Abstract
Novel series of niacin esters of chalcones 2, 4 and 6 were designed as antineoplastic agents that have the potential to release the chemoprotectant niacin. These enones are cytotoxic to human CD4+ T-lymphocyte Molt 4/C8 and CEM and murine leukemia L1210 cells. Quantitative structure–activity relationship (QSAR) studies of the biodata in series 4 revealed that cytotoxic potency was enhanced by placing electron-repelling groups in one of the aryl rings. The compounds are lethal to HL-60, HSC-2, HSC-3 and HSC-4 neoplasms but less toxic to nonmalignant hepatocyte growth factor, hematopoietic progenitor cell and human periodontal ligament fibroblast cells. Hence, the compounds display tumor-selective toxicity. These chalcones are well tolerated in mice and no overt toxicity was noted. The results establish that in general the compounds in series 2, 4 and 6 have positive characteristics which warrant further studies.
Introduction
The principal aims of these laboratories are the design and syntheses of conjugated unsaturated ketones as candidate antineoplastic agents. These compounds react either exclusively or preferentially with thiols rather than amino and hydroxyl groupsCitation1,Citation2. Hence, they should avoid interactions with nucleic acids and thus be devoid of the genotoxic side effects displayed by a number of contemporary anticancer drugsCitation3.
One of the problems of anticancer drug therapy is that it may lead to a deficiency of niacin (vitamin B3, nicotinic acid)Citation4 which in turn can cause such adverse clinical conditions as dermatitisCitation5. Two of the difficulties of administering niacin to counteract this problem are its very short half-life in humans, and in most cases, niacin leads to acute flushing of the face and neckCitation6. However, greater tolerance to niacin may be possible if a gradual release of this compound takes place. Hence, the decision was made to prepare some representative compounds that have the potential to liberate niacin and demonstrate cytotoxic properties (Scheme 1).
The design of the compounds in Scheme 1 was based on the following considerations. (1) Niacin has a polar carboxy group that will impede penetration of neoplasms. Hence, the formulation of niacin as an ester was suggested. (2) An important issue is the choice of the potential cytotoxins to which niacin should be attached. The chalcone motif was selected since a number of these compounds have cytotoxic propertiesCitation7 and they are often well tolerated in vivoCitation8. (3) The aryl substituents in ring A of series 2 and 4 were chosen in view of their differing electronic properties having Hammett σ values ranging from −0.27 in 2c and 4c to 0.78 in 2e and 4eCitation9. These aryl groups are predicted to influence the rates of both reactions of the compounds with cellular thiols and the release of niacin. (4) In the case of the bisester 6, the ester groups are in different locations that may lead to a prolonged release of niacin (Scheme 1).
The aims of the present investigation are threefold, (i) to assess antineoplastic properties of the compounds in series 2, 4 and 6 in order to identify lead compounds for further development (ii) to evaluate selective cytotoxic properties of these molecules toward neoplasms compared to normal cells and (iii) to estimate in vivo toxicity of these compounds in mice. Should these initial experiments reveal that one or more of the enones in series 2, 4 and 6 are promising lead molecules, then future studies will be performed with special reference to the extent of the release of niacin in biological matrices.
Materials and methods
Synthesis of series 2, 4 and 6
Uncorrected melting points were obtained on a Gallenkamp instrument. 1H NMR spectra were obtained using a Bruker Avance 500 MHz spectrometer which has a 5-mm BBO probe. Chemical shifts are recorded in ppm. Elemental analyses were obtained using an Elementer CHNS analyzer.
A literature procedure was followed in preparing the intermediate ketones 1, 3 and 5Citation10. In brief, equimolar quantities of the appropriate aryl aldehyde and aryl methyl ketone in ethanol were stirred at room temperature overnight in the presence of sodium hydroxide solution (series 1) or hydrogen chloride (series 3 and 5). The precipitate formed was collected and recrystallized from water–ethanol and the structures of the compounds were confirmed by 1H NMR spectroscopy.
Nicotinoyl chloride hydrochloride (0.013 mol) was added to a solution of the appropriate phenolic intermediate (0.011 mol) and triethylamine (0.026 mol) in dichloromethane (25 mL) cooled to 5 °C. Subsequently, 4-dimethylaminopyridine (0.0005 mol) in dichloromethane (25 mL) was added and the mixture was stirred at 5–7 °C for 1 h. After the temperature of the reaction mixture had risen to room temperature, stirring was continued for 4 h. The reaction mixture was washed with water (3 × 25 mL), dried over anhydrous sodium sulfate and evaporation of the solvent afforded the crude product which was crystallized from 20% aqueous ethanol to produce the compounds in series 2 and 4. Compound 6 was prepared in a similar way except the ratio of nicotinoyl chloride hydrochloride, 5 and triethylamine was 2:1:4.
(E)-3-Phenyl-1-[4-(pyrid-3-yl-carbonyloxy)phenyl]-prop-2-en-1-one, 2a
Yield =88%, M. Pt. 145 °C. 1H NMR (500 MHz, CDCl3): δ 7.32–7.42 (m, 5H), 7.46 (dd, 1H, J = 6.94, 5.04 Hz), 7.51 (d, 1H J = 15.8 Hz), 7.62 (d, 2H, J = 3.78 Hz), 7.81 (d, 1H, J = 15.8 Hz), 8.10 (d, 2H, J = 8.51 Hz), 8.43 (d, 1H, J = 7.88 Hz), 8.85 (d, 1H, J = 3.78 Hz), 9.39 (s, 1H). 13C NMR (125 MHz, CDCl3): δ 189.01, 163.32, 154.19, 153.98, 151.34, 145.11, 137.64, 134.74, 130.66, 130.21, 128.97, 128.49, 125.20, 123.55, 121.82, 121.38. Found C, 76.36; H, 4.54 N, 3.96%. Anal. (C21H15NO3) requires C, 76.58; H, 4.59; N, 4.25.
(E)-3–(4-Methylphenyl)-1-[4-(pyrid-3-yl-carbonyloxy)phenyl]-prop-2-en-1-one, 2b
Yield =89%, M. Pt. 158–159 °C. 1H NMR (500 MHz, CDCl3): δ 2.35 (s, 3H), 7.19 (d, 2H, J = 7.57 Hz), 7.35 (m, 2H, J = 8.51 Hz), 7.41–7.47 (m, 2H), 7.48 (s, 1H), 7.51 (m, 2H, J = 7.88 Hz), 7.78 (d, 1H, J = 15.8 Hz), 8.09 (d, 2H, J = 8.51 Hz), 8.42 (dd, 1H, J = 7.57, 1.58 Hz), 8.80–8.88 (m, 1H), 9.38 (s, 1H). Found C, 76.58; H, 4.96; N, 4.12%. Anal. (C22H17NO3) requires C, 76.95; H, 4.99; N, 4.08.
(E)-3–(4-Methoxyphenyl)-1-[4-(pyrid-3-yl-carbonyloxy)phenyl]-prop-2-en-1-one, 2c
Yield = 82%, M. Pt. 162–163 °C. 1H NMR (500 MHz, CDCl3): δ 3.84 (d, 3H, J = 1.26 Hz), 6.90–6.97 (m, 2H), 7.33–7.44 (m, 3H), 7.45–7.51 (m, 1H), 7.60 (d, 2H, J = 7.57 Hz), 7.80 (d, 1H, J = 15.5 Hz), 8.07–8.14 (m, 2H), 8.43–8.49 (m, 1H), 8.87 (dd, 1H, J = 3.31, 1.42 Hz), 9.40 (s, 1H). Found C, 73.33; H, 4.78; N, 3.78%. Anal. (C22H17NO4) requires C, 73.53; H, 4.77; N, 3.90.
(E)-3–(4-Chlorophenyl -1-[4-(pyrid-3-ylcarbonyloxy)phenyl]-prop-2-en-1-one, 2d
Yield = 72%, M. Pt. 183–184 °C. 1H NMR (500 MHz, CDCl3): δ 7.40 (dd, 4H, J = 8.35, 1.73 Hz), 7.47–7.54 (m, 2H), 7.58 (d, 2H, J = 8.51 Hz), 7.78 (d, 1H, J = 15.8 Hz), 8.09–8.16 (m, 2H), 8.48 (dt, 1H, J = 7.88, 1.89 Hz), 8.89 (d, 1H, J = 3.78 Hz), 9.42 (s, 1H). Found C, 69.18; H, 3.84; N, 3.80%. Anal. (C21H14ClNO3) requires C, 69.33; H, 3.88; N, 3.85.
(E)-3–(4-Nitrophenyl)-1-[4-(pyrid-3-yl-carbonyloxy)phenyl]-prop-2-en-1-one, 2e
Yield = 79%, M. Pt. 194–195 °C. 1H NMR (500 MHz, CDCl3): δ 7.42 (d, 2H, J = 8.51 Hz), 7.51 (dd, 1H, J = 7.57, 4.73 Hz), 7.65 (d, 1H, J = 15.5 Hz), 7.73–7.91 (m, 4H), 8.15 (d, 3H, J = 8.51 Hz), 8.29 (d, 3H, J = 8.51 Hz), 8.48 (d, 1H, J = 7.88 Hz), 8.89 (d, 1H, J = 3.78 Hz), 9.42 (br s, 1H). Found C, 67.27; H, 3.68; N, 7.45%. Anal. (C21H14N2O5) requires C, 67.38; H, 3.77; N, 7.48.
(E)-1-Phenyl-3-[4-(pyrid-3-yl-carbonyloxy)phenyl]-prop-2-en-1-one, 4a
Yield = 62%, M. Pt. 117–118 °C. 1H NMR (500 MHz, CDCl3): δ 7.28 (d, 2H, J = 8.51 Hz), 7.41–7.53 (m, 4H), 7.53–7.59 (m, 1H), 7.69 (m, 2H, J = 8.51 Hz), 7.78 (d, 1H, J = 15.8 Hz), 8.00 (d, 2H, J = 7.25 Hz, 8.42 (d, 1H, J = 7.88 Hz), 8.81–8.87 (m, 1H), 9.37 (s, 1H). 13C NMR (125 MHz, CDCl3): δ 190.05, 163.42, 154.00, 151.98, 143.32, 137.99, 137.60, 132.94, 132.81, 129.64, 128.60, 128.44, 125.26, 123.51, 122.33, 122.12. Found C, 76.39; H, 4.57; N, 4.21%. Anal. (C21H15NO3) requires C, 76.58; H, 4.59; N, 4.25.
(E)-1–(4-Methylphenyl)-3-[4-(pyrid-3-yl-carbonyloxy)phenyl]-prop-2-en-1-one, 4b
Yield = 84%, M. Pt. 151–152 °C. 1H NMR (500 MHz, CDCl3): δ 2.41 (s, 3H), 7.29 (d, 4H, J = 7.88 Hz), 7.42–7.48 (m, 1H), 7.51 (d, 1H, J = 15.5 Hz), 7.70 (d, 2H, J = 8.20 Hz), 7.79 (d, 1H, J = 15.8 Hz), 7.93 (d, 2H, J = 7.88 Hz), 8.44 (d, 1H, J = 7.88 Hz), 8.85 (d, 1H, J = 3.15 Hz), 9.39 (br s, 1H). Found C, 76.69; H, 5.01; N, 4.02%. Anal. (C22H17NO3) requires C, 76.95; H, 4.99; N, 4.08.
(E)-1–(4-Methoxyphenyl)-3-[4-(pyrid-3-yl-carbonyloxy)phenyl]-prop-2-en-1-one, 4c
Yield = 61%, M. Pt. 128–129 °C. 1H NMR (500 MHz, CDCl3): δ 3.87 (s, 3H), 6.97 (d, 2H, J = 8.83 Hz), 7.29 (m, 2H, J = 8.51 Hz), 7.48 (dd, 1H, J = 7.88, 5.04 Hz), 7.53 (d, 1H, J = 15.5 Hz), 7.71 (m, 2H, J = 8.51 Hz), 7.79 (d, 1H, J = 15.8 Hz), 8.03 (d, 2H, J = 8.83 Hz), 8.45 (d, 1H, J = 7.88 Hz), 8.86 (d, 1H, J = 3.78 Hz), 9.39 (s, 1H). Found C, 73.29; H, 4.80; N, 3.84%. Anal. (C22H17NO4) requires C, 73.53; H, 4.77; N, 3.90.
(E)-1–(4-Chlorophenyl)-3-[4-(pyrid-3-yl-carbonyloxy)phenyl]-prop-2-en-1-one, 4d
Yield = 82%, M. Pt. 172–173 °C. 1H NMR (500 MHz, CDCl3): δ 7.33 (m, 2H), 7.47–7.52 (m, 3H), 7.70–7.77 (m, 3H), 7.83 (d, 1H, J = 15.8 Hz), 7.95–8.01 (m, 2H), 8.69 (d, 1H, J = 8.20 Hz), 8.95 (dd, 1H, J = 5.04, 1.26 Hz), 9.44–9.49 (m, 1H). Found C, 69.18; H, 3.87; N, 3.78%. Anal. (C21H14ClNO3) requires C, 69.33; H, 3.88; N, 3.85.
(E)-1–(4-Nitrophenyl-3-[-(pyrid-3-yl-carbonyloxy)phenyl]-prop-2-en-1-one, 4e
Yield = 79%, M. Pt. 198–199 °C. 1H NMR (500 MHz, CDCl3): δ 7.35 (d, 2H), 7.49 (d, 1H, J = 15.8 Hz), 7.52–7.58 (m, 1H), 7.76 (d, 2H), 7.87 (d, 1H, J = 15.5 Hz), 8.13–8.19 (m, 2H), 8.32–8.40 (m, 2H), 8.51 (dd, 1H, J = 7.88, 1.58 Hz), 8.89 (br s, 1H), 9.42 (br s, 1H). Found C, 67.31; H, 3.74; N, 7.37%. Anal. (C32H24N2O5) requires C, 67.38; H, 3.77; N, 7.48.
(E)-1,3-bis[4–(3-Pyrid-3-ylcarbonyloxy)phenyl]-prop-2-en-1-one, 6
Yield = 88%, M. Pt. 166–167 °C. 1H NMR (500 MHz, CDCl3): δ 7.32 (d, J = 8.51 Hz, 2H), 7.36–7.43 (m, 2H), 7.50–7.52 (m, 2H), 7.53 (d, J = 15.0 Hz, 1H), 7.75 (m, J = 8.51 Hz, 2H) 7.85 (d, J = 15.5 Hz, 1H), 8.11–8.17 (m, 2H), 8.45–8.52 (m, 2H), 8.84–8.92 (m, 2H), 9.42 (dd, J = 4.41, 1.89 Hz, 2H). 13C NMR (125 MHz, CDCl3): δ 189.06, 163.57, 154.19, 163.42, 154.16, 151.34, 144.00, 138.04, 136.21, 133.09, 130.40, 129.91, 123.78, 123.37, 122.02. Found C, 71.78; H, 3.94; N, 6.23%. Anal. (C27H18N2O5) requires C, 71.99; H, 4.03; N, 6.22.
QSAR analysis
The σ, π and MR constants of the aryl substituents in series 2 and 4 were taken from the literatureCitation9. Linear and semilogarithmic plots between these constants and the IC50 values in the Molt4/C8, CEM and L1210 assays were made using a commercial software packageCitation11. The linear plots between the σ values of the aryl groups in 4a–e and the IC50 values in the Molt4/C8, CEM and L1210 bioassays correlated significantly (p < 0.05). The p values of the semilogarithmic plots were <0.1 (Molt4/C8 and CEM screens) and <0.05 (L1210 test).
Cytotoxic assays
The compounds in series 2, 4 and 6 were evaluated against Molt4/C8, CEM and L1210 cells using a literature procedureCitation12. In brief, different concentrations of the compounds were incubated with the cells for 72 h (Molt4/C8 and CEM cells) or 48 h (L1210 cells) at 37 °C. The biodata portrayed in were obtained from at least three separate determinations.
Table 1. The evaluation of the compounds in series 2, 4 and 6 against Molt 4/C8, CEM and L1210 cells.
The evaluation of 2c, d, 4c, d against 56 ± 2 human tumor cell lines was undertaken by a literature procedureCitation13. The cell lines whose growth was maximally inhibited and stimulated by the dienones are as follows namely 2c: RPMI-8826 (leukemia), SK-MEL-28 (melanoma); 2d: HOP-92 (nonsmall cell lung cancer), SK-MEL-2 (melanoma); 4c: RXF 393 (renal cancer), A498 (renal cancer) and 4d: RPMI-8226 (leukemia), TK-10 (renal cancer).
The evaluation against HL-60, HSC-2, HSC-3, HSC-4, hepatocyte growth factor (HGF), hematopoietic progenitor cell (HPC) and human periodontal ligament fibroblast (HPLF) cells was achieved following a published methodologyCitation14 except the time of incubation was extended to 48 h. The CC50 values were obtained from dose–response curves.
Evaluation of in vivo toxicity
All of the compounds in series 2, 4 and 6 were examined for lethal effects and neurotoxicity in mice using a literature methodCitation15. Doses of 30, 100 and 300 mg/kg of the enones were injected intraperitoneally into mice who were observed after 0.5 and 4 h. Neurotoxicity was noted for the following compounds (dose in mg/kg, number of animals displaying neurotoxicity/number of mice treated with the compound), namely 2c (100, 1/8), 4e (100, 1/8; 300, 1/4) and 6 (100, 2/8; 300, 1/4). These observations were made 0.5 h after administration of the compounds, and in each case, neurotoxicity was absent after 4 h. Both 2d (200 mg/kg) and 4d (50 mg/kg) were injected intraperitoneally into rats, while 4d (30 mg/kg) and 4e (30 mg/kg) were administered orally to rats. The animals were observed after 0.25, 0.5, 1, 2 and 4 h for overt toxicity, but none was observed. Neurotoxicity in mice was indicated by their failing to remain on a rotating rod, while neurotoxicity in rats was by observation of impaired mobility.
Results and discussion
The evaluation of 2a–e, 4a–e and 6 against Molt4/C8, CEM and L1210 cells was undertaken and the results are portrayed in . In the Molt4/C8 bioassay, 2a,e and 4a–c have IC50 values of less than 10 μM and both 4b and 4c are equipotent to melphalan. In general, the compounds are less potent toward CEM and L1210 cells. The very low potency of 4e is surprising as the strongly electron-attracting aryl nitro group will reduce the atomic charge on the olefinic carbon atom adjacent to ring B, thereby enhancing its capacity to react with cellular thiols. An attempt was made to determine whether one or more of the physicochemical properties of the aryl substituents in series 2 and 4 correlated with cytotoxic potencies. The Hammett σ, Hansch π and molar refractivity (MR) values reflect the electronic, hydrophobic and steric properties, respectively, of the aryl substituents. Linear and semilogarithmic plots were made between the IC50 values and the σ, π and MR constants of the aryl groups in series 2 and 4. Positive correlations (p < 0.05) were observed between the σ values of the aryl groups in 4a–e and the IC50 values in the Molt4/C8, CEM and L1210 screens. Thus, in developing these compounds, strongly electron-repelling groups should be placed in ring A of series 4 such as the 4-isopropyloxy and 4-dimethylamino groups which have σp values of −0.45 and −0.83, respectivelyCitation16.
In order to find which of the spacer groups between rings A and B in series 2 and 4 is associated with greater potencies, the IC50 values of the compounds with the same aryl substituent were compared in each of the three screens. Thus, the potencies of 2a and 4a in the Molt4/C8 assay were compared and so forth in which standard deviations were taken into account. In seven comparisons, the analogs in series 2 were more potent, while in four cases the analogs in series 4 have lower IC50 figures and equal potency was noted in four comparisons. Thus, the spacer group present in 2 is marginally preferable and should be considered in the development of these compounds. This general assessment was also noted by the average IC50 values of 2a, b, d, e being lower than the figures for 4a, b, d, e, respectively.
The substitution of a 3-pyridylcarboxy group at the para position of ring A of 4a led to 6. A comparison of the potencies of 4a and 6 revealed that while 4a has marginally lower IC50 values than 6 towards Molt 4/C8 cells, the diester is approximately twice as potent as 4a in the CEM and L1210 screens. In future, the placement of the 3-pyridylcarboxy group in different positions of ring A of 4a should be undertaken in order to determine whether the capacity to liberate two molecules of niacin is associated with increases in cytotoxic properties.
If the esters 2, 4 and 6 hydrolyze, the question arises as to whether the cytotoxicity is due to the liberated enone. The unsaturated ketones 1a–e and 3a have been evaluated previously against Molt4/C8, CEM and L1210 cellsCitation10. The average IC50 values in these three screens of 1a–e and 3a are as follows, namely 17.1, 20.5, 22.0, 6.48, 5.16 and 18.6 μM, respectively. Hence, hydrolysis should occur with 2a–e and 4a (and likely with 4b–e and 6), cytotoxic phenols will be released. Thus, the bioactivity of series 2, 4 and 6 may be due to the molecules per se or to the liberated phenol or to a combination of both molecular species.
The next phase of the investigation was to evaluate several compounds against a wide range of human tumor cell lines. The principal reason for this endeavor was to find whether the dienones demonstrate different toxicities to various neoplasms. Such a variation in cytotoxicity toward different neoplastic cell lines may reveal compounds with greater growth-inhibitory properties towards tumors than nonmalignant cells. Hence, an extensive study probing for tumor-selective toxicity would be justified. Four representative compounds 2c, d and 4c, d were evaluated against approximately 56 human tumor cell lines from nine different neoplastic conditions, viz leukemia, melanoma and nonsmall cell lung, colon, central nervous system (CNS), ovarian, renal, prostate and breast cancersCitation13. Using a concentration of 10 μM, the data in reveal that while the compounds have modest cytotoxicity, the differences in toxicity toward approximately 56 human tumor cell lines are noteworthy. The range indicated in may be illustrated as follows. In the case of 10 μM of 4d, the percentage inhibition of RPMI-8226 leukemic cells is 47.4%, while growth of TK-10 renal cancer cells is stimulated by 64.6%. Hence, the range of cytotoxicity demonstrated is 47.4 plus 64.6 or 112. The evidence produced is sufficient to justify a more extensive examination of all of the compounds in series 2, 4 and 6 against both neoplasms and nonmalignant cells.
Table 2. The effect of 2c, 2d, 4c, 4d on approximately 56 human tumor cell lines.
All of the compounds in series 2, 4 and 6 were evaluated against four additional neoplastic cells namely human HL-60, HSC-2, HSC-3 and HSC-4 cell lines. These data are presented in . In general, the HL-60 cell line is the most sensitive to these compounds and in this bioassay 2a–c and 4a–d have CC50 values of less than 10 μM. Several compounds are more potent than melphalan (bioassay in parentheses), namely 2a, b, e, 4a (HSC-4). Equipotency with melphalan was demonstrated by 4a (HL-60), 2a, b, e, 4a, 6 (HSC-2), 2a–e, 4a–d, 6 (HSC-3) and 2c, d, 4b–d, 6 (HSC-4). Thus, in comparison with melphalan, the compounds display equipotency in 50% of the bioassays while in a further 9% of the cases, greater potency than this established drug was observed.
Table 3. Evaluation of 2a–e, 4a–e and 6 against various human neoplastic and nonmalignant cell lines.
Scheme 1. The synthetic methodology used in preparing series 2, 4 and 6. The reagents used are as follows: i = NaOH; ii = nicotinoyl chloride; iii = hydrogen chloride. The aryl substituents in series 2 and 4 are a: R = H; b: R = CH3; c: R = OCH3; d: R = Cl; e: R = NO2.
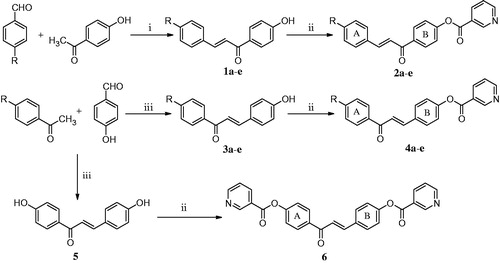
As noted previously in the discussion of the data presented in , 4e is substantially less toxic than all of the other compounds in series 2, 4 and 6. This enone could be utilized in developing the concept of activated soft compoundsCitation17. In this approach, a pharmacophoric group is attached to a nontoxic carrier moiety. The value of this concept is claimed to be that the gradual release of a chemically unstable compound is possible and also activated soft drugs can be developed with favorable physicochemical properties such as optimal hydrophobicity and hydrophilicity.
With a view to determining which of the linkers between rings A and B in series 2 and 4 is associated with greater cytotoxic potencies, the CC50 values of the compounds in series 2 were compared with those of series 4 which have the same aryl substituents. In 55% of the comparisons, the analogs in series 2 have higher potency while in 30% of the cases, the enones in series 4 have lower CC50 values. In three comparisons, equipotencies were observed. Furthermore, the average CC50 values in series 2 and 4 are 20.9 and >60.3 μM, respectively, and even when the outlier 4e is omitted from consideration, the average CC50 values of 2a–d and 4a–d are 19.0 and 25.4 μM, respectively. Hence, in developing these compounds, the antineoplastic data presented in both and reveal that the linker present in series 2 should be employed.
The unsaturated ketones 2, 4 and 6 were also evaluated against nonmalignant HGF, HPC and HPLF cells. The results are presented in . In general, these cell lines tolerate the compounds well. The lowest average CC50 figures are displayed by the unsubstituted compounds 2a and 4a, that is, substitution in the aryl ring leads to less toxic compounds toward these nonmalignant cells. This result may be due to ring A of 2a and 4a aligning easily at binding sites, whereas the bulky para substituents in 2b–e, 4b–e and 6 could prevent this toxic interaction from taking place. The CC50 values of the compounds in series 2, 4 and 6 were compared with that of melphalan. In 77% of the comparisons, melphalan was more toxic than the enones and only 4a had lower CC50 values toward HGF and HPLF cells than the reference drug. In 18% of the comparisons, equipotency was noted. Hence, in general, the compounds are substantially less toxic to various nonmalignant cells than a contemporary anticancer drug. However, an important reason for evaluating the compounds in series 2, 4 and 6 against three nonmalignant cell lines is to determine whether these enones display greater toxicity to neoplasms than normal cells. Under clinical conditions, a tumor will be surrounded by different types of nonmalignant cells. Hence, selectivity index (SI) figures were calculated which are the quotients of the average CC50 value of a compound towards HGF, HPC and HPLF cells and the CC50 figure against a specific neoplasm. The SI data are presented in which reveal that in general, the compounds display tumor-selective toxicity, especially toward HL-60 cells. In particular, 2b–d and 4a–c have average SI values in excess of 10 and are clearly lead molecules. The selective toxicity of melphalan to HL-60 cells is impressive, while on the other hand, 2a–e, 6 (HSC-2), 2a–e, 4a–d, 6 (HSC-3) and 2a–e, 4a–d, 6 (HSC-4) have higher SI values than melphalan, that is, in 79% of the comparisons made.
In order to identify the most promising compounds in terms of both cytotoxic potencies and selectivity, the potency-selectivity expression (PSE)Citation18 was applied. The PSE values are the product of the reciprocal of the average CC50 figure of the four neoplastic cell lines and the average SI value multiplied by 100; these data are presented in . The compounds with the highest PSE figures are 2b (>86.1) and 4a (114) which are greater than the PSE value of melphalan (80.2) and are lead molecules. In addition, 2a, c, d and 4b, c have PSE figures in excess of half of the value for melphalan. The enones 2b–e have higher PSE values than 4b–e, respectively, and hence, in terms of potency and tumor-selective toxicity, in general the spacer group in series 2 is favored.
Finally in view of the marked lethality of many anticancer drugsCitation19, a short-term evaluation of the toxicities of the compounds in series 2, 4 and 6 was performed. Doses of 30, 100 and 300 mg/kg were administered intraperitoneally to mice who were observed after 0.5 and 4 h. No mortalities were noted. After 0.5 h neurotoxicity was displayed by 2c (100 mg/kg), 4e (100 and 300 mg/kg) and 6 (100 and 300 mg/kg) in less than half of the animals. A further toxicological evaluation of representative compounds in rats was undertaken. Both 2d (200 mg/kg) and 4d (50 mg/kg) were injected intraperitoneally into rats while 4d (30 mg/kg) and 4e (30 mg/kg) were administered orally to these animals. The rats were observed at the end of 0.25, 0.5, 1, 2 and 4 h and no signs of overt toxicity were noted. These animal biodata are an additional encouragement reflecting the potential to develop these enones as candidate antineoplastics.
Conclusions
The evaluation of the compounds in series 2, 4 and 6 against seven neoplastic or transformed cells revealed that in general the enones have significant cytotoxic properties. In terms of potencies, the lead molecules identified are 2a, e, 4a–c in the Molt4/C8 bioassay and 2a–c, 4a–d in the HL-60 screen. Several of these compounds have similar potency as melphalan. The enones 2b–d, 4a–c have average SI values of greater than 10 and are clearly lead molecules with regard to tumor-selective toxicity. Furthermore, when SI values for individual cell lines are considered, most of the SI values generated are greater than the figures for melphalan. Both 2b and 4a have impressive PSE figures. The group between rings A and B in series 2 is marginally preferable to the spacer in 4a–e when potency and selective toxicity are concerned. A positive feature of these compounds is their being well tolerated in a short-term evaluation in mice.
In summary, this study provides ample evidence to justify expansion of these clusters of cytotoxins by analog development by the insertion of a variety of electron-repelling groups into ring A of series 4. Experiments should be undertaken to evaluate whether the esters undergo hydrolysis and if so, whether there is an optimal rate of hydrolysis in terms of potency and tumor-selective toxicity.
Acknowledgements
The authors thank the following agencies for financial support. The Canadian Institutes of Health Research provided a grant to J. R. Dimmock. The Belgian Concerted Research Actions (GOA 10/014) awarded funds to J. Balzarini which enabled L. van Berckelaer to generate the data presented in . The National Cancer Institute, USA kindly generated the results which are presented in . The Ministry of Education, Science, Sports and Culture gave a grant to H. Sakagami which covered the cost incurred in obtaining the data presented in . The in vivo evaluation for lethality and neurotoxicity was undertaken by the National Institute of Neurological Disorders and Stroke, NIH, USA.
Declaration of interest
The authors report no conflicts of interest. The authors alone are responsible for the content and writing of this article.
References
- Pati HN, Das U, Sharma RK, Dimmock JR. Cytotoxic thiol alkylators. Mini Rev Med Chem 2007;7:131–9
- Mutus B, Wagner JD, Talpas CJ, et al. 1-p-Chlorophenyl-4,4-dimethyl-5-diethylamino-1-penten-3-one hydrobromide, a sulfhydryl-specific compound which reacts irreversibly with protein thiols but reversibly with small molecular weight thiols. Anal Biochem 1989;177:237–43
- Page CP, Curtis M, Walker M, Hoffman B. Integrated pharmacology. 3rd ed. Philadelphia: Mosby, Elsevier; 2006. p. 643
- Dreizen S, McCredie KB, Keating MJ, Andersson BS. Nutritional deficiencies in patients receiving cancer chemotherapy. Postgrad Med 1990;87:163–7
- Ward WE. Principles of medical pharmacology. In: Kalant H, Grant DM, Mitchell J. eds. 7th ed. Toronto: Saunders Elsevier; 2007:856
- Raghavan S, Tria GS, Shen HC, et al. Tetrahydro anthranilic acid as a surrogate for anthranilic acid: application to the discovery of potent niacin receptor agonists. Bioorg Med Chem Lett 2008;18:3163–7
- Dimmock JR, Elias DW, Beazely MA, Kandepu NM. Bioactivities of chalcones. Curr Med Chem 1999;6:1125–49
- Orlikova B, Tasdemir D, Golais F, et al. Dietary chalcones with chemopreventive and chemotherapeutic potential. Genes Nutr 2011;6:125–47
- Hansch C. Leo AJ. Substituent constants for correlation analysis in chemistry and biology. New York: John Wiley and Sons; 1979:49
- Dimmock JR, Kandepu NM, Hetherington M, et al. Cytotoxic activities of Mannich bases of chalcones and related compounds. J Med Chem 1998;41:1014–26
- Statistical Package for Social Sciences, SPSS for Windows, Release 17.0, SPSS Inc., Chicago, 2008
- Baraldi PG, Nunez M. d C, Tabrizi MA, et al. Design, synthesis, and biological evaluation of hybrid molecules containing α-methylene-γ-butyrolactones and polypyrrole minor groove binders. J Med Chem 2004;47:2877–86
- Boyd MR, Paull KD. Some practical considerations and applications of the National Cancer Institute in vitro anticancer drug discovery screen. Drug Dev Res 1995;34:91–109
- Motohashi N, Wakabayashi W, Kurihara T, et al. Biological activity of Barbados cherry (Acerola fruits, fruit of Malphigia emarginata DC) extracts and fractions. Phytother Res 2004;18:221–3
- Stables JP, Kupferberg HJ. Molecular and cellular targets for antiepileptic drugs. In: Vanzini G, Tanganelli P, Avoli M, eds. London: John Libby and Company Ltd.; 1997:191–8
- Hansch C. Leo AJ. Substituent constants for correlation analysis in chemistry and biology. New York: John Wiley and Sons; 1979:50
- Bodor N. Novel approaches to the design of safer drugs: soft drugs and site-specific chemical delivery systems. Adv Drug Res 1984;13:255–331
- Das S, Das U, Sakagami H, et al. Dimeric 3,5-bis(benzylidene)-4-piperidones: a novel cluster of tumour-selective cytotoxins possessing multidrug-resistant properties. Eur J Med Chem 2012;51:193–9
- Quinn FR, Milne GWA. Toxicities derived from anti-tumor screening data. Fundam Appl Toxicol 1986;6:270–7