar (H+)-ATPases-nature’s most versatile proton pumps. Nat Rev Mol Cell Biol 2002;3:94–103
- Merzendorfer H, Gräf R, Huss M, et al Regulation of proton-translocating V-ATPases. J Exp Biol 1997;200:225–35
- Fais S, De Milito A, You H, Qin W. Targeting vacuolar H+-ATPases as a new strategy against cancer. Cancer Res 2007;67:10627–30
- Sennoune SR, Bakunts K, Martínez GM, et al Vacuolar H+-ATPase in human breast cancer cells with distinct metastatic potential: distribution and functional activity. Am J Physiol Cell Physiol 2004;286:C1443–52
- Martínez-Zaguilán R, Raghunand N, Lynch RM, et al pH and drug resistance. I. Functional expression of plasmalemmal V-type H+-ATPase in drug-resistant human breast carcinoma cell lines. Biochem Pharmacol 1999;57:1037–46
- Lu Q, Lu S, Huang L, et al The expression of V-ATPase is associated with drug resistance and pathology of non-small-cell lung cancer. Diagn Pathol 2013;8:145
- Wang B-Y, Zhang J, Wang J-L, et al Intermittent high dose proton pump inhibitor enhances the antitumor effects of chemotherapy in metastatic breast cancer. J Exp Clin Cancer Res 2015;34:85
- Spugnini EP, Buglioni S, Carocci F, et al High dose lansoprazole combined with metronomic chemotherapy: a phase I/II study in companion animals with spontaneously occurring tumors. J Transl Med 2014;12:225
- Ferrari S, Perut F, Fagioli F, et al Proton pump inhibitor chemosensitization in human osteosarcoma: from the bench to the patients’ bed. J Transl Med 2013;11:268
- Spugnini EP, Baldi A, Buglioni S, et al Lansoprazole as a rescue agent in chemoresistant tumors: a phase I/II study in companion animals with spontaneously occurring tumors. J Transl Med 2011;9:221
- Lugini L, Federici C, Borghi M, et al Proton pump inhibitors while belonging to the same family of generic drugs show different anti-tumor effect. J Enzyme Inhib Med Chem 2015;30:1–8
- Papagerakis S, Bellile E, Peterson LA, et al Proton pump inhibitors and histamine 2 blockers are associated with improved overall survival in patients with head and neck squamous carcinoma. Cancer Prev Res (Phila) 2014;7:1258–69
- Opavský R, Pastoreková S, Zelník V, et al Human MN/CA9 gene, a novel member of the carbonic anhydrase family: structure and exon to protein domain relationships. Genomics 1996;33:480–7
- Chen J, Röcken C, Hoffmann J, et al Expression of carbonic anhydrase 9 at the invasion front of gastric cancers. Gut 2005;54:920–7
- Swietach P, Vaughan-Jones RD, Harris AL. Regulation of tumor pH and the role of carbonic anhydrase 9. Cancer Metastasis Rev 2007;26:299–310
- Robertson N, Potter C, Harris AL. Role of carbonic anhydrase IX in human tumor cell growth, survival, and invasion. Cancer Res 2004;64:6160–5
- Supuran CT. Carbonic anhydrases: novel therapeutic applications for inhibitors and activators. Nat Rev Drug Discov 2008;7:168–81
- Svastova E, Witarski W, Csaderova L, et al Carbonic anhydrase IX interacts with bicarbonate transporters in lamellipodia and increases cell migration via its catalytic domain. J Biol Chem 2012;287:3392–402
- Ivanov S, Liao S-Y, Ivanova A, et al Expression of hypoxia-inducible cell-surface transmembrane carbonic anhydrases in human cancer. Am J Pathol 2001;158:905–19
- Yang J-S, Lin C-W, Chuang C-Y, et al Carbonic anhydrase IX overexpression regulates the migration and progression in oral squamous cell carcinoma. Tumour Biol 2015;36:9517–24
- Lou Y, McDonald PC, Oloumi A, et al Targeting tumor hypoxia: suppression of breast tumor growth and metastasis by novel carbonic anhydrase IX inhibitors. Cancer Res 2011;71:3364–76
- McDonald PC, Winum J-Y, Supuran CT, Dedhar S. Recent developments in targeting carbonic anhydrase IX for cancer therapeutics. Oncotarget 2012;3:84–97
- Tan EY, Yan M, Campo L, et al The key hypoxia regulated gene CAIX is upregulated in basal-like breast tumours and is associated with resistance to chemotherapy. Br J Cancer 2009;100:405–11
- Said HM, Supuran CT, Hageman C, et al Modulation of carbonic anhydrase 9 (CA9) in human brain cancer. Curr Pharm Des 2010;16:3288–99
- Betof AS, Rabbani ZN, Hardee ME, et al Carbonic anhydrase IX is a predictive marker of doxorubicin resistance in early-stage breast cancer independent of HER2 and TOP2A amplification. Br J Cancer 2012;106:916–22
- Aomatsu N, Yashiro M, Kashiwagi S, et al Carbonic anhydrase 9 is associated with chemosensitivity and prognosis in breast cancer patients treated with taxane and anthracycline. BMC Cancer 2014;14:400
- Zheng G, Peng C, Jia X, et al ZEB1 transcriptionally regulated carbonic anhydrase 9 mediates the chemoresistance of tongue cancer via maintaining intracellular pH. Mol Cancer 2015;14:84
- Türeci O, Sahin U, Vollmar E, et al Human carbonic anhydrase XII: cDNA cloning, expression, and chromosomal localization of a carbonic anhydrase gene that is overexpressed in some renal cell cancers. Proc Natl Acad Sci USA 1998;95:7608–13
- Loncaster JA, Harris AL, Davidson SE, et al Carbonic anhydrase (CA IX) expression, a potential new intrinsic marker of hypoxia: correlations with tumor oxygen measurements and prognosis in locally advanced carcinoma of the cervix. Cancer Res 2001;61:6394–9
- Hsieh M-J, Chen K-S, Chiou H-L, Hsieh Y-S. Carbonic anhydrase XII promotes invasion and migration ability of MDA-MB-231 breast cancer cells through the p38 MAPK signaling pathway. Eur J Cell Biol 2010;89:598–606
- Chien M-H, Ying T-H, Hsieh Y-H, et al Tumor-associated carbonic anhydrase XII is linked to the growth of primary oral squamous cell carcinoma and its poor prognosis. Oral Oncol 2012;48:417–23
- Watson PH, Chia SK, Wykoff CC, et al Carbonic anhydrase XII is a marker of good prognosis in invasive breast carcinoma. Br J Cancer 2003;88:1065–70
- Tafreshi NK, Lloyd MC, Bui MM, et al Carbonic anhydrase IX as an imaging and therapeutic target for tumors and metastases. Subcell Biochem 2014;75:221–54
- Neri D, Supuran CT. Interfering with pH regulation in tumours as a therapeutic strategy. Nat Rev Drug Discov 2011;10:767–77
- Grandane A, Tanc M, Di Cesare Mannelli L, et al 6-Substituted sulfocoumarins are selective carbonic anhdydrase IX and XII inhibitors with significant cytotoxicity against colorectal cancer cells. J Med Chem 2015;58:3975–83
- Teixeira SA, Pezuk JA, Brassesco MS, et al Abstract C292: inhibition of carbonic anhydrase (9 and 12) decreases cell proliferation and gene expression in human glioblastoma cell. Mol Cancer Ther 2014;12:C292
- Balkwill FR, Capasso M, Hagemann T. The tumor microenvironment at a glance. J Cell Sci 2012;125:5591–6
- Gajewski TF, Schreiber H, Fu Y-X. Innate and adaptive immune cells in the tumor microenvironment. Nat Immunol 2013;14:1014–22
- Whiteside TL. The tumor microenvironment and its role in promoting tumor growth. Oncogene 2008;27:5904–12
- Xing F, Saidou J, Watabe K. Cancer associated fibroblasts (CAFs) in tumor microenvironment. Front Biosci (Landmark Ed) 2010;15:166–79
- Kharaishvili G, Simkova D, Bouchalova K, et al The role of cancer-associated fibroblasts, solid stress and other microenvironmental factors in tumor progression and therapy resistance. Cancer Cell Int 2014;14:41
- Gelmon KA, Eisenhauer EA, Harris AL, et al Anticancer agents targeting signaling molecules and cancer cell environment: challenges for drug development? J Natl Cancer Inst 1999;91:1281–7
- Macheda ML, Stacker SA. Importance of Wnt signaling in the tumor stroma microenvironment. Curr Cancer Drug Targets 2008;8:454–65
- Bierie B, Moses HL. Tumour microenvironment: TGFbeta: the molecular Jekyll and Hyde of cancer. Nat Rev Cancer 2006;6:506–20
- Lu P, Weaver VM, Werb Z. The extracellular matrix: a dynamic niche in cancer progression. J Cell Biol 2012;196:395–406
- Huber V, De Milito A, Harguindey S, et al Proton dynamics in cancer. J Transl Med 2010;8:57
- Xu K, Mao X, Mehta M, et al Elucidation of how cancer cells avoid acidosis through comparative transcriptomic data analysis. PLoS One 2013;8:e71177
- Szakács G, Paterson JK, Ludwig JA, et al Targeting multidrug resistance in cancer. Nat Rev Drug Discov 2006;5:219–34
- Persidis A. Cancer multidrug resistance. Nat Biotechnol 1999;17:94–5
- Bao L, Haque A, Jackson K, et al Increased expression of P-glycoprotein is associated with doxorubicin chemoresistance in the metastatic 4T1 breast cancer model. Am J Pathol 2011;178:838–52
- Wu H, Hait WN, Yang J-M. Small interfering RNA-induced suppression of MDR1 (P-glycoprotein) restores sensitivity to multidrug-resistant cancer cells. Cancer Res 2003;63:1515–19
- Donnenberg VS, Donnenberg AD. Multiple drug resistance in cancer revisited: the cancer stem cell hypothesis. J Clin Pharmacol 2005;45:872–7
- Alfarouk KO, Stock C-M, Taylor S, et al Resistance to cancer chemotherapy: failure in drug response from ADME to P-gp. Cancer Cell Int 2015;15:71
- Siemann DW, ed. Tumor vasculature: a target for anticancer therapies. In: Vascular-targeted therapies in oncology. Chichester: John Wiley & Sons, Ltd; 2006:1–8
- Alfarouk KO, Ibrahim ME, Gatenby RA, Brown JS. Riparian ecosystems in human cancers. Evol Appl 2013;6:46–53
- Lloyd MC, Alfarouk KO, Verduzco D, et al Vascular measurements correlate with estrogen receptor status. BMC Cancer 2014;14:279
- Ma J, Waxman DJ. Combination of antiangiogenesis with chemotherapy for more effective cancer treatment. Mol Cancer Ther 2008;7:3670–84
- Daniel C, Bell C, Burton C, et al The role of proton dynamics in the development and maintenance of multidrug resistance in cancer. Biochim Biophys Acta 2013;1832:606–17
- Rauch C. On the relationship between drug’s size, cell membrane mechanical properties and high levels of multi drug resistance: a comparison to published data. Eur Biophys J 2009;38:537–46
- Rauch C. Toward a mechanical control of drug delivery. On the relationship between Lipinski’s 2nd rule and cytosolic pH changes in doxorubicin resistance levels in cancer cells: a comparison to published data. Eur Biophys J 2009;38:829–46
- Raghunand N, Gillies RJ. pH and drug resistance in tumors. Drug Resist Updat 2000;3:39–47
- Raghunand N, Martínez-Zaguilán R, Wright SH, Gillies RJ. pH and drug resistance. II. Turnover of acidic vesicles and resistance to weakly basic chemotherapeutic drugs. Biochem Pharmacol 1999;57:1047–58
- Song CW, Griffin R, Park HJ. Influence of tumor pH on therapeutic response. In: Teicher B, ed. Cancer drug discovery and development: cancer drug Resistance. Totowa (NJ): Humana Press Inc.; 2006:21–43
- Noël J, Pouysségur J. Hormonal regulation, pharmacology, and membrane sorting of vertebrate Na+/H + exchanger isoforms. Am J Physiol 1995;268:C283–96
- Bookstein C, Xie Y, Rabenau K, et al Tissue distribution of Na+/H + exchanger isoforms NHE2 and NHE4 in rat intestine and kidney. Am J Physiol 1997;273:C1496–505
- Biemesderfer D, Pizzonia J, Abu-Alfa A, et al NHE3: a Na+/H + exchanger isoform of renal brush border. Am J Physiol 1993;265:F736–42
- Orlowski J, Kandasamy RA, Shull GE. Molecular cloning of putative members of the Na/H exchanger gene family. cDNA cloning, deduced amino acid sequence, and mRNA tissue expression of the rat Na/H exchanger NHE-1 and two structurally related proteins. J Biol Chem 1992;267:9331–9
- Bookstein C, Musch MW, DePaoli A, et al A unique sodium-hydrogen exchange isoform (NHE-4) of the inner medulla of the rat kidney is induced by hyperosmolarity. J Biol Chem 1994;269:29704–9
- Xinhan L, Matsushita M, Numaza M, et al Na+/H + exchanger isoform 6 (NHE6/SLC9A6) is involved in clathrin-dependent endocytosis of transferrin. Am J Physiol Cell Physiol 2011;301:C1431–44
- Schwede M, Garbett K, Mirnics K, et al Genes for endosomal NHE6 and NHE9 are misregulated in autism brains. Mol Psychiatry 2013;19:277–9
- Onishi I, Lin PJC, Numata Y, et al Organellar (Na+, K+)/H + exchanger NHE7 regulates cell adhesion, invasion and anchorage-independent growth of breast cancer MDA-MB-231 cells. Oncol Rep 2012;27:311–17
- Johnson LR, Ghishan FK, Kaunitz JD, et al Physiology of the gastrointestinal tract. Chapter 66. Amsterdam: Elsevier; 2012:1799. ISBN: 978-0-12-382026-6
- Gawenis LR, Greeb JM, Prasad V, et al Impaired gastric acid secretion in mice with a targeted disruption of the NHE4 Na+/H + exchanger. J Biol Chem 2005;280:12781–9
- Nakamura N, Tanaka S, Teko Y, et al Four Na+/H + exchanger isoforms are distributed to Golgi and post-Golgi compartments and are involved in organelle pH regulation. J Biol Chem 2005;280:1561–72
- Helbig G, Christopherson KW. Bhat-Nakshatri., et al NF-kappaB promotes breast cancer cell migration and metastasis by inducing the expression of the chemokine receptor CXCR4. J Biol Chem 2003;278:21631–8
- Zhao M, Gao Y, Wang L, et al Overexpression of integrin-linked kinase promotes lung cancer cell migration and invasion via NF-κB-mediated upregulation of matrix metalloproteinase-9. Int J Med Sci 2013;10:995–1002
- Karin M. NF-kappaB as a critical link between inflammation and cancer. Cold Spring Harb Perspect Biol 2009;1:a000141
- Samuvel DJ, Sundararaj KP, Nareika A, et al Lactate boosts TLR4 signaling and NF-kappaB pathway-mediated gene transcription in macrophages via monocarboxylate transporters and MD-2 up-regulation. J Immunol 2009;182:2476–84
- Karin M. Nuclear factor-kappaB in cancer development and progression. Nature 2006;441:431–6
- Liu F, Bardhan K, Yang D, et al NF-κB directly regulates Fas transcription to modulate Fas-mediated apoptosis and tumor suppression. J Biol Chem 2012;287:25530–40
- Parfentjev IA, Suntzeff VD, Devrient WK. The influence of various preparations of lactic acid and sugars on the growth of transplanted tumors: II. Mouse Sarcoma 180. Am J Cancer 1934;20:117–36
- Lee Y-J, Kang I-J, Bünger R, et al Mechanisms of pyruvate inhibition of oxidant-induced apoptosis in human endothelial cells. Microvasc Res 2003;66:91–101