Abstract
A series of hybrids, which are composed of glycyrrhetic acid (GA) and slowly hydrogen sulfide-releasing donor ADT-OH, were designed and synthesized to develop anticancer and anti-inflammatory agents. Most of the compounds, whose inhibitory rates were comparable to or higher than those of GA and aspirin, respectively, significantly inhibited xylene-induced ear edema in mice. Especially, compound V4 exhibited the most potent inhibitory rate of 60.7%. Furthermore, preliminary structure–activity relationship studies demonstrated that 3-substituted GA derivatives had stronger anti-inflammatory activities than the corresponding 3-unsubstituted GA derivatives. In addition, anti-proliferative activities of compounds V1−9 were evaluated in three different human cancer cell lines. Compound V4 showed the most high potency against all three tumor cell lines with IC50 values ranging from 10.01 μM in Hep G2 cells to 17.8 μM in MDA-MB-231 cells, which were superior to positive GA.
Introduction
Hydrogen sulfide (H2S) is best known for pollution and its characteristic smell of rotten eggs. However, it is becoming increasingly clear that H2S takes part in a range of physiological and pathophysiological processes, regarded as the third gaseous signal molecule along with nitric oxide (NO) and carbon monoxide (CO)Citation1. H2S also plays an important role in the cardiovascular system, inflammation and central nervous system (CNS)Citation2. Inorganic NaHS, an hydrogen sulfide donor, has been extensively studied previously. However, its short half-time leads to an excessive amount of H2S released from NaHS rapidly in biological fluidCitation3, which may cause neurotoxicity and other side effects. So far, many slowly releasing H2S donor compounds have been developed. ADT-OH [5–(4-hydroxyphenyl)-3H-1,2-dithiole-3-thione] (1, ) is one of the most widely used slow-releasing H2S donorsCitation2. Our previous studies revealed that ADT-OH or its derivative (ADT-OCH3) was more potent than NaHS in protecting hippocampal neuronal HT22 cells against glutamate toxicity, and ADT-OH suppressed neuroinflammation by CaMKKβ-dependent activation of AMP-activated protein kinase in BV2 microglial cells. Moreover, ADT-OCH3 protected blood–brain barrier integrity following cerebral ischemia through NF-κB inhibition to suppress neuroinflammation-induced matrix metalloproteinase-9 and NADPH oxidase-4Citation4–6. In order to obtain tissue-specific H2S-related function, some new types of ADT-OH-compound hybrids have been developed. Recently, it has been reported that a new class of H2S-releasing ADT-OH moiety attached by an ester linkage to NSAIDs (a, b, c, ) exhibited more potent anti-inflammatory activity than NSAIDs alone. More importantly, they caused less gastric toxicity than NSAIDs themselvesCitation7–9. Compound d also inhibited the proliferation of cancer cellCitation10–12 (). An aspirin derivative (e, ) bearing both H2S and NO-releasing moieties inhibited 11 different human cancer cell lines with IC50 in the low nanomolar range, and showed anti-inflammatory activity comparable to aspirin in carrageenan-induced rat paw edema model. Significantly, this compound was devoid of any cellular toxicity by LDH release assayCitation13.
18-β-Glycyrrhetic acid (GA, 2, ), a pentacyclic triterpenoid compound, is the functional ingredient of many natural products. Many studies indicate that GA and its derivatives exhibit a variety of biological activities, for example, anti-inflammatory, antinociceptive, antiulcer, antioxidative, antiallergic, antiviral, antitumor, antibacterial, hepatic protective, antifilarial, anticoagulant and free-radical scavenging activitiesCitation14–25. GA has been used in therapy as a typical anti-inflammatory agent. In recent years, much interest has been attracted to the explanation of structure–activity relationships of GA derivatives due to its anticancer property without apparent toxicity. However, the antitumor activity of GA is weak, some novel GA derivatives have synthesized to enhance its potency. There are four positions, 2, 3, 11 and 30-C amenable to chemical modification in GA molecular structure. Pharmaceutical studies indicated that the removal of 11-carbonyl in ring C of GA not only eliminated pseudoaldosterone effect but also improved its anti-inflammatory, antiulcer and antiallergic activities, because the 11-oxo competed with the 3-oxo in ring A of cortical steroids at the active site of the reducing enzymeCitation14. GA ester obtained by linking glycosyl triazol to 30-COOH of GA (f, ), showed anticancer activityCitation26 and GA derivatives (g, ), with pyrazole at C-2 and C-3 positions, resulted in a lower inhibition of cell growth than the corresponding methyl or benzyl esters in position C-30Citation27. Compound (h, ), obtained by introducing 1-en-3-one and 9(11), 12-diene and 2, 20-dinitrile functionalities into the scaffold of GA, displayed 99% inhibition rate at a dose of 50 μM and exhibited stronger inhibitory activities against the LPS-induced NO production in RAW 264.7 macrophages relative to the parent compound GACitation28.
By far, most studies on GA and its derivatives were the antitumor and anti-inflammation, therefore, we focused on the synthesis and evaluation of anti-inflammatory and antiproliferative activities of several novel GA derivatives. In an attempt to probe further the roles of H2S in anti-inflammation and antitumor, we did some work on the chemical synthesis and pharmacological investigation of some novel H2S-releasing compounds beforeCitation29,Citation30. Therefore, we used GA as a lead compound and modified the structure on its C-3 or the removal of 11-carbonyl and then coupled the COOH of GA or its derivatives with H2S-releasing group ADT-OH through linker to enhance the anti-inflammatory and antitumor activities of these derivatives.
Results and discussion
Chemistry
We modified the structure of GA and expected to find novel GA derivatives applied to clinical drugs. In this present study, a series of GA derivatives coupling with H2S donor, including three GA esters (V1--V3), five 3-substituted GA esters (V4–V6, V8, V9) and one 11-deoxo GA esters (V7), described herein were synthesizedCitation31,Citation32 following the synthetic pathways depicted in Scheme 1, 2, 3, 4. The 3-acetyl GA (compound 4) has been synthesized from GA using acetyl chloride in pyridine at room temperatureCitation17. The synthesis of 11-deoxo GA (compound 5) was performed by the reduction of GA in the presence of Zn (containing 10% HgCl2) and concentrated. HCl in 1,4-dioxaneCitation33. Esterification of GA and 2-chloroacetyl chloride in the presence of pyridine in tetrahydrofuran afforded the desired compound 6 in high yieldCitation34. Subsequently, the corresponding 3-[2-(piperidin-1-yl)acetyl] GA (compound 7) was prepared by nucleophilic displacement reaction of compound 6 with piperidine in the presence of triethylamine in tetrahydrofuran at refluxing temperature. The target compounds V were prepared by the condensation of compound 2 (or 4, 5, 7) with corresponding compound 3, respectively, in the presence of anhydrous potassium carbonate and catalytic amount of potassium iodide in dry N,N-dimethylformamide at room temperature. The reactions were monitored by thin layer chromatography (TLC). The solvent was removed in evaporation, and the crude products were purified by column chromatography (silica gel, 200–300 mesh, petroleum ether (60–90 °C)/ethyl acetate). With increased reaction temperature, more GA derivatives were generated. All of the target compounds were novel and confirmed by 1H NMR, 13C NMR and HR-MS (See Supporting information).
Scheme 1. Reagents and conditions: (a) K2CO3, cat. KI, 60 °C, 12 h, chromatography, 86.2% for V1, 86.6% for V2, and 89.2% for V3.
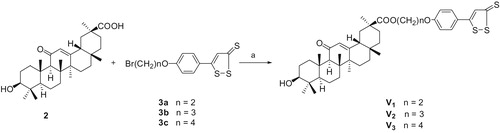
Scheme 2. Reagents and conditions: (a) Ac2O, Py, rt, 3 h, chromatography 92.7% for 4; (b) K2CO3, cat. KI, 3a, or 3b or 3c, 60 °C, 12 h, chromatography, 86.0% for V4, 89.5% for V5, and 89.6% for V6.
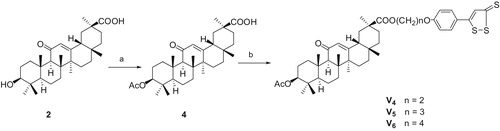
Biological activity
Anti-inflammatory activities of the GA derivatives V1–9 on the acute inflammation were evaluated on the xylene-induced ear edema in miceCitation35,Citation36. The data (shown in ) revealed that most of the GA derivatives exhibited moderate to good anti-inflammatory activities, compared with GA (at a dose of 18.8 mg/kg, inhibition 37.8%) and positive control drug aspirin (at a dose of 200 mg/kg, inhibition 41.0%) under identical conditions. Compound V4 showed the highest potency with an inhibitory rate of 60.7%, which was superior to the GA and aspirin. In addition, compound V3, V5, V6, V8, V9 possessed potent activities against xylene-induced mice ear edema, with inhibitory rates of 55.6%, 47.0%, 56.1%, 50.3% and 58.4%, respectively. However, V1 had slightly less anti-inflammatory activity, whose inhibitory rate was only 26.5%. Furthermore, preliminary structure–activity relationship studies (SARs) demonstrated that the potency of the GA derivative V1–3 increased with the extension of carbon chain of linker and the inhibitory activity order of compounds with the length of linker was n = 4 > n = 3 > n = 2. Compound V3 with a linker of n = 4 showed stronger anti-inflammatory activity with inhibitory rate of 55.6% against mouse ear edema than compound V1 with a linker of n = 2 (26.5%). Based on the data obtained, we obtained a variety of 3 substituents and found that various substituents, such as acetyl and 2-(piperidin-1-yl) acetyl leaded to different anti-inflammatory activities of these target compounds. The SARs of inflammatory activities demonstrated that introducing substituted groups on 3-position in GA increased the inhibitory rates compared with those of corresponding 3-unsubstituted GA derivatives. However, the most active compound V4 with the acetyl group at 3-position of GA had a linker of n = 2. Compound V7, removed 11-carbonyl in ring C of GA and coupled with ADT-OH, did not improved significantly its anti-inflammatory activity as design idea, with inhibitory rate of 39.4% compared with GA.
Table 1. Anti-inflammatory activities of GA derivatives against xylene-induced ear edema in mice*,†.
In this work, the cytotoxic activities of the GA derivatives V were evaluated through a MTT assayCitation37–39 in the following tumor cell lines: human breast cancer MDA-MB-231 cells, human prostate cancer DU-145 cells and human hepatocellular carcinoma Hep G2 cells. The data were presented in . The 3-acetyl GA derivatives (compound V4) with linker n = 2 not only showed the most highest potency of anti-inflammation, but also displayed the most potent antiproliferation activity against the tested cancer cell lines with IC50 values of 10.01, 11.96 and 17.80 μM, respectively, comparable to GA with corresponding IC50 values of 74.35, 69.40, 72.65 μM, respectively. Compound V1 with linker n = 2 showed more potent antitumor activity with IC50 values of 21.59, 24.66 μM, respectively, against DU-145 and MDA-MB-231 cell lines than GA. Compound V6 with linker n = 4 also possessed better potency with IC50 values of 36.37, 40.65 μM, respectively, against Hep G2 and MDA-MB-231 cell lines than GA. Other compounds had weak proliferative effect may be because of their poor solubility.
Table 2. Antiproliferation of GA derivatives vTable Footnote*,Table Footnote†.
Experimental
Synthesis
General chemistry
All chemicals (reagent grade) used were purchased from Sinopharm Chemical Reagent Co. Ltd (China). Citation1H NMR spectra was measured on Varian Unity Inova 400/600 MHz NMR Spectrometer and 13C NMR spectra was measured on Varian Unity Inova 300/400 MHz NMR Spectrometer at 25 °C and referenced to TMS. Chemical shifts are reported in ppm (d) using the residual solvent line as internal standard. Splitting patterns are designed as s, singlet; d, doublet; t, triplet; m, multiplet. High-resolution mass spectrometry (HRMS) acquired on Bruker Esquire liquid chromatography–ion trap mass spectrometer. Analytical thin-layer chromatography (TLC) was performed on the glass-backed silica gel sheets (silica gel 60 Å GF254). All compounds were detected using UV light (254 nm). Analytical HPLC was conducted on SHIMADZU LC-20AD. Hoechst 33 258 was bought from Beyotime (Haimen, China).
General procedure for the preparation of compounds
3-Acetyl-o-glycyrrhetinic acid (compound 4)
GA (1.88 g, 4.0 mmol) was dissolved in pyridine (10 mL), and then, acetic anhydride (3.78 mL, 40.0 mmol) was added dropwise to reaction solution, while the mixture was stirred at room temperature. After being stirred at room temperature for 12 h, the mixture was poured into ice water and filtered and the solid was dried. The crude product was purified by column chromatography (silica gel, 200 mesh) using petroleum ether/ethyl acetate (10:1, v/v) as the eluent to get compound 4 (1.90 g) as a white solid.
Yield 92%, m.p.: 312.0–313.0 °C. 1H NMR (400 MHz, CDCl3), δ(ppm): 5.72 (s, 1H, C12–H), 4.52 (dd, 1H, C3–H), 2.37 (s, 1H, C9–H), 2.06 (s, 3H, CH3), 1.37 (s, 3H, CH3), 1.23 (s, 3H, CH3), 1.17 (s, 3H, CH3), 1.13 (s, 3H, CH3), 0.88 (s, 6H, CH3), 0.84 (s, 3H, CH3).
11-Deoxy-glycyrrhetinic acid (compound 5)
GA (2.0 g, 4.26 mmol) and zinc powder (20 g, containing 1% HgCl2) were added to 1,4-dioxane (10 mL) and concentrated hydrochloric acid (1.20 mL) was added dropwise. After being stirred at the temperature of 15–20 °C for 2 h, the reaction mixture was filtrated and the solvent was evaporated. White solid (1.48 g, 74.0% yield) was got by column chromatography (silica gel, 200 mesh) using petroleum ether/ethyl acetate (7:1, v/v) as the eluent.
Yield 74%, m.p.: 323.1–324.0 °C. HR-MS: Calcd. For C30H47O3 [M − H]− 455.3531, Found: 455.3524.
3-o-(2-Chloro-acetyl) glycyrrhetinic acid (compound 6)
GA (1.41 g, 3.0 mmol) was dissolved in tetrahydrofuran (THF) (10 mL) and pyridine (0.36 mL, 4.5 mmol) was added. While the mixture was stirred at room temperature, chloroacetyl chloride (0.29 mL, 3.9 mmol) was added dropwise into the solution. After be stirring for 4 h, the reaction mixture was poured into water (40 mL), filtrated and the filter residue was dried to get 1.54 g white solid.
Yield 96.2%, m.p.: 260.8–261.8 °C. 1H NMR (400 MHz, CDCl3), δ(ppm): 5.72 (s, 1H, C12–H), 4.62 (m, 1H, C3–H), 4.07 (s, 2H, CH2), 2.37 (s, 1H, C9–H), 1.38 (s, 3H, CH3), 1.25 (s, 3H, CH3), 1.20 (s, 3H, CH3), 1.13 (s, 3H, CH3), 0.90 (s, 6H, CH3), 0.84 (s, 3H, CH3).
3-O-(2-Piperidyl-acetyl) glycyrrhetinic acid (compound 7)
Compound 6 (1.066 g, 1.95 mmol) was dissolved in THF (15 mL) and triethylamine (0.28 mL, 1.95 mmol) was added. After being stirring at room temperature for 10 min, piperidine (0.252 mL, 2.54 mmol) was dripped into the reaction mixture, which was stirred and refluxed for 10 h. After cooling to room temperature, the solvent was removed on a rotary evaporator. The crude product was recrystallized from acetone:water (10:1, v/v) to get 1.03 g white solid.
Yield 89%, m.p.: 305.6–307.2 °C. 1H NMR (400 MHz, CDCl3), δ(ppm): 5.71 (s, 1H, C12–H), 4.57 (m, 1H, C3–H), 3.19 (s, 2H, NCH2), 2.32 (s, 1H, C9–H), 1.32 (s, 3H, CH3), 1.01 (s, 3H, CH3), 0.86 (s, 6H, CH3), 0.72 (s, 3H, CH3), 1.10 (s, 3H, CH3), 1.07 (s, 3H, CH3).
General procedure for the preparation of compounds (V1–9)
Compound 3 (0.65 mmol), GA or its derivative compounds 4, 5, 7 (0.65 mmol), anhydrous K2CO3 (0.178 g, 1.30 mmol) and catalytic amount of KI were added to DMF (10 mL) and stirred at room temperature for 10 h. Then, ethyl acetate (10 mL) was added to the reaction mixture and extracted with water (3 × 10 mL). The organic layer was dried over anhydrous Na2SO4. After solvent was evaporated, the residue was purified by column chromatography (silica gel, 200 mesh) using petroleum ether/ethyl acetate as the eluent to obtain compound V1–9.
Glycyrrhetinic acid 2-[4–(3H-1,2-dithiole-3-thioxo-5-yl)-phenoxy] ethyl ester (V1)
Red powder, yield 86%, m.p.: 220.5–221.5 °C. 1H NMR (400 MHz, CDCl3), δ (ppm): 7.62 (d, 2H, J = 8.8 Hz, ArH), 7.39 (s, 1H, =CH), 7.01 (d, 2H, J = 8.8 Hz, ArH), 5.60 (s, 1H, C12–H), 4.50(m, 2H, OCH2), 4.27 (t, 2H, OCH2), 3.23 (m, 1H, C3–H), 2.78 (brs, 1H, OH), 2.32 (s, 1H, C9–H), 1.35 (s, 3H, CH3), 1.16 (s, 3H, CH3), 1.12 (s, 3H, CH3), 1.09 (s, 3H, CH3), 1.00 (m, 4H, CH3 & CH2), 0.81 (s, 3H, CH3), 0.74 (s, 3H, CH3); 13C NMR (400 MHz,CDCl3), δ(ppm): 215.53, 200.55, 176.75, 173.35, 169.55, 169.52, 162.09, 135.19, 129.11, 128.89, 125.00, 115.96, 79.14, 66.66, 62.73, 62.23, 55.31, 48.71, 45.79, 44.56, 43.55, 41.44, 39.54, 39.51, 38.08, 37.47, 33.10, 32.19, 31.55, 28.98, 28.69, 28.50, 27.71, 26.83, 26.79, 23.84, 19.03, 17.86, 16.80, 16.00; HR-MS: Calcd. For C41H55O5S3 [M + H]+: 723.3206, Found: 723.3191.
Glycyrrhetinic acid 3-[4–(3H-1,2-dithiole-3-thioxo-5-yl)-phenoxy] propylester (V2)
Red powder, yield 86%, m.p.: 79.1–80.7 °C; 1H NMR (400 MHz, CDCl3), δ (ppm): 7.54 (d, 2H, J = 8.8 Hz, ArH), 7.31 (s, 1H, =CH), 6.91 (d, 2H, J = 8.8 Hz, ArH), 5.54 (s, 1H, C12–H), 4.24 (t, 2H, OCH2), 4.06 (t, 2H, OCH2), 3.16 (dd, 1H, C3–H), 2.72 (brs, 1H, OH), 2.26 (s, 1H, C9–H), 2.10 (p, 2H, CH2), 1.29 (s, 3H, CH3), 1.08 (s, 3H, CH3), 1.07 (s, 3H, CH3), 1.03 (s, 3H, CH3), 0.93 (s, 3H, CH3), 0.74 (s, 3H, CH3), 0.69 (s, 3H, CH3); 13C NMR (400 MHz,CDCl3), δ(ppm): 215.21, 200.44, 176.58, 173.34, 169.52, 162.30, 134.80, 128.90, 128.66, 124.45, 115.69, 78.89, 72.01, 65.00, 62.04, 61.22, 55.10, 48.67, 45.62, 44.29, 43.42, 41.25, 39.34, 37.92, 37.29, 32.92, 32.04, 31.29, 28.76, 28.72, 28.64, 28.32, 27.92, 27.47, 26.63, 26.57, 23.64, 21.31, 19.40, 18.85, 16.62, 15.85; HR-MS: Calcd. For C42H57O5S3 [M + H]+: 737.3363, Found: 737.3387.
Glycyrrhetinic acid 4-[4–(3H-1,2-dithiole-3-thioxo-5-yl)-phenoxy] butyl ester (V3)
Red powder, yield 89%, m.p.: 138.2–139.2 °C; 1H NMR (400 MHz, CDCl3), δ (ppm): 7.60 (d, 2H, J = 8.8 Hz,), 7.39 (s, 1H, =CH), 6.98 (d, 2H, J = 8.8 Hz, ArH), 5.64 (s, 1H, C12–H), 4.20 (t, 2H, CH2), 4.08 (t, 2H, CH2), 3.23 (m, 1H, C3–H), 2.78 (brs, 1H, OH), 2.34 (s, 1H, C9–H), 1.37 (s, 3H, CH3), 1.16 (s, 3H, CH3), 1.13 (s, 3H, CH3), 1.12 (s, 3H, CH3), 1.01 (s, 3H, CH3), 0.81 (s, 6H, CH3); 13C NMR (400 MHz, CDCl3), δ (ppm): 215.23, 200.48, 176.67, 173.40, 169.58, 162.51, 134.74, 128.81, 128.690, 124.261, 115.706, 78.893, 67.907, 64.179, 62.053, 55.122, 48.687, 45.62, 44.25, 43.43, 41.27, 39.35, 37.95, 37.30, 32.95, 32.07, 31.31, 28.79, 28.67, 28.33, 27.49, 26.67, 26.60, 26.03, 25.74, 23.63, 18.88, 17.69, 16.60, 15.84; HR-MS: Calcd. For C43H59O5S3 [M + H]+ 751.3519, Found: 751.3477.
3-Acetyl-O-glycyrrhetinic acid 2-[4–(3H-1,2-dithiole-3-thioxo-5-yl)-phenoxy] ethyl ester (V4)
Red powder, yield 86%. m.p.: 96.0–97.0 °C; 1H NMR (600 MHz, CDCl3), δ (ppm): 7.64 (d, 2H, J = 8.8 Hz, ArH), 7.40 (s, 1H, =CH), 7.03 (d, 2H, J = 8.8 Hz, ArH), 5.58(s, 1H, C12-H), 4.57–4.43(m, 3H, OCH2 & C3–H), 4.28 (t, 2H, OCH2, J = 4.2 Hz), 2.34 (s, 1H, C9-H), 2.05(s, 3H, CH3), 1.35(s, 3H, CH3), 1.17(s, 3H, CH3), 1.15(s, 3H, CH3), 1.09(s, 3H, CH3), 0.88(s, 6H, CH3), 0.75(s, 3H, CH3); 13C NMR (300 MHz, CDCl3), δ (ppm): 214.99, 199.88, 176.24, 172.81, 170.95, 169.07, 161.57, 134.66, 128.61, 128.33, 124.50, 115.45, 80.52, 66.19, 62.19, 61.64, 54.90, 48.22, 45.30, 44.06, 43.06, 40.93, 38.68, 37.95, 37.60, 36.84, 32.56, 31.69, 31.07, 28.49, 28.17, 27.95, 26.32, 23.47, 23.28, 21.25, 18.55, 17.27, 16.61, 16.33; HR-MS: Calcd. For C43H57O6S3 [M + H]+: 765.3312, Found: 765.3229.
3-Acetyl-O-glycyrrhetinic acid 3-[4–(3H-1,2-dithiole-3-thioxo-5-yl)-phenoxy] propyl ester (V5)
Red powder, yield 89%, m.p.: 253.2–254.2 °C; 1H NMR (400 MHz, CDCl3), δ (ppm): 7.62 (d, 2H, J = 8.8 Hz, ArH), 7.40 (s, 1H, CH = CH), 6.98 (d, 2H, J = 8.8 Hz, ArH), 5.61 (s, 1H, C12–H), 4.52 (m, 1H, C3–H), 4.31 (t, 2H, OCH2), 4.12 (t, 2H, OCH2), 2.35 (s, 1H, C9–H), 2.18 (m, 2H, CH2), 2.06(s, 3H, CH3), 1.35 (s, 3H, CH3), 1.16(s, 3H, CH3), 1.15(s, 3H, CH3), 1.10 (s, 3H, CH3), 0.88(s, 6H, CH3), 0.75 (s, 3H, CH3); 13C NMR (400 MHz, CDCl3), δ(ppm): 215.07, 199.99, 176.31, 173.01, 171.01, 169.17, 162.03, 134.63, 128.64, 128.42, 124.26, 115.44, 80.54, 64.71, 61.71, 60.94, 54.96, 48.45, 45.37, 44.04, 43.17, 40.99, 38.77, 38.00, 37.67, 36.90, 32.63, 31.79, 31.06, 28.50, 28.39, 28.00, 26.36, 23.53, 23.32, 21.31, 18.61, 17.32, 16.65, 16.40; HR-MS: Calcd. For C44H59O6S3 [M + H]+ 779.3832, Found: 779.3849.
3-Acetyl-O-glycyrrhetinic acid 4-[4–(3H-1,2-dithiole-3-thioxo-5-yl)-phenoxy] butyl ester (V6)
Red powder, yield 89%, m.p.: 253.8–254.8 °C; 1H NMR (400 MHz, CDCl3), δ (ppm): 7.61 (d, 2H, J = 8.7 Hz, ArH), 7.40 (s, 1H, CH = CH), 6.98 (d, 2H, J = 8.7 Hz, ArH), 5.64 (s, 1H, C12–H), 4.23–4.16 (m, 2H, OCH2), 4.08 (t, 2H, OCH2), 2.36 (s, 1H, C9–H), 2.06 (s, 3H, CH3), 1.36 (s, 3H, CH3), 1.16 (s, 6H, CH3), 1.12 (s, 3H, CH3), 0.88 (s, 6H, CH3), 0.80 (s, 3H, CH3); 13C NMR (400 MHz, DMSO), δ (ppm): 214.76, 198.96, 177.63, 175.80, 173.82, 170.19, 169.89, 169.59, 162.04, 134.12, 129.00, 127.33, 127.24, 123.60, 115.52, 79.68, 67.59, 63.67, 60.85, 53.72, 48.07, 44.87, 43.55, 42.95, 37.91, 37.59, 36.50, 31.91, 31.54, 30.34, 28.39, 28.25, 27.76, 27.68, 26.08, 25.79, 25.29, 25.05, 23.23, 23.00, 21.00, 18.30, 16.94, 16.61, 16.17; HR-MS: Calcd. For C45H61O6S3 [M + H]+ 793.3625, Found: 793.3635.
11-Deoxyglycyrrhetinic acid 3-[4–(3H-1,2-dithiole-3-thioxo-5-yl)-phenoxy] propyl ester (V7)
Red powder, yield 82%, m.p.: 141.0–142.0 °C; 1H NMR (400 MHz, CDCl3), δ (ppm): 7.62 (d, 2H, J = 8.6 Hz, ArH), 7.40 (s, 1H, CH = CH), 6.98 (d, 2H, J = 8.6 Hz, ArH), 5.13 (m, 1H, C12–H), 4.30 (t, 2H, CH2), 4.14 (t, 2H, CH2), 3.22 (m, 1H, C3–H), 2.18 (p, 2H, CH2), 1.14 (s, 3H, CH3), 1.12 (s, 3H, CH3), 0.99 (s, 3H, CH3), 0.92 (s, 3H, CH3), 0.91 (s, 3H, CH3), 0.78 (s, 3H, CH3), 0.74 (s, 3H, CH3); 13C NMR (400 MHz, CDCl3), δ(ppm): 177.31, 173.16, 162.39, 144.71, 134.86, 131.17, 129.07, 128.82, 122.71, 115.69, 79.21, 65.82, 65.07, 60.77, 55.36, 48.61, 47.79, 44.54, 43.07, 41.73, 39.96, 38.98, 38.54, 37.12, 32.80, 32.18, 31.47, 28.86, 28.78, 28.44, 28.31, 27.43, 27.10, 26.33, 26.20, 23.69, 18.55, 16.96, 15.83, 15.72; HR-MS: Calcd. For C42H59O4S3 [M + H]+ 723.3570, Found: 723.3570.
3-[2-(Piperidyl-1-yl) acetyl-O]-glycyrrhetinic acid 3-[4–(3H-1,2-dithiole-3-thioxo-5-yl)- phenoxy] propyl ester (V8)
Red powder, yield 81%, m.p: 142.4–143.4 °C; 1H NMR (400 MHz, CDCl3), δ (ppm): 7.62 (d, 2H, J = 8.7 Hz, ArH), 7.39 (s, 1H, CH = CH), 6.99 (d, 2H, J = 8.7 Hz, ArH), 5.61 (s, 1H, C12–H), 4.61 (m, 1H, C3–H), 4.31 (t, 2H, OCH2), 4.13 (t, 2H, OCH2), 3.50 (s, 2H, NCH2), 2.35 (s, 1H, C9–H), 2.18 (m, 2H, CH2), 1.36 (s, 3H, CH3), 1.15 (s, 6H, CH3), 1.10 (s, 3H, CH3), 0.87 (s, 6H, CH3), 0.75 (s, 3H, CH3); 13C NMR (300 MHz, CDCl3), δ (ppm): 214.92, 199.90, 176.23, 172.97, 170.08, 169.19, 161.95, 134.50, 128.57, 128.30, 124.15, 115.35, 80.73, 64.64, 61.60, 60.87, 59.97, 54.82, 53.99, 48.37, 45.29, 43.97, 43.10, 40.91, 38.64, 37.95, 37.60, 36.80, 32.53, 31.72, 30.98, 29.61, 28.44, 28.33, 28.01, 26.29, 25.57, 23.68, 23.59, 23.28, 18.53, 17.26, 16.69, 16.33.; HR-MS: Calcd. For C49H68NO6S3 [M + H] + 862.4203, Found: 862.4203.
3-[2-(Piperidyl-1-yl) acetyl-O]-glycyrrhetinic acid 4-[4–(3H-1,2-dithiole-3-thioxo 5-yl)- phenoxy] butyl ester (V9)
Red powder, yield 86%, m.p.: 107.3–108.3 °C; 1H NMR (400 MHz, CDCl3), δ (ppm): 7.60 (d, 2H, J = 8.8 Hz, ArH), 7.39 (s, 1H, CH = CH), 6.98 (d, 2H, J = 8.7 Hz, ArH), 5.64 (s, 1H, C12–H), 4.59 (m, 1H, C3–H), 4.19 (m, 2H, OCH2), 4.08 (m, 2H, OCH2), 3.58 (s, 2H, NCH2), 2.35 (s, 1H, C9–H), 1.37 (s, 3H, CH3), 1.16 (s, 6H, CH3), 1.12 (s, 3H, CH3), 0.87 (s, 6H, CH3), 0.80 (s, 3H, CH3); 13C NMR (400 MHz,CDCl3), δ(ppm):13C NMR (300 MHz, CDCl3), δ (ppm): 214.98, 199.92, 176.34, 169.38, 162.20, 134.51, 128.53, 128.37, 127.98, 124.02, 115.41, 115.16, 81.86, 67.61, 63.87, 61.61, 54.91, 53.28, 48.41, 45.32, 43.97, 43.16, 40.97, 38.65, 37.98, 37.64, 36.84, 32.55, 31.79, 29.15, 28.50, 28.47, 28.38, 28.06, 26.37, 26.29, 26.25, 26.18, 25.73, 25.47, 23.55, 23.31, 18.59, 17.27, 16.66, 16.34; HR-MS: Calcd. For C50H70NO6S3 [M + H]+ 876.4360, Found: 876.4360.
Biological assay
Xylene-induced ear edema testCitation31
GA derivatives V1–9 was administered orally, as finely homogenized suspension in 0.5% carboxymethylcellulose (2 mL per 100 g body weight), at doses of 40 μmol/kg except aspirin group at the dose of 200 mg/kg 1 h before application of xylene. A total of 20 μL of xylene was applied to the inner surface of the right ear of each mouse. The left ear remained untreated. Control animals received the vehicle (0.5% carboxymethylcellulose). GA was used as reference drug. The animals were sacrificed by cervical dislocation 1 h later, and two ear plugs (7 mm in diameter) were removed from both the treated ear and the untreated ear. Weights of treated and untreated ear plugs were measured with an electronic balance. The difference in weight of the two ear plugs was taken as a measure of edematous response.
MTT assay
Cell lines were seeded at a density of 7 × 103 cells/well in 96-well microtiter plates (Costar). After 24 h, exponentially growing cells were exposed to the indicated compounds at final concentrations ranging from 0.1–40 mg/mL. After 48 h, cell survival was determined by the addition of an MTT solution (20 lL of 5 mg/mL MTT in PBS). After 6 h, 100 mL of 10% SDS in 0.01 N HCl was added, and the plates were incubated at 37 °C for a further 4 h; optical absorbance was measured at 570 nm on an LX300 Epson Diagnostic microplate reader. Survival ratios are expressed in percentages with respect to untreated cells. IC50 values were determined from replicates of six wells from at least two independent experiments.
Conclusion
In this study, a series of glycyrrhetic acid derivatives were synthesized and evaluated for anti-inflammatory activity as well as antiproliferative activity of three cancer cell lines. As expected, these compounds exhibited remarkable effects on mice against xylene-induced ear edema. Especially, compound V4 showed the most potent inhibitory activity (inhibitory rate = 60.7%), much better than that of GA (inhibitory rate 37.8%). V4 also exhibited the most potent antiproliferative activity with IC 50 10.01 μM for Hep G2, 11.96 μM for DU-145 and 17.80 μM for MDA-MB-231), much better than that of the positive control GA with IC 50 69.40 μM for Hep G2, 69.40 μM for DU-145 and 72.65 μM for MDA-MB-231). Thus, compound V4 is a potential anti-inflammatory agent
Supplementary material available online
IENZ_1144596_Supplementary_Information.pdf
Download PDF (394.1 KB)Declaration of interest
This research was funded by Science and Technology Program from Suzhou City (Grant No: SYS20 1317) and PAPD (the Priority Academic Program Development of Jiangsu Higher Education Institutions)
References
- Wang R. Two's company, three's a crowd: can H2S be the third endogenous gaseous transmitter?. FASEB J 2002;16:1792–8
- Caliendo G, Cirino G, Santagada V, Wallace JL. Synthesis and biological effects of hydrogen sulfide (H2S): development of H2S-releasing drugs as pharmaceuticals. J Med Chem 2010;53:6275–86
- Distrutti E, Sediari L, Mencarelli A, et al. Evidence that hydrogen sulfide exerts antinociceptive effects in the gastrointestinal tract by activating KATP channels. J Pharmacol Exp Ther 2006;316:325–35
- Jia J, Xiao Y, Wang W, et al. Differential mechanisms underlying neuroprotection of hydrogen sulfide donors against oxidative stress. Neurochem Int 2013;62:1072–8
- Wang Y, Jia J, Ao GZ, et al. Hydrogen sulfide protects blood-brain barrier integrity following cerebral ischemia. J Neurochem 2014;129:827–38
- Zhou X, Cao Y, Ao GZ, et al. CaMKKβ-dependent activation of AMP-activated protein kinase is critical to suppressive effects of hydrogen sulfide on neuroinflammation. Antioxid Redox Signal 2014;21:1741–58
- Fiorucci S, Orlandi S, Mencarelli A, et al. Enhanced activity of a hydrogen sulphide-releasing derivative of mesalamine (ATB-429) in a mouse model of colitis. Br J Pharmacol 2007;150:996–1002
- Wallace JL. Hydrogen sulfide-releasing anti-inflammatory drugs. Trends Pharmacol Sci 2007;28:501–6
- Wallace JL, Caliendo G, Santagada V, Cirino G. Markedly reduced toxicity of a hydrogen sulphide-releasing derivative of naproxen (ATB-346). Brit J Pharmacol 2010;159:1236–46
- Chattopadhyay M, Kodela R, Nath N, et al. Hydrogen sulfide-releasing aspirin suppresses NF-κB signaling in estrogen receptor negative breast cancer cells in vitro and in vivo. Biochem Pharmacol 2012;83:723–32
- Chattopadhyay M, Kodela R, Nath N, et al. Hydrogen sulfide-releasing NSAIDs inhibit the growth of human cancer cells: a general property and evidence of a tissue type-independent effect. Biochem Pharmacol 2012;83:715–22
- Chattopadhyay M, Kodela R, Nath N, et al. Hydrogen sulfide-releasing aspirin modulates xenobiotic metabolizing enzymes in vitro and in vivo. Biochem Pharmacol 2012;83:733–40
- Kodela R, Chattopadhyay M, Kashfi K. NOSH-aspirin: a novel nitric oxide-hydrogen sulfide-releasing hybrid: a new class of anti-inflammatory pharmaceuticals. ACS Med Chem Lett 2012;3:257–62
- Wang CY, Kao TC, Lo WH, Yen GC. Glycyrrhizic acid and 18β-glycyrrhetinic acid modulate lipopolysaccharide-induced inflammatory response by suppression of NF-κB through PI3K p110δ and p110γ inhibitions. J Agric Food Chem 2011;59:7726–33
- Kao TC, Shyu MH, Yen GC. Glycyrrhizic acid and 18beta-glycyrrhetinic acid inhibit inflammation via PI3K/Akt/GSK3beta signaling and glucocorticoid receptor activation. J Agric Food Chem Food 2010;58:8623–9
- Takahashi K, Shibata S, Yano S, et al. Chemical modification of glycyrrhetinic acid in relation to the biological activities. Chem Pharm Bull 1980;28:3449–52
- Beseda I, Czollner L, Shah PS, et al. Synthesis of glycyrrhetinic acid derivatives for the treatment of metabolic diseases. Bioorg Med Chem 2010;18:433–54
- Kalani K, Kushwaha V, Verma R, et al. Glycyrrhetinic acid and its analogs: a new class of antifilarial agents. Bioorg Med Chem Lett 2013;23:2566–70
- Song J, Ko Hs, Sohn EJ, et al. Inhibition of protein kinase C α/βII and activation of c-Jun NH2-terminal kinase mediate glycyrrhetinic acid induced apoptosis in non-small cell lung cancer NCI-H460 cells. Bioorg Med Chem Lett 2014;24:1188–91
- Carraher CE Jr., Ngoc Trang Caroline T, Roner MR, et al. Synthesis of organotin poly(ether esters) from reaction with glycyrrhetinic acid and their preliminary activity against various cancer cell lines. Inorg Chim Acta 2014;423:83–92
- Jiang L, Wang Q, Shen S, et al. Discovery of glycyrrhetinic acid as an orally active, direct inhibitor of blood coagulation factor xa. Thromb Res 2014;133:501–6
- Jayasooriya RGPT, Dilshara MG, Park SR, et al. 18β-Glycyrrhetinic acid suppresses TNF-α induced matrix metalloproteinase-9 and vascular endothelial growth factor by suppressing the Akt-dependent NF-κB pathway. Toxicol In Vitro 2014;28:751–8
- Schwarz S, Siewert B, Xavier NM, et al. A “natural” approach: synthesis and cytoxicity of monodesmosidic glycyrrhetinic acid glycosides. Eur J Med Chem 2014;72:78–83
- Fu XX, Du LL, Zhao N, et al. 18β-Glycyrrhetinic acid potently inhibits Kv1.3 potassium channels and T cell activation in human Jurkat T cells. J Ethnopharmacol 2013;148:647–54
- Salomatina OV, Markov AV, Logashenko EB, et al. Synthesis of novel 2-cyano substituted glycyrrhetinic acid derivatives as inhibitors of cancer cells growth and NO production in LPS-activated J-774 cells. Bioorg Med Chem 2014;22:585–93
- Parida PK, Sau A, Ghosh T, et al. Synthesis and evaluation of triazole linked glycosylated 18β-glycyrrhetinic acid derivatives as anticancer agents. Bioorg Med Chem Lett 2014;24:3865–8
- Gao C, Dai FJ, Cui HW, et al. Synthesis of novel heterocyclic ring-fused 18β-glycyrrhetinic acid derivatives with antitumor and antimetastatic activity. Chem Biol Drug Des 2014;84:223–33
- You R, Long W, Lai Z, et al. Discovery of a potential anti-inflammatory agent: 3-oxo-29-noroleana-1,9(11),12-trien-2,20-dicarbonitrile. J Med Chem 2013;56:1984–95
- Ao GZ, Qiao CH, Hou BB, et al. Glycyrrhetinic acid derivatives and their application as antitumor agents. Soochow University. CN102241726; 2013
- Ao GZ, Xu GL, Yang SW, et al. Application of glycyrrhetinic acid derivatives in the preparation of medicine for anti-inflammation. Soochow University. CN102579462; 2013
- Bennett F, Girijavallabhan VM, Patel NM, et al. Tetrahydrofuran phosphate- and hydroxy esters, as prodrugs for the corresponding antifungal agent. Assignee. Schering Corporation, WO. 9915522; 1999
- Gok Y, Alici B, Cetinkaya E, et al. Ionic liquids as solvent for efficient esterification of carboxylic acids with alkyl halides. Turk J Chem 2010;34:187–91
- Csuk R, Schwarz S, Kluge R, Stroehl D. Synthesis and biological activity of some antitumor active derivatives from glycyrrhetinic acid. Eur J Med Chem J Med. 2010;45:5718–23
- Yong JP, Wang JW, Aisa HA, Liu L. Synthesis of glycyrrhetic acid derivatives. J Chem Nat Compd 2008;44:194–6
- Xu GL, Du YF, Cheng J, et al. Inhibition of inflammatory mediators contributes to the anti-inflammatory activity of KYKZL-1 via MAPK and NF-κB pathway. Toxicol Appl Pharmacol 2013;272:221–9
- Xu GL, Liu F, Ao GZ, et al. Anti-inflammatory effects and gastrointestinal safety of NNU-hdpa, a novel dual COX/5-LOX inhibitor. Eur J Pharmacol 2009;611:100–6
- Huang B, Du D, Zhang R, et al. Synthesis, characterization and biological studies of diosgenyl analogues. Bioorg Med Chem Lett 2012;22:7330–4
- Atherden LM. Studies with glycyrrhetic acid; inhibition of metabolism of steroids in vitro. Biochem J 1958;69:75–8
- Xu YY, Cao Y, Ma H, et al. Design, synthesis and molecular docking of α,β-unsaturated cyclohexanone analogous of curcumin as potent EGFR inhibitors with antiproliferative activity. Bioorg Med Chem 2013;21:388–94