Abstract
Acetylcholinesterase inhibitors and compounds that trigger Aβ amyloid oligomerization and fibrillization represent an opportunity to discover new drug candidates to treat Alzheimer’s disease. In this work, we synthesized nine new acylhydrazones and a known one, both employing 3-carboethoxy-4-quinolone derivatives as starting materials with chemical yields ranging from 63% to 90%. We evaluated the effect of these compounds on the acetylcholinesterase (AChE) activity and the fibrillization of Aβ42 peptide. Except for one acylhydrazone, the compounds exhibited good inhibitory effect on AChE (1.2 μM < IC50 values < 17 μM). They also showed a significant decrease in the thioflavin-T fluorescence emission, suggesting an inhibitory effect on the Aβ42 fibril formation.
Introduction
Alzheimer’s disease (AD) is an age-related neurodegenerative process with a limited therapeutic arsenal. The physiopathology of AD is very complex, but a cognitive decline due to decreased cholinergic activity is quite acceptedCitation1,Citation2. Therefore, acetylcholinesterase inhibitors, such as donepezil, galantamine and rivastigmine, are currently indicated for the treatment of AD symptoms. In the context of the development of AD drug candidates, we are involved in the synthesis and biological evaluation of some 3-carboethoxy-4-quinolone derivativesCitation3,Citation4. We noted that 3-carboethoxy-4-quinolone inhibited acetylcholinesterase (AChE), an important target for drugs action. However, the effect was moderated (IC50 = 70 μM). More recently, Pudlo et al.Citation5 have found that quinolone–benzylpiperidine hybrids inhibited AChE, with IC50 values ranging from 0.37 to 2.46 μM. Thus, we were dedicated to find out more effective quinolone derivatives. Hydrazides, hydrazones and acylhydrazones are a class of nitrogen compounds, which are extensively studied and most of them are bioactive compounds, including some naturally occurring onesCitation6. These organic functions have been pointed out as the structural moiety related to the biological activityCitation7. For example, anticancerCitation8, antimycobacterialCitation9, analgesic and anti-inflammatoryCitation10 activities are distinctive in this class of compounds. In view of this, we studied some molecular hybrids based on the structures of the 3-carboxy-4-quinolone and hydrazones derived from aromatic aldehydes ().
Figure 1. Structure of acylhydrazones derived from 3-carboethoxy-4-quinolone and aromatic aldehydes.
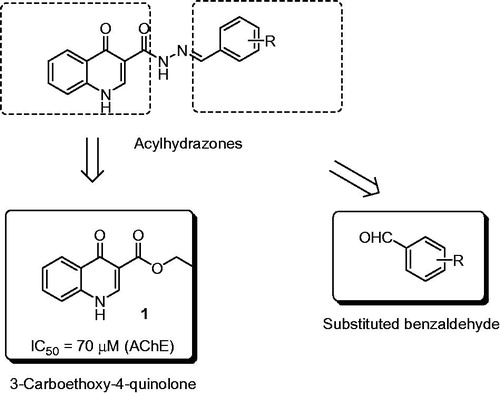
In this work, we report the synthesis and effect of 10 acylhydrazone-4-quinolone derivatives on the acetylcholinesterase activity. Since β-amyloid (Aβ) peptide is also involved in the pathogenesis of AD, we studied the effect of some acylhydrazones on the Aβ fibril formation.
Experimental
Chemistry
Solvents and reagents were purchased from Sigma Aldrich (São Paulo, Brazil) and used without purification. The reaction progress was monitored by thin-layer chromatography (TLC) and revealed by UV-Vis. Compounds were purified by column chromatography on silica gel (230–4000 mesh) and a mixture of ethyl acetate and hexane as eluent. Uncorrected melting points were determined in a Fisatom Mod.431 (115 V) device. IR spectra were recorded on a BOMEN (MBSERIES model) equipment. 1H and 13C NMR spectra (1D and 2D) were acquired in a Bruker spectrometer operating at 400 or 500 MHz for 1H and 100 MHz or 125 MHz for 13C, respectively. 1H and 13C spectra at 600 MHz were obtained in Avance 600 as specified for each compound. Tetramethylsilane was used as internal standard. High-resolution mass spectrometry spectra were obtained in a LTQ-Orbitrap Discovery Thermo Scientific.
Synthesis and characterization
The synthesis of the desired acylhydrazones started with the preparation of 3-carboethoxy-4-quinolone 1 (), which was converted into the hydrazide 2. The next step consisted in the coupling of hydrazide 2 with substituted aromatic aldehydes. The synthesized acylhydrazones melt above 250 °C, with decomposition.
Preparation of ethyl-4-oxo-1,4-dihydroquinoline-3-carboxylate (1)
Beige solid, yield: 88%. mp 284–286 °C. IR (KBr, cm −1): ν 3101–3169 (–NH), 1701 (C=O, hydrazide), 1622 (C=O, 4-carboxy); 1H NMR (CF3CO2D, 600 MHz), δ (ppm): 10.09 (s, 1H, H-2), 9.38 (dd, J = 8.7 Hz and 0.6 Hz, 1H, H-5), 9.10 (t, J = 8.7 Hz, 1H, H-6), 8.97 (td, J = 7.8 and 0.6 Hz, 1H, H-7), 8.71 (d, J = 7.8 Hz, 1H, H-8), 5.34 (q, J = 7.2 Hz, 2H), 2.20 (t, J = 7.2 Hz, 3H); Citation11 C NMR (CF3CO2D, 150 MHz), δ (ppm): 173.8 (C=O), 167.7 (C=O), 139.7 (CH-2), 139.5 (C0–9), 138.5 (CH-7), 130.6 (CH-5), 130.3 (C0–10), 125.1 (CH-6), 124.9 (CH-8), 119.9 (C0–3), 65.3 (CH2), 12.7 (CH3).
These data are in accordance with the literatureCitation3,Citation12.
The numbering of H and C of was done as follows (Scheme 1):
Preparation of 4-oxo-1,4- dihydroquinolone-3-carbohydrazide (2)
A stirred equimolar mixture of 3-carboethoxy-4-quinolone 1 (0.55 mmol) and 50% aqueous hydrazine monohydrate (10.28 mmol) in ethanol (5 mL) was heated for 20 h. After this period, the solvent was removed under reduced pressure. The crude product was purified by recrystallization with ethanol to afford hydrazide 2.
82% yield, yellow solid. Melting point 280–282 °C (decomposition). IR (KBr, cm− 1): ν 3448–3265 (HN–C=O and H2N–N), 1676 (C=O, hydrazide), 1631 (C=O, 4-carboxy); 1H NMR (d6-DMSO, 600 MHz) δ (ppm): 10.72 (s, 1H, –CONH), 8.74 (s, 1H, H-2), 8.27 (dd, J = 8.4 and 1.2 Hz, 1H, H-5), 7.78 (td, J = 7.5 and 1.2 Hz, 1H, H-6), 7.68 (dd, J = 7.5 Hz, 1H, H-7), 7.47 (td, J = 7.5 and 1.2 Hz, 1H, H-8). 13C NMR (d6-DMSO, 125 MHz) δ (ppm): 176.2 (C=O), 164.6 (C=ONHNH2), 143.6 (CH-2), 139.5 (C0–9), 133.2 (CH-7), 126.4 (C0–10), 125.9 (CH-5), 125.4 (CH-6), 119.4 (CH-8), 110.8 (C0–3). These data are in accordance with the literatureCitation13.
General procedures for the preparation of acylhydrazones derivatives
An equimolar mixture of the substituted benzaldehyde (4.3 mmol) and hydrazide 2 (4.3 mmol) was stirred under reflux in ethanol (3 mL) in the presence of 20 drops of piperidine for 12–18 h. The solvent excess was removed under reduced pressure and the collected solid washed with the minimum amount of ethanol possible and then dried at room temperature.
4-oxo-N′-[(1E)-phenylmethylidene]-1,4-dihydroquinoline-3-carbohydrazide (3)
Beige solid; yield: 68%. IR (KBr, cm− 1): ν 3267–3057 (HN–C=O and H2N–N), 1680 (C=O hydrazide), 1617 (C=O, quinolone); 1H NMR (d6-DMSO, 500 MHz) δ (ppm): 13.58 (s, 1H, –CONH), 8.85 (s, 1H, H-2), 8.43 (s, 1H, -N=CH-14), 8.31 (dd, J = 8.1 and 1.0 Hz, 1H, H-5), 7.81 (td, J = 8.1 and 1.0 Hz, 1H, H-6), 7.77 (dd, J = 7.4 and 1.4 Hz, 2H, H-16 and H-20), 7.75 (d, J = 7.8 Hz, 1H, H-7), 7.53 (td, J = 7.8 Hz and 1.0 Hz, 1H, H-8), 7.45 (m, 3H, H-17, H-18 and H-19), 13C NMR (d6-DMSO, 150 MHz) δ (ppm): 176.1 (C=O), 161.5 (C=ONH), 147.8 (CH-14), 144.4 (CH-2) 139.2 (C0–9), 134.5 (C0–15), 133.2 (CH-7), 130.1 (CH-18), 128.9 (CH-16 and CH-20), 127.3 (CH-17 and CH-19); 125.9 (CH-5), 125.6 (C0–10), 125.5 (CH-6), 119.3 (CH-8), 110.1 (C0–3); Calculated for C17H14N3O2 (M + H+): 292.1086, Found 292.1086.
N′-[(1E)-(4-chlorophenyl)methylidene]-4-oxo-1,4-dihydroquinoline-3-carbohydrazide (4)
Beige solid, yield: 72%. IR (KBr, cm −1): ν 3273–3026 (HN–C=O and H2N–N), 1645 (C=O hydrazide), 1618 (C=O, quinolone); 1H NMR (d6-DMSO, 500 MHz) δ (ppm): 13.40 (s, 1H, –CONH), 8.89 (s, 1H, H-2), 8.47 (s, 1H, –N=CH-14), 8.34 (dd, J = 8.0 and 1.0 Hz, 1H, H-5), 7.83 (d, J = 8.6 Hz, 2H, H-16 and H-20), 7.81 (td, J = 8.2 and 1.0 Hz, 1H, H-7), 7.78 (d, J = 8.2 Hz, 1H, H-8), 7.57 (td, J = 8.0 and 1.0 Hz, 1H, H-6), 7.57 (d, J = 8.6 Hz, 2H, H-17 and H-19); 13C NMR (d6-DMSO, 125 MHz) δ (ppm): 176.5 (C=O), 162. 1 (C=ONH), 146.9 (CH-14), 144.9 (CH-2) 139.7 (C0–9), 134.9 (C0–18), 133.8 (C0–15), 133.6 (CH-7), 129.4 (CH-17 and CH-19), 129.3 (CH-16 and CH-20), 126.3 (C0–10), 126.3 (CH-6), 125.9 (CH-5), 119.8 (CH-8), 110.3 (C0–3); TOF MS (ESI+) Calculated for C17H13ClN3O2 (M + H+): 326.0696, Found 326.0716.
N′-[(1E)-(3-chlorophenyl)methylidene]-4-oxo-1,4-dihydroquinoline-3-carbohydrazide (5)
Yellow solid, yield: 63%.
IR (KBr, cm−1): ν 3265–3062 (HN–C=O and H2N–N), 1646 (C=O hydrazide), 1620 (C=O, quinolone); 1H NMR (d6-DMSO, 400 MHz) δ (ppm): 13.38 (s, 1H, –CONH), 8.86 (s, 1H, H-2), 8.43 (s, 1H, –N=CH-14), 8.30 (dd, J = 8.1 and 1.2 Hz, 1H, H-5), 7.82 (td, J = 7.0 and 1.2 Hz, 1H, H-7), 7.81 (s, 1H, H-16), 7.74 (d, J = 7.0 Hz, 1H, H-8), 7.72 (dd, J = 5.5 and 3.3 Hz, 1H, H-20), 7.54 (td, J = 8.1 and 1.2 Hz, 1H, H-6), 7.50 (dd, J = 5.5 and 3.3 Hz, 2H, H-18 and H-19); 13C NMR (d6-DMSO, 100 MHz) δ (ppm): 176.5 (C=O), 162.1 (C=ONH), 146.6 (CH-14), 144.9 (CH-2), 139.5 (C0–9), 137.1 (C0–15), 134.1 (C0–17), 133.6 (CH-7); 131.2 (CH-18), 130.1 (CH-6), 126.9 (CH-16), 126.3 (C0–10), 126.3 (CH-19), 125.9 (CH-5), 125.9 (CH-20), 119.7 (CH-8), 110.4 (C0–3). Calculated for C17H13ClN3O2 (M + H+): 326.0696. Found, 326.0716.
N′-[(1E)-(2,3-dichlorophenyl)methylidene]-4-oxo-1,4-dihydroquinoline-3-carbohydrazide (6)
Yellow solid, yield: 65%.
IR (KBr, cm −1): ν 3265–3067 (HN–C=O and H2N–N), 1662 (C=O hydrazide), 1621 (C=O, quinolone); 1H NMR (d6-DMSO, 500 MHz) δ (ppm): 13.59 (s, 1H, –CONH), 8.89 (s, 1H, H-2), 8.76 (s, 1H, –N=CH-14), 8.32 (d, J = 7.8 Hz, 1H, H-5), 8.00 (d, J = 7.0 Hz, 1H, H-8), 7.83 (s, 1H, H-18), 7.76 (m, 2H, H-19 and H-20), 7.55 (t, J = 7.0 Hz, 1H, H-7), 7.49 (t, J = 7.8 Hz, 1H, H-6); 13C NMR (d6-DMSO, 125 MHz) δ (ppm): 173.5 (C=O), 161.9 (C=ONH), 148.2 (C0–19), 145.5 (CH-14), 142.8 (CH-2), 142.6 (C0–16), 141.4 (C0–17), 139.9 (C0–9), 133.4 (CH-18), 131.9 (CH-7), 128.9 (CH-6), 126.9 (C0–10), 125.8 (CH-20), 126.3 (CH-19), 124.5 (CH-5), 116.8 (CH-8), 107.9 (C0–3); TOF MS (ESI+) Calculated for C17H12Cl2N3O2 (M + H+): 360.0307; Found: 361.1557.
N′-[(1E)-(4-nitrophenyl)methylidene]-4-oxo-1,4-dihydroquinoline-3-carbohydrazide (7)
Yellow solid, yield: 90%.
IR (KBr): ν 3267–3067 (HN–C=O and H2N–N), 1670 (C=O hydrazide), 1630 (C=O, quinolone); 1H NMR (d6-DMSO, 500 MHz) δ (ppm): 13.50 (s, 1H, –CONH-12), 8.84 (s, 1H, H-2), 8.63 (s, 1H, –N=CH-14), 8.34 (dd, J = 8.0 and 1.0 Hz, 1H, H-5), 8.03 (d, J = 8.5 Hz, 2H, H-17 and H-19), 8.02 (d, J = 8.5 Hz, 2H, H-16 and H-20), 7.80 (td, J = 8.0 and 1.0 Hz, 1H, H-7), 7.75 (d, J = 8.5 Hz, 1H, H-8), 7.53 (td, J = 8.0 and 1.0 Hz, 1H, H-6); 13C NMR (d6-DMSO, 125 MHz) δ (ppm): 175.9 (C=O), 161.9 (C=ONH), 147.8 (CH-14), 147.5 (C0–18), 145.2 (CH-2), 140.9 (C0–15), 139.7 (C0–9), 132.9 (CH-7), 128.0 (CH-17 and CH-19), 127.8 (CH-16 and CH-20), 125.9 (C0–10), 125.4 (CH-6), 124.1 (CH-5), 119.7 (CH-8), 109.6 (C0–3); 15N (d6-DMSO, 60 MHz) δ (ppm) 145.40 (s, 1N, NH-1), 176.80 (s, 1H, –CONH-12), 329.70 (s, 1N, –N = C-13), 369.80 (s, 1N, NO2–21). TOF MS (ESI+) Calculated for C17H13N4O4 (M + H+): 337.0937. Found: 337.0963.
N′-[(1E)-(2-nitrophenyl)methylidene]-4-oxo-1,4-dihydroquinoline-3-carbohydrazide (8)
Yellow solid, yield: 84%.
IR (KBr): ν 3099–3029 (HN–C=O and H2N–N), 1672 (C=O hydrazide), 1608 (C=O, quinolone); 1H NMR (d6-DMSO, 500 M
(Hz) δ (ppm): 13.48 (s, 1H, –CONH), 8.86 (s, 1H, H-2), 8.73 (s, 1H, –N=CH-14), 8.30 (d, J = 7.5 Hz, 1H, H-5), 8.09 (d, J = 8.0 Hz, 1H, H-17), 8.07 (d, J = 8.0 Hz, 1H, H-20), 7.82 (t, J = 7.5 Hz, 2H, H-7 and H-18), 7.74 (t, J = 7.5 Hz, 1H, H-8), 7.68 (t, J = 7.5 Hz, 1H, H-19), 7.54 (t, J = 7.5 Hz, 1H, H-6); 13C NMR (d6-DMSO, 125 MHz) δ (ppm): 176.5 (C=O), 162.3 (C=ONH), 148.7 (C0–16), 145.0 (CH-14), 143.8 (CH-2) 139.5 (C0–9), 134.2 (CH-19), 133.7 (CH-7), 131.2 (CH-18), 129.2 (C0–15), 128.9 (CH-17), 126.3 (C0–10), 126.0 (CH-7), 125.9 (CH-5), 125.2 (CH-20), 119.7 (CH-8), 110.2 (C0–3); 15N (d6-DMSO, 60 MHz): δ (ppm) 143.40 (s, 1N, NH-1), 177.10 (s, 1H, –CONH-12), 325.29 (s, 1N, –N = C-13); TOF MS (ESI+) Calculated for C17H13N4O4 (M + H+): 337.0937. Found: 337.0965.
N′[(1E)-(4-methanesulfonylphenyl)methylidene]-4-oxo-1,4-dihydroquinoline-3-carbohydrazide (9)
Yellow solid, yield: 63%.
IR (KBr): ν 3261–3064 (HN–C=O and H2N–N), 1654 (C=O hydrazide), 1637 (C=O, quinolone); 1H NMR (d6-DMSO, 500 MHz) δ (ppm): 13.51 (s, 1H, –CONH-12), 8.89 (s, 1H, H-2), 8.56 (s, 1H, –N=CH-14), 8.35 (d, J = 8.1 Hz, 1H, H-5), 8.01 (s, 4H, H-16, H17, H19 and H20), 7.81 (td, J = 8.1 Hz, 1.0 Hz, 1H, H-7), 7.76 (d, J = 8.1 Hz, 1H, H-8), 7.54 (td, J = 8.1 and 1.0 Hz, 1H, H-6), 3.27 (s, 3H, CH3); 13C NMR (d6-DMSO, 125 MHz) δ (ppm): 176.5 (C=O), 162.2 (C=ONH), 146.3 (CH-14), 145.0 (CH-2), 141.8 (C0–18), 139.8 (C0–15), 139.6 (C0–9), 133.6 (CH-7), 128.2 (CH-17 and 19), 127.9 (CH-16 and 20), 126.3 (C0–9), 125.9 (CH-7), 125.9 (CH-6), 119.8 (CH-8), 110.3 (C0–3), 43.9 (CH3); TOF MS (ESI+) Calculated for C18H16N3O4S (M + H+): 370.0862. Found: 370.0889.
N′-[(1E)-[4-(methylsulfanyl)phenyl]methylidene]-4-oxo-1,4-dihydroquinoline-3-carbohydrazide (10)
White solid, yield: 85%.
IR (KBr): ν 3265–2920 (HN-C=O and H2N-N), 1670 (C=O hydrazide), 1630 (C=O, quinolone). 1H NMR (d6-DMSO, 500 MHz) δ (ppm): 13.33 (s, 1H, –CONH-12), 8.86 (s, 1H, H-2), 8.38 (s, 1H, –N=CH-14), 8.30 (dd, J = 8.1 and 0.9 Hz, 1H, H-5), 7.80 (td, 8.2 Hz and 1.0 Hz, 1H, H-7), 7.75 (dd, J = 8.2 and 1.0 Hz, 1H, H-8), 7.69 (d, J = 8.5 Hz, 2H, H-16 and H-20), 7.53 (td, 8.0 Hz and 0.9 Hz, 1H, H-6), 7.33 (d, J = 8.5 Hz, 2H, H-17 and H-19), 2.52 (s, 3H, CH3); 13C NMR (d6-DMSO, 125 MHz) δ (ppm): 175.9 (C=O), 161.9 (C=ONH), 147.6 (CH-14), 144.99 (CH-2), 141.3 (C0–18), 139.9 (C0–9), 131.4 (C0–15), 128.1 (CH-16 and 20), 126.4 (C0–10), 125.9 (CH-17 and 19), 125.7 (CH-5), 125.7 (CH-6), 133.4 (CH-7), 119.9 (CH-8), 110.5 (C0–3), 14.8 (CH3); TOF MS (ESI+) Calculated for C18H16N3O2S (M + H+): 338.0963. Found: 338.0988.
4-oxo-N′-[(1E)-thiophen-2-ylmethylidene]-1,4-dihydroquinoline-3-carbohydrazide (11)
White solid, yield: 79%.
IR (KBr): ν 3264–3058 (HN–C=O and H2N–N), 1645 (C=O hydrazide), 1620 (C=O, quinolone).
1H NMR (d6-DMSO, 500 MHz) δ (ppm): 13.20 (s, 1H, -CONH-12), 8.84 (s, 1H, H-2), 8.66 (s, 1H, –N=CH-14), 8.31 (d, J = 7.9 Hz, 1H, H-5), 7.80 (t, J = 8.0 Hz, 1H, H-7), 7.74 (d, J = 8.0 Hz, 1H, H-8), 7.68 (d, J = 4.0 Hz, 1H, H-16), 7.53 (t, J = 7.9 Hz, 1H, H-6), 7.47 (d, J = 2.4 Hz, 1H, H-18), 7.16 (d, J = 4.4 Hz, 1H, H-17); 13C NMR (d6-DMSO, 125 MHz) δ (ppm): 176.25 (C=O), 162.12 (C=ONH), 145.42 (CH-14), 143.19 (CH-2), 140.51 (C0–9), 139.55 (C0–15), 133.25 (CH-7), 131.47 (CH-16), 129.32 (CH-17), 128.37 (CH-18), 126.34 (C0–10), 125.86 (CH-5), 125.62 (CH-6), 120.40 (CH-8), 110.2 (C0–3); TOF MS (ESI+) Calculated for C15H12N3O2S (M + H+): 298.0650. Found: 298.0672.
N′-[(1E)-(4-methoxyphenyl)methylidene]-4-oxo-1,4-dihydroquinoline-3-carbohydrazide (12)
White solid, yield: 85%.
IR (KBr): ν 3267–2894 (HN–C=O and H2N–N), 1647 (C=O hydrazide), 1604 (C=O, quinolone); 1H NMR (d6-DMSO, 500 MHz) δ (ppm): 13.20 (s, 1H, –CONH-12), 12.98 (d, J = 6.1 Hz, 1H, –NH-1), 8.83 (d, J = 6.1 Hz, 1H, H-2), 8.34 (s, J = 1 Hz, –N=CH-14), 8.30 (d, J = 7.7 Hz, 1H, H-5), 7.81 (d, J = 8.2 Hz, 1H, H-7), 7.73 (d, J = 8.2 Hz, 3H, H-8, H-16 and H-20), 7.53 (t, J = 7.7 Hz, 1H, H-6), 7.02 (d, J = 8.5 Hz, 2H, H-17 and H-19), 3.80 (s, 3H, CH3); 13C NMR (d6-DMSO, 125 MHz) δ (ppm): 176.5 (C=O), 161.7 (C=ONH), 161.3 (C0–18), 148.1 (CH-2), 144.6 (CH-14), 139.5 (C0–9), 133.6 (CH-7), 129.4 (CH-16 and 20), 127.4 (C0–15), 126.3 (C0–10), 125.9 (CH-5), 125.8 (CH-6), 119.6 (CH-8), 114.8 (CH-17 and 19), 100.6 (C0–3), 55.8 (CH3). TOF MS (ESI+) Calculated for C18H16N3O3 (M + H+): 322.1192. Found: 322.1215.
Biology
Acetylcholinesterase activity
Acetylcholinesterase of electrophorus electricus (electric β-amyloid hypothesis in Alzheimer's disease: Seeing is believing.) – Type VI-S, acid 5,5′-dithio-bis(2-nitrobenzoic (DTNB), tacrine hydrochloride, 2,2-diphenyl-2-picrylhydrazyl hydrate (DPPH) and acetylthiocholine iodide (ATC) were acquired from Sigma-Aldrich (St. Louis, MO); dimethyl sulfoxide (DMSO) and ethanol from Labsynth (Brazil). Acetylcholinesterase (AChE) activity was evaluated by the quantitative Ellmańs colorimetric assay. Firstly, compounds were tested at 200 μM concentration and tacrine, a known AChE inhibitor, was used as positive control of inhibition. After 15 min of incubation at 25 °C, 30 μL of 0.8 mM acetylthiocholine iodide (ATC) were added to microplates containing the solution of acylhydrazones. The microplate was read at 415 nm every 30 s for 5 min (Microplate Reader Model 680, Bio-Rad Laboratories, Herts, UK). Experiments were performed in triplicate. AChE activities are expressed as % of inhibition.
Hydrazide 2 and acylhydrazone 9 inhibited the AChE activity in 22% and 36%, respectively. In turn, the other acylhydrazones showed an inhibition percentage ranging from 58 to 80%. For these compounds, the minimum concentration to inhibit the AChE activity in 50% (IC50) was calculated by nonlinear regression method.
Effect of acylhydrazones on the aβ fibril formation
Lyophilized Aβ42 peptide was purchased from American Peptide Co (Sunnyvale, CA). Films were prepared as previously reportedCitation1,Citation3 The effect of acylhydrazones on the Aβ42 fibrillization was evaluated by the thioflavin-T (ThT) fluorescence emission using a Tecan Infinite® (Männedorf, Switzerland) 200 PRO apparatus, at 450 nm and 485 nm, for excitation and emission, respectively. For this purpose, in a 96-well plate, 0.25 μL of a solution of the peptide film (0.166 mg in 6 μL of sterile DMSO), 0.25 μL of a 0.2 mM solution of acylhydrazone in sterile DMSO, 1.25 μL of 10 mM HCl, to induce fibril formation and 298.25 μL of a solution of ThT in phosphate buffer, pH 7.4 (0.05 mmol/L) were added. After mixing, samples were immediately analysed (t0) by fluorescence, and then, the microplate was incubated at 37 °C for 24 h and another aliquot was analysed (t24). A positive control of fibrillization was prepared in the same way as sample, but without adding test-compounds.
Results and discussion
Chemistry
In a previous workCitation3, quinolone 1 was prepared from a Morita–Baylis–Hillman adduct derived from 2-nitrobenzaldehyde. However, the methodology described by Moerdyk et al.Citation14, proved to be more effective and practical. Thus, 1 was obtained in 82% yield, through a condensation reaction involving diethyl (diethoxymethylene) malonate (DEM) and aniline, as described in the experimental section. After this, quinolone 1 was treated with hydrazine monohydrate to furnish hydrazide 2, as outlined in .
Figure 2. Preparation of the desired acylhydrazones: reagents and conditions. (a) aniline, Δ, 1 h; then, diphenyl ether, 120 °C, 24 h; 80% yield; (b) 50% aqueous NH2NH2, ethanol, Δ, 55 °C, 12 h; 82% yield; (c) aromatic aldehyde, ethanol, piperidine; Δ, 12–18 h; 63–90% yields.
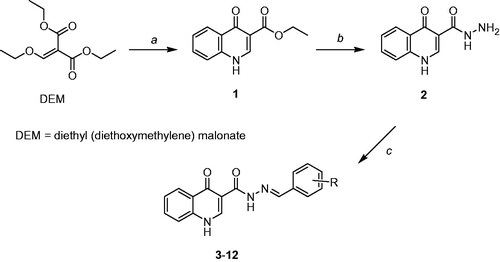
In order to accomplish our synthetic plan, hydrazide 2 was coupled with the appropriate aromatic aldehyde to produce acylhydrazones 3–12 in 63 to 90% yields, as presented in .
Table 1. Synthesized acylhydrazones and chemical yields.
The synthesized acylhydrazones were characterized by spectroscopic methods, including infrared (IR), 1H and 13C NMR spectroscopy. The 1H-NMR spectra showed some duplicated signals. According to the literatureCitation15, such duplication is due to the presence of rotamers of the amide bond. To confirm this statement, we conducted 1H NMR experiments at higher temperatures. An example of expanded spectrum is presented in . At room temperature (), we observed a duplication of the peaks attributed to H-2, H-12 and H-14 absorptions. As the temperature rises (), the interconversion between the conformational isomers derived from the rotation around the amide bond occurs. In our study, a complete coalescence of the signals was reached at 120 °C ().
Analytical data of the synthesized compounds and full spectra are available in the experimental section and supplementary material, respectively.
Acetylcholinesterase activity
Acetylcholinesterase is a serine hydrolaseCitation11 involved in the pathology of AD in two ways. Firstly, this enzyme is responsible for the hydrolysis of acetylcholine, which plays an important role in the cognitive process, such as memory and learningCitation16,Citation17. Additionally, the peripheral site in the AChE structureCitation18,Citation19 has been implicated in the amyloidogenic processCitation20. These evidences have been contributing for the development of compounds able to interact with the catalytic site and/or the peripheral oneCitation21.
The great interest in AChE inhibitorsCitation22,Citation23 motivated us to study the effect of hydrazide 2 and acylhydrazones 3–12 on the acetylcholinesterase (AChE) activity. After complete characterization, the synthesized compounds were evaluated by the quantitative Ellman’s colorimetric assayCitation24, as detailed in the experimental section. Hydrazide 2 and the acylhydrazone derived from 4-methylsulphonylbenzaldehyde 9 impairs the AChE activity in 36 and 44%, respectively, at the maximum concentration (200 μM). In contrast, the other acylhydrazones inhibited the enzyme in a significant way, even at the lowest concentration. shows a comparative graph of % inhibition of AChE promoted by the acylhydrazones 3–8, 10–12 and tacrine, at the minimum concentration (12.5 μM).
The minimum concentration to inhibit AChE in 50% (IC50) was determined for the most active compounds. In order to do this, they were tested at four different concentrations, that is, 200, 100, 50, 25 and 12.5 μmol/L and IC50 was calculated using nonlinear regression by the software GraphPad PrismaCitation25. Acylhydrazones inhibited AChE in a significant way, with IC50 values ranging from 1.2 to 17 μM as summarized in .
Table 2. Results of the inhibition acetylcholinesterase by acylhydrazones.
In general, we can state that the nature of the substituent of the C-3 in the quinolonic ring influences on the inhibitory effect on the AChE. When acylhydrazone derivatives are compared to the quinolone 1 and hydrazide 2, we found a remarkable inhibitory effect. This observation may be due a number of properties, including size and shape. The electronic nature of the aromatic substituent cannot be directly related to the observed activity.
Effect on the Aβ42 fibrils formation
The abnormal cleavage of amyloid protein precursor (APP) promoted by β and γ-secretases is one of the most important events in AD, leading to the formation of toxic peptides, especially the one containing 42 aminoacid residues, known as Aβ42Citation26,Citation27. This peptide triggers a self-aggregation and fibril formation processes. Thus, compounds having antiaggregation and antifibrillization properties have been studied as potential drug candidates to treat ADCitation28–30. In this context, acylhydrazones 3–8 and 10–12 were tested for their antifibrillization effect. For this purpose, commercially available Aβ42 peptide was incubated with each acylhydrazone, in the presence of 10 mM HCl to induce fibril formationCitation2. Details are provided in the experimental section. Thioflavin-T (ThT) binds to Aβ fibrils causing an increase in its fluorescence emissionCitation31. Here, we selected this method to monitor the fibril formation. Measurements of ThT fluorescence emission are taken at the beginning of experiment (t0) and after 24 h of incubation (t24). A positive control of fibrillization was prepared by mixing Aβ42 peptide and HCl, in the absence of acylhydrazones. Results are presented in .
Figure 5. Effect of acylhydrazones (3–8 and 10–12) on the ThT fluorescence emission at the beginning of the experiment (t = 0) and after 24 h of incubation at 37 °C (t = 24 h). Control represents the Aβ42 peptide in the presence of HCl, without acylhydrazones.
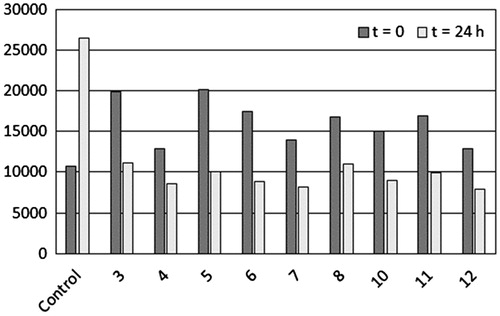
As expected, after 24 h of incubation an increase in the ThT fluorescence emission was observed in the control, due to fibrils formation. Meanwhile, after 24 h, the emission of fluorescence in samples containing acylhydrazones decreased at least 49% (acylhydrazone 5) and a maximum inhibitory effect was promoted by acylhydrazone 8 (67%).
These observations associated to the inhibitory effect on AChE, make these synthesized acylhydrazones very promising dual-targeting prototypes for the development of AD drug candidates.
Conclusion
We synthesized 10 acylhydrazones having a 4-quinolone moiety, and nine of them are not reported in literature. These compounds were obtained in three steps from 2-nitrobenzaldehyde in moderate to good yields. Acylhydrazones were fully characterized by spectroscopic methods, and experiments to evaluate their effect on the acetylcholinesterase activity and Aβ42 fibrillization were conducted. We found that the ability of the majority of acylhydrazones in inhibiting AChE is interesting, with IC50 values ranging from 1.2 to 17 μM. These results encourage us to search more potent analogues. With respect to the effect on the Aβ fibrils formation, except for acylhydrazone 9, the studied compounds have an impressive inhibitory effect on the formation of Aβ42 fibrils. These findings encourage us to continue the search for more potent analogues.
Supplementary material available online
IENZ_1144597_Supplementary_material.pdf
Download PDF (1.2 MB)Acknowledgements
Authors are grateful to the São Paulo Foundation for Science (Fapesp, Proc. 2013/18203–5) and CNPq for GSS and MF fellowship.
Declaration of interest
The authors report no conflicts of interest. The authors alone are responsible for the content and writing of this article.
References
- Kung HF. The β-amyloid hypothesis in Alzheimer's disease: seeing is believing. ACS Med Chem Lett 2012;3:265–7
- Dahlgren KK, Manelli AM, Stine WB Jr, et al. Oligomeric and fibrillar species of amyloid-beta peptides differentially affect neuronal viability. J Biol Chem 2002;277:32046–53
- Huber PC, Reis GP, Amstalden MC, Lancellotti M. Synthesis, spectroscopic characterizations and antimicrobial activity of copper and zinc complexes of levofloxacin, ciprofloxacin and 3-carboethoxy-quinolone. Polyhedron 2013;57:14–19
- Huber PC, Benevento CE, Castilho RF, Almeida WP. Effect of levofloxacin and 3-carboethoxy-4-quinolone on the β-amyloid fibrils formation. J Chem Pharm Res 2015;7:993–1000
- Pudlo M, Luzet V, Ismaïli L, et al. Quinolone-benzylpiperidine derivatives as novel acetylcholinesterase inhibitor and antioxidante hybrids for Alzheimer disease. Biooorg Med Chem 2014;22:2496–507
- Le Goff G, Ouazzani J. Natural hydrazine-containing compounds: biosynthesis, isolation, biological activities and synthesis. Bioorg Med Chem 2014;22:6529–44
- Maia RD, Tesch R, Fraga CAM. Acylhydrazone derivatives: a patent review. Expert Opin Ther Pat 2014;24:1161–70
- Mohareb RM, Fleita DH, Sakka OK. Novel synthesis of hydrazine-hydrazone derivatives and their utilization in the synthesis of coumarin, pyridine, thizole and thiophene derivatives with antitumor activity. Molecules 2011;16:16–27
- Ellis S, Kalinowski DS, Leotta L, et al. Potent antimycobacterial activity of the pyridoxal isonicotinoyl hydrazone: a lipophilic transport vehicle for isonicotinic acid hydrazide. Mol Pharmacol 2014;85:269–78
- Navidpour L, Shafaroodi H, Saeedi-Motahar G, Shafiee A. Synthesis, anti-inflammatory and analgesic activities of arylidene-2-(3-chloroanilino)nicotinic acid hydrazyde. Med Chem Res 2014;23:2793–802
- Massoulié J, Bon S. The C-terminal T peptide of cholinesterases: structure, interactions, and influence on protein folding and secretion. J Mol Neurosci 2006;30:233–6
- Amarante GW, Benassi M, Pascoal RN, et al. Mechanism and synthesis of pharmacologically active quinolones from Morita–Baylis–Hillman adducts. Tetrahedron 2010;66:4370–6
- Srivatava N, Kumar A. Synthesis of substituted-4-oxo-1, 4-dihydro-3-[1-oxo-2-hydrazino-3-{p-toluenesulfon}] quinoline derivatives and their biological activity against bacterial infections. Orient J Chem 2013;29:507–11
- Moerdyk JO, Speelman AL, Kuper III KE, et al. Synthesis and photochemistry of two quinolines analogs of the perimidinespirohexadienone family of photochromes. J Photochem Photobiol A 2009;205:84–92
- Lopez AB, Miguez E, Kummerle AE, et al. Characterization of amide bond conformers for a novel heterocyclic template of N-acylhydrazone derivatives. Molecules 2013;18:11683–704
- Blake MG, Krawczyk MC, Baratti CM, Boccia MM. Neuropharmacology of memory consolidation and reconsolidation: Insights on central cholinergic mechanisms. J Physiol Paris 2014;108:286–91
- Lombardo S, Maskos U. Role of the nicotinic acetylcholine receptor in Alzheimer's disease pathology and treatment. Neuropharmacology 2014;96:255–62
- Johnson G, Moore SW. The peripheral anionic site of acetylcholinesterase: structure, functions and potential role in rational drug design. Curr Pharm Des 2006;12:217–25
- Dikersont TJ, Beuscher IV AE, Rogers CJ, et al. Discovery of acetylcholinesterase peripheral anionic site ligands through computational refinement of a directed library. Biochemistry 2005;44:14845–3
- Inestros NC, Dinamarca MC, Alvarez A. Amyloid-cholinesterase interactions. Implications for Alzheimer's disease. FEBS J 2008;275:625–32
- Kryger G, Silman I, Sussman JL. Structure of acetylcholinesterase complexed with E2020 (Aricept): implications for the design of new anti-Alzheimer drugs. Structure 1999;7:297–307
- Prakash A, Kalra J, Mani V, et al. Pharmacological approaches for Alzheimer's disease: neurotransmitter as drug targets. Expert Rev Neurother 2015;15:53–71
- Singh M, Kaur M, Kukreja H, et al. Acetylcholinesterase inhibitors as Alzheimer therapy: from nerve toxins to neuroprotection. Eur J Med Chem 2013;70:165–88
- Ellman GL. Tissue sulfhydryl groups. Arch Biochem Biophys 1959;82:70–7
- GraphPad Prism version 6.04 for Windows, GraphPad Software. La Jolla, CA. Available from: www.graphpad.com
- Multhaup G, Huber O, Buee L, Galas MC. Amyloid precursor protein (APP), metabolites APP, intracellular fragment (AICD), a beta 42 and Tau in nuclear roles. J Biol Chem 2015;39:23515–22
- Puzzo D, Gulisano W, Arancio O, Palmeri A. The keystone of Alzheimer pathogenesis might be sought in Aβ physiology. Neuroscience 2015;307:26–36
- Karran E, Mercken M, De Strooper B. The amyloid cascade hypothesis for Alzheimer's disease: an appraisal for the development of therapeutics. Nat Rev Drug Discov 2011;10:698–712
- Mohamed T, Rao PPN. Alzheimer's disease: emerging trends in small molecule therapies. Curr Med Chem 2011;18:4299–320
- Trippier PC, Labby KJ, Hawker DD, Mataka JJ. Target- and mechanism-based therapeutics for neurodegenerative diseases: strength in numbers. J Med Chem 2013;56:3121–47
- Krebs MRH, Bromley EHC, Donald AM. The binding of thioflavin-T to amyloid fibrils: localisation and implications. J Struct Biol 2005;149:30–7