Abstract
Synthesis, characterization and investigation of in vivo anticonvulsant activities of 13 novel cyclopentanecarbaldehyde-based 2,4-disubstituted 1,3-thiazoles are presented. Their structures were determined using 1H and 13C NMR, FAB(+)-MS, HRMS and elemental analyses. The results of anticonvulsant screening reveal that seven intraperitoneally administered compounds: 3a, 3b, 3d, 3e, 3f, 3k and 3m containing F-, Cl-, Br-, CF3-, CH3- and adamantyl substituents demonstrated significant anticonvulsant activity in the pentylenetetrazole model with median effective doses (ED50) ≤ 20 mg/kg, respectively, which was approximately seven-fold lower than that reported for the reference drug, ethosuximide. Noteworthy, none of these compounds impaired animals’ motor skills in the rotarod test.
Introduction
Epilepsy is a chronic neurological disorder characterized by recurrent and unprovoked seizure episodes. Although numerous clinically effective antiepileptic drugs (AEDs) are available, it is estimated that ∼30% of epileptic patients are drug-resistant. Furthermore, adverse effects and poor tolerability of so-called first- and second-generation of AEDs necessitate the search for novel anticonvulsant active agentsCitation1–3.
Thiazole derivatives are among of the most intensively studied classes of aromatic five-membered heterocycles because of their diverse pharmacological activities. Examples of antifungal (ravuconazole)Citation4, antineoplastic (tiazofurin)Citation5, anti-HIV (ritonavir)Citation6, antiparasitic (nitazoxanide)Citation7, as well as anti-inflammatory drugs (fentiazac, meloxicam)Citation8 and MAO inhibitorsCitation9,Citation10 can be found in this group.
Finally, it has been demonstrated that 3-(4-(4-bromophenyl)-1,3-thiazol-2-ylamino)-4-(2-methylphenyl)-4,5-dihydro-1H-1,2,4-triazole-5-thione showed high anticonvulsant activity in the maximal electroshock seizure (MES) model and in pentylenetetrazole (PTZ) test with median effective doses (ED50) of 13.4 mg/kg and 81.6 mg/kg, respectivelyCitation11. Also imidazolyl derivative, 1-((2-(4-chlorophenyl)thiazol-4-yl)methyl)-1H-imidazole and triazolyl derivative, 1-((2-phenylthiazol-4-yl)methyl)-1H-1,2,4-triazole showed the highest anticonvulsant activity in both MES and PTZ models with protection range of 33–100%Citation12. Several triazolyl-thiazole derivatives were obtained and tested as anticonvulsant agents in the PTZ model in mice. The results showed that these derivatives protect from seizures and reduce mortality rate in this modelCitation13.
Considering that the thiazole derivatives can exhibit various biological properties, and continuing our previous investigationCitation14–18 we decided to incorporate cyclopentylmethylidene moiety into the thiazol-2-yl-hydrazine pharmacophore containing various electron-donating and electron-withdrawing substituents. These substituents are useful to modulate electronic effects, and may influence the hydrophilic and hydrophobic properties of the molecules.
Consequently, we prepared 13 novel thiazole derivatives and evaluated their in vivo anticonvulsant properties in mouse models of chemically and electrically induced seizures. Additionally, the effect of the test compounds on animals’ motor coordination was assessed using the rotarod test.
Experimental
Materials and methods
All experiments were carried out under air atmosphere unless stated otherwise. Reagents were generally the best quality commercial-grade products and were used without further purification. 1H NMR (700 and 400 MHz) and 13C NMR (100 MHz) spectra were recorded on a Bruker Avance III multinuclear instrument (Toruń, Poland). FAB(+)-MS and HRMS analyses were performed by the Laboratory for Analysis of Organic Compounds and Polymers of the Centre for Molecular and Macromolecular Studies of the Polish Academy of Sciences in Łódź, Poland. MS spectra were recorded on a Finnigan MAT 95 spectrometer. Elemental analysis was performed on ELEMENTAR Vario MACRO CHN (Toruń, Poland). Melting points were determined in open glass capillaries and are uncorrected. Analytical TLC was performed using Macherey-Nagel Polygram Sil G/UV254 0.2 mm plates (Sigma Aldrich, Poznań, Poland). Cyclopentanecarbaldehyde, ethyl 4-chloro-3-oxobutanoate, ethyl 2-chloro-3-oxobutanoate, 1-chloropropan-2-one and appropriate bromoketones were commercial materials (Sigma Aldrich, Poznań, Poland).
(E)-2-(Cyclopentylmethylidene)hydrazinecarbothioamide (2)
Thiosemicarbazide (1.86 g, 20.38 mmol) was added to a stirred solution of cyclopentanecarbaldehydeCitation1 (2.00 g, 20.38 mmol) in absolute ethyl alcohol (20 ml) and then acetic acid (1 ml) was added. The reaction mixture was stirred under reflux for 20 h. After that, the reaction mixture was poured on the crushed ice and kept aside. The product was filtered off and subsequently washed with water to yield 2.72 g, 78%; mp 76–78 °C; eluent: dichloromethane/methanol (90:10), Rf = 0.73. 1H NMR (DMSO-d6); δ (ppm); 1.45–1.68 (m, 6H, 3CH2); 1.69–1.82 (m, 2H, CH2); 2.57–2.68 (m, 1H, CH); 7.36 (d, 1H, CH, J = 6.0 Hz); 7.41 (s, 1H, NH); 7.94 (s, 1H, NH); 10.99 (bs, 1H, NH). 13C NMR (DMSO-d6, 100 MHz), δ (ppm): 25.39 (2C); 30.39 (2C); 42.28; 151.01; 178.06. Anal. Calcd. for C7H12N2S: C, 49.09; H, 7.65; N, 24.54. Found: C, 49.11; H, 7.67; N, 24.56.
(E)-2-(2-(Cyclopentylmethylene)hydrazinyl)-4-(4-fluorophenyl)thiazole (3a). Typical procedure
Carbothioamide 2 (0.171 g, 1.0 mmol) was added to a stirred solution of 2-bromo-1-(4-fluorophenyl)ethanone (0.217 g, 1.0 mmol) in absolute ethyl alcohol (10 ml). The reaction mixture was stirred at room temperature for 20 h. The separated precipitate was purified on silica gel column chromatography (230–400 mesh) using (dichloromethane/methanol, 90:10, Rf = 0.77) to afford the desired product: 0.18 g, 62%; mp 145–146 °C. 1H NMR (DMSO-d6), δ (ppm): 1.45–1.70 (m, 6H, 3CH2); 1.73–1.88 (m, 2H, CH2); 2.60–2.73 (m, 1H, CH); 7.16–7.26 (m, 3H, 3CH); 7.31 (d, 1H, CH, J = 6.0 Hz); 7.81–7.89 (m, 2H, 2CH); 11.54 (bs, 1H, NH). 13C NMR (DMSO-d6, 100 MHz), δ (ppm): 25.32 (2C); 30.62 (2C); 42.44; 103.08; 115.82 (d, 2C, J = 21.0 Hz); 127.89 (d, 2C, J = 8.0 Hz); 131.92; 149.61; 160.78; 163.21; 169.11. FAB(+)-MS (m/z, %): 290.2 [(M++1), 100], 185.1 (20). HRMS (m/z) calculated for C15H16FN3S: 289.1049. Found: 289.1052. Anal. Calcd. for C15H16FN3S: C, 62.26; H, 5.57; N, 14.52. Found: C, 62.24; H, 5.56; N, 14.55.
(E)-4-(4-Chlorophenyl)-2-(2-(cyclopentylmethylene)hydrazinyl)thiazole (3b)
2-Bromo-1-(4-chlorophenyl)ethanone was reacted with 2. Yield: 0.12 g, 38%, (dichloromethane/methanol, 90:10, Rf = 0.86); mp 155–156 °C. 1H NMR (DMSO-d6), δ (ppm): 1.47–1.70 (m, 6H, 3CH2); 1.74–1.86 (m, 2H, CH2); 2.61–2.73 (m, 1H, CH); 7.29 (s, 1H, CH); 7.34 (d, 1H, CH, J= 6.0 Hz); 7.45 (d, 2H, 2CH, J = 9.0 Hz); 7.83 (d, 2H, 2CH, J = 9.0 Hz); 11.60 (bs, 1H, NH). 13C NMR (DMSO-d6, 100 MHz), δ (ppm): 25.33 (2C); 30.55 (2C); 42.44; 104.45; 127.81 (2C); 129.07 (2C); 132.61; 133.19; 148.12; 151.16; 169.21. FAB(+)-MS (m/z, %): 306.2 [(M++1), 100], 212.0 (15), 210.1 (40). HRMS (m/z) calculated for C15H16ClN3S: 305.0753. Found: 305.0760. Anal. Calcd. for C15H16ClN3S: C, 58.91; H, 5.27; N, 13.74. Found: C, 58.90; H, 5.28; N, 13.76.
(E)-2-(2-(Cyclopentylmethylene)hydrazinyl)-4-(4-methoxyphenyl)thiazole (3c)
2-Bromo-1-(4-methoxyphenyl)ethanone was reacted with 2. Yield: 0.25 g, 82%, (dichloromethane/methanol, 95:5, Rf = 0.83); mp 109–112 °C. 1H NMR (DMSO-d6), δ (ppm): 1.45–1.70 (m, 6H, 3CH2); 1.75–1.85 (m, 2H, CH2); 2.60–2.73 (m, 1H, CH); 3.78 (s, 3H, CH3); 6.94 (d, 2H, 2CH, J= 9.0 Hz); 7.03 (s, 1H, CH); 7.30 (d, 1H, CH, J = 6.0 Hz); 7.75 (d, 2H, 2CH, J = 9.0 Hz); 11.50 (bs, 1H, NH). 13C NMR (DMSO-d6, 100 MHz), δ (ppm): 25.33 (2C); 30.63 (2C); 42.43; 55.58; 101.19; 114.38 (2C); 127.27 (2C); 128.13; 149.47; 150.53; 159.19; 168.90. FAB(+)-MS (m/z, %): 302.3 [(M++1), 100], 232.1 (20), 206.1 (30). HRMS (m/z) calculated for C16H19N3OS: 301.1249. Found: 301.1239. Anal. Calcd. for C16H19N3OS: C, 63.76; H, 6.35; N, 13.94. Found: C, 63.79; H, 6.33; N, 13.95.
(E)-4-(4-Bromophenyl)-2-(2-(cyclopentylmethylene)hydrazinyl)thiazole (3d)
2-Bromo-1-(4-bromophenyl)ethanone was reacted with 2. Yield: 0.23 g, 66%, (dichloromethane/methanol, 90:10, Rf = 0.84); mp 155–156 °C. 1H NMR (DMSO-d6), δ (ppm): 1.45–1.71 (m, 6H, 3CH2); 1.74–1.87 (m, 2H, CH2); 2.61–2.73 (m, 1H, CH); 7.30 (s, 1H, CH); 7.33 (d, 1H, CH, J = 6.0 Hz); 7.58 (d, 2H, 2CH, J = 9.0 Hz); 7.77 (d, 2H, 2CH, J = 9.0 Hz); 11.60 (bs, 1H, NH). 13C NMR (DMSO-d6, 100 MHz), δ (ppm): 25.60 (2C); 30.69 (2C); 42.44; 104.51; 121.16; 128.10 (2C); 131.98 (2C); 133.67; 148.34; 151.04; 169.20. FAB(+)-MS (m/z, %): 350.2 [(M++1), 100], 256.1 (15), 174.1 (30). HRMS (m/z) calculated for C15H16BrN3S: 349.0248. Found: 349.0238. Anal. Calcd. for C15H16BrN3S: C, 51.43; H, 4.60; N, 12.00. Found: C, 51.42; H, 4.60; N, 12.03.
(E)-2-(2-(Cyclopentylmethylene)hydrazinyl)-4-(4-(trifluoromethyl)phenyl)thiazole (3e)
2-Bromo-1-(4-trifluoromethylphenyl)ethanone was reacted with 2. Yield: 0.16 g, 49%, (dichloromethane/methanol, 95:5, Rf = 0.74); mp 146–147 °C. 1H NMR (DMSO-d6), δ (ppm): 1.46–1.70 (m, 6H, 3CH2); 1.75–1.86 (m, 2H, CH2); 2.61–2.73 (m, 1H, CH); 7.34 (d, 1H, CH, J = 6.0 Hz); 7.47 (s, 1H, CH); 7.74 (d, 2H, 2CH, J = 8.0 Hz); 8.03 (d, 2H, 2CH, J = 8.0 Hz); 11.60 (bs, 1H, NH). 13C NMR (DMSO-d6, 100 MHz), δ (ppm): 25.32 (2C); 30.56 (2C); 42.43; 106.29; 125.99 (m, 2C); 126.54 (2C); 128.03 (q, J = 32.0 Hz); 128.87; 138.56; 148.65; 150.51; 169.31. FAB(+)-MS (m/z, %): 340.3 [(M++1), 100], 244.2 (15). HRMS (m/z) calculated for C16H16F3N3S: 339.1017. Found: 339.1016. Anal. Calcd. for C16H16F3N3S: C, 56.62; H, 4.75; N, 12.38. Found: C, 56.59; H, 4.77; N, 12.40.
(E)-2-(2-(Cyclopentylmethylene)hydrazinyl)-4-p-tolylthiazole (3f)
2-Bromo-1-(4-methylphenyl)ethanone was reacted with 2. Yield: 0.24 g, 85%, (dichloromethane/methanol, 95:5, Rf = 0.74); mp 156–157 °C. 1H NMR (DMSO-d6), δ (ppm): 1.48–1.70 (m, 6H, 3CH2); 1.75–1.86 (m, 2H, CH2); 2.32 (s, 3H, CH3); 2.62–2.74 (m, 1H, CH); 7.16 (s, 1H, CH); 7.21 (d, 2H, 2CH, J = 8.0 Hz); 7.39 (d, 1H, CH, J = 6.0 Hz); 7.69 (d, 2H, 2CH, J = 8.0 Hz); 11.50 (bs, 1H, NH). 13C NMR (DMSO-d6, 100 MHz), δ (ppm): 21.29; 25.36 (2C); 30.49 (2C); 42.46; 103.11; 126.21 (2C); 129.70 (2C); 138.07; 147.37; 150.88; 152.86; 169.16. FAB(+)-MS (m/z, %): 286.3 [(M++1), 100], 190.1 (15). HRMS (m/z) calculated for C16H19N3S: 285.1300. Found: 285.1303. Anal. Calcd. for C16H19N3S: C, 67.33; H, 6.71; N, 14.72. Found: C, 67.31; H, 6.70; N, 14.75.
(E)-4-(2-(2-(Cyclopentylmethylene)hydrazinyl)thiazol-4-yl)benzonitrile (3g)
4-(Bromoacetyl)benzonitrile was reacted with 2. Yield: 0.26 g, 87%, (dichloromethane/methanol, 95:5, Rf = 0.76); mp 151–153 °C. 1H NMR (DMSO-d6), δ (ppm): 1.46–1.71 (m, 6H, 3CH2); 1.74–1.86 (m, 2H, CH2); 2.61–2.73 (m, 1H, CH); 7.34 (d, 1H, CH, J = 6.0 Hz); 7.54 (s, 1H, CH); 7.85 (d, 2H, 2CH, J = 9.0 Hz); 8.00 (d, 2H, 2CH, J = 8.0 Hz); 11.60 (bs, 1H, NH). 13C NMR (DMSO-d6, 100 MHz), δ (ppm): 25.32 (2C); 30.57 (2C); 42.44; 107.33; 109.99; 119.44; 126.58 (2C);132.81; 133.10 (2C); 139.13; 150.39; 169.32. FAB(+)-MS (m/z, %): 297.2 [(M++1), 100], 201.1 (15). HRMS (m/z) calculated for C16H16N4S: 296.1096. Found 296.1096. Anal. Calcd. for C16H16N4S: C, 64.84; H, 5.44; N, 18.90. Found: C, 64.81; H, 5.46; N, 18.91.
(E)-2-(2-(Cyclopentylmethylene)hydrazinyl)-4-(4-nitrophenyl)thiazole (3h)
2-Bromo-1-(4-nitrophenyl)ethanone was reacted with 2. Yield: 0.24 g, 75%, (dichloromethane/methanol, 95:5, Rf = 0.54); mp 140–144 °C. 1H NMR (DMSO-d6), δ (ppm): 1.46–1.71 (m, 6H, 3CH2); 1.74–1.87 (m, 2H, CH2); 2.60–2.74 (m, 1H, CH); 7.34 (d, 1H, CH, J = 6.0 Hz); 7.62 (s, 1H, CH); 8.08 (d, 2H, 2CH, J = 9.0 Hz); 8.26 (d, 2H, 2CH, J = 9.0 Hz); 11.70 (bs, 1H, NH). 13C NMR (DMSO-d6, 100 MHz), δ (ppm): 25.33 (2C); 30.58 (2C); 42.44; 108.33; 124.53 (2C); 126.76 (2C); 141.22; 146.62; 148.75; 150.23; 169.41. FAB(+)-MS (m/z, %): 317.3 [(M++1), 100], 301.3 (30), 147.1 (30). HRMS (m/z) calculated for C15H16N4O2S: 316.0994. Found: 316.0986. Anal. Calcd. for C15H16N4O2S: C, 56.94; H, 5.10; N, 17.71. Found: C, 56.92; H, 5.09; N, 17.72.
(E)-2-(2-(Cyclopentylmethylene)hydrazinyl)-4-(3,4-dichlorophenyl)thiazole (3i)
2-Bromo-1-(3′4′-dichlorophenyl)ethanone was reacted with 2. Yield: 0.22 g, 65%, (dichloromethane/methanol, 95:5, Rf = 0.81); mp 149–150 °C. 1H NMR (DMSO-d6), δ (ppm): 1.45–1.70 (m, 6H, 3CH2); 1.73–1.86 (m, 2H, CH2); 2.60–2.73 (m, 1H, CH); 7.32 (d, 1H, CH, J= 6.0 Hz); 7.44 (s, 1H, CH); 7.65 (d, 1H, CH, J = 8.0 Hz); 7.80 (dd, 1H, CH, J1 = 2.0 Hz; J2= 8.0); 8.04 (d, 1H, CH, J= 2.0 Hz); 11.50 (bs, 1H, NH). 13C NMR (DMSO-d6, 100 MHz), δ (ppm): 25.32 (2C); 30.58 (2C); 40.43; 105.67; 126.01; 127.60; 130.06; 131.28; 131.84; 135.67; 147.99; 150.19; 169.24. FAB(+)-MS (m/z, %): 240.2 [(M++1), 100]. HRMS (m/z) calculated for C15H15Cl2N3S: 339.0364. Found: 339.0352. Anal. Calcd. for C15H15Cl2N3S: C, 52.95; H, 4.44; N, 12.35. Found: C, 52.92; H, 4.41; N, 12.35.
(E)-Ethyl 2-(2-(2-(cyclopentylmethylene)hydrazinyl)thiazol-4-yl)acetate (3j)
Ethyl 4-chloro-3-oxobutanoate was reacted with 2. Yield: 0.14 g, 39%, (dichloromethane/methanol, 95:5, Rf = 0.50); mp 109–110 °C. 1H NMR (DMSO-d6), δ (ppm): 1.18 (t, 3H, CH3, J = 7.0 Hz); 1.43–1.68 (m, 6H, 3CH2); 1.73–1.83 (m, 2H, CH2); 2.60–2.71 (m, 1H, CH); 3.53 (s, 2H, CH2); 4.07 (q, 2H, CH2, J = 7.0 Hz); 6.53 (s, 1H, CH); 7.24 (d, 1H, CH, J = 6.0 Hz); 11.35 (bs, 1H, NH). 13C NMR (DMSO-d6, 100 MHz), δ (ppm): 14.56; 25.30 (2C); 30.62 (2C); 37.54; 42.38; 60.64; 105.15; 145.30; 149.30; 168.85; 170.50. FAB(+)-MS (m/z, %): 282.3 [(M++1), 100]. HRMS (m/z) calculated for C13H19N3O2S: 281.1198. Found: 295.1191. Anal. Calcd. for C13H19N3O2S: C, 55.49; H, 6.81; N, 14.93. Found: C, 55.52; H, 6.79; N, 14.95.
(E)-Ethyl 2-(2-(cyclopentylmethylene)hydrazinyl)-4-methylthiazole-5-carboxylate (3k)
Ethyl 2-chloro-3-oxobutanoate was reacted with 2. Yield: 0.18 g, 68%, (dichloromethane/methanol, 95:5, Rf = 0.59); mp 143–147 °C. 1H NMR (DMSO-d6), δ (ppm): 1.25 (t, 3H, CH3, J = 7.0 Hz); 1.47–1.68 (m, 6H, 3CH2); 1.74–1.85 (m, 2H, CH2); 2.44 (s, 3H, CH3); 2.61–2.74 (m, 1H, CH); 4.18 (q, 2H, CH2, J = 7.0 Hz); 7.38 (d, 1H, CH, J = 6.0 Hz); 11.93 (bs, 1H, NH). 13C NMR (DMSO-d6, 100 MHz), δ (ppm): 14.76; 17.71; 25.32 (2C); 30.41 (2C); 42.41; 60.42; 112.29; 152.60; 162.43; 169.89. FAB(+)-MS (m/z, %): 282.3 [(M++1), 100]. HRMS (m/z) calculated for C13H19N3O2S: 281.1198. Found: 281.1193. Anal. Calcd. for C13H19N3O2S: C, 55.49; H, 6.81; N, 14.93. Found: C, 55.47; H, 6.80; N, 14.95.
(E)-2-(2-(Cyclopentylmethylene)hydrazinyl)-4-methylthiazole (3l)
1-Chloropropan-2-one was reacted with 2. Yield: 0.15 g, 72%, (dichloromethane/methanol, 95:5, Rf = 0.55); mp 70–75 °C. 1H NMR (DMSO-d6), δ (ppm): 1.44–1.67 (m, 6H, 3CH2); 1.73–1.84 (m, 2H, CH2); 2.12 (s, 3H, CH3); 2.57–2.69 (m, 1H, CH); 6.27 (s, 1H, CH); 7.25 (d, 1H, CH, J = 6.0 Hz); 11.17 (bs, 1H, NH). 13C NMR (DMSO-d6, 100 MHz), δ (ppm): 17.65; 25.31 (2C); 30.65 (2C); 42.41; 102.15; 148.00; 149.20; 168.63. FAB(+)-MS (m/z, %): 210.2 [(M++1), 100]. HRMS (m/z) calculated for C10H15N3S: 209.0987. Found: 209.0990. Anal. Calcd. for C10H15N3S: C, 57.38; H, 7.22; N, 20.08. Found: C, 57.35; H, 7.20; N, 20.10.
(E)-2-(2-(Cyclopentylmethylene)hydrazinyl)-4-(adamant-1-yl)thiazole (3m)
1-Adamantyl bromomethyl ketone was reacted with 2. Yield: 0.24 g, 74%, (dichloromethane/methanol, 95:5, Rf = 0.44); mp 153–154 °C. 1H NMR (DMSO-d6), δ (ppm): 1.48–1.68 (m, 11H, 6CH2); 1.68–1.76 (m, 2H, CH2); 1.76–1.90 (m, 7H, 4CH2); 1.99–2.05 (m, 3H, 3CH); 2.66–2.78 (m, 1H, CH); 6.52–6.55 (m, 1H, CH); 7.63–7.70 (m, 1H, CH); 12.05 (bs, 1H, NH). 13C NMR (DMSO-d6, 100 MHz), δ (ppm): 25.38 (2C); 28.09 (3C); 30.41 (2C); 35.82 (3C); 36.39 (3C); 40.80; 42.43; 101.32; 154.46; 156.05; 169.28. FAB(+)-MS (m/z, %): 230.3 [(M++1), 100]. HRMS (m/z) calculated for C19H27N3S: 329.1926. Found: 329.1935. Anal. Calcd. for C19H27N3S: C, 69.26; H, 8.26; N, 12.75. Found: C, 69.30; H, 8.29; N, 12.78.
In vivo pharmacology
Animals and housing conditions
Adult male Albino Swiss (CD-1) mice weighing between 18 and 22 g were used for in vivo tests. The animals were housed in groups of 10 mice per cage at room temperature of 22 ± 2 °C, under light/dark (12:12) cycle. The animals had free access to food and tap water before the experiments. The ambient temperature of the experimental room and humidity was kept consistent throughout all the tests. For behavioral experiments, the animals were selected randomly. Each group consisted of 7–10 animals/dose, and each mouse was used only once. The experiments were performed between 8 a.m. and 2 p.m. Immediately after the in vivo assay the animals were euthanized by cervical dislocation. All procedures for animal maintenance and treatment were approved by the Local Ethics Committee of the Jagiellonian University in Krakow (ZI/862/2013).
Chemicals used in pharmacological tests
PTZ, pilocarpine hydrochloride and scopolamine butylbromide were provided by Sigma Aldrich (Poznań, Poland). For in vivo tests they were prepared in 0.9% saline (Polfa Kutno, Poland). Ethosuximide (ETX, ICN Polfa Rzeszow, Poland) dissolved in distilled water was used as a reference drug in the PTZ model. PTZ and pilocarpine were administered 60 min after the test compound or the vehicle. The test compounds were prepared in 1% Tween 80 (Polskie Odczynniki Chemiczne, Gliwice, Poland). Control mice received 1% Tween 80.
In behavioral assays to establish ED50 value, a dose of 30 mg/kg was chosen as a starting dose for each compound.
The dose of 300 mg/kg was the maximal dose that was tested in vivo.
PTZ seizure test
The test was performed according to Łuszczki et al.Citation19 with some minor modificationCitation20. Clonic convulsions were induced by the subcutaneous (sc) administration of PTZ at a dose of 100 mg/kg. After PTZ injection, the mice were placed separately into transparent Plexiglas cages (30 × 20 × 15) and were observed during the next 30 min for the occurrence of clonic seizures. Clonic seizures were defined as clonus of the whole body lasting more than 3 s, with an accompanying loss of righting reflex. The latency to first clonus, the number of seizure episodes and mortality rate were noted and compared with vehicle-treated and drug-treated groups.
Maximal electroshock seizure test
MES test was performed as previously describedCitation20. Briefly, vehicle-treated mice and drug-treated mice received a stimulus of 25 mA delivered by an electroshock generator (Hugo Sachs rodent shocker, March-Hugstetten, Germany) to induce maximal seizures (tonic extension) of hind limbs. Electroconvulsions were produced with the use of auricular electrodes and the stimulus duration was 0.2 s. The endpoint for this procedure was the tonic extension of the hind limbs.
Pilocarpine-induced seizures
The mice were intraperitoneally pretreated with the investigated compound or vehicle and 60 min later they received pilocarpine (400 mg/kg, ip). To avoid peripheral toxicity and diarrhea, masticatory and stereotyped movements, animals treated with pilocarpine also received scopolamine butylbromide (1 mg/kg, ip) which was injected 45 min before pilocarpine. After the administration of the convulsant, the mice were observed during 60 min for behavioral changes. Latency time to the onset of status epilepticus and latency time to death were noted in each experimental groupCitation21.
Rotarod test
In this test, only the highest, previously tested in the PTZ model, dose of each compound was assessed for its potential ability to impair motor coordination. The test was performed according to the method recently describedCitation22. Briefly the mice were trained daily for three days on the rotarod apparatus (Rotarod apparatus, May Commat RR0711, Ankara, Turkey; rod diameter: 2 cm) rotating at a constant speed of 18 rotations per minute (rpm). During each training session, the animals were placed on a rotating rod for 3 min with an unlimited number of trials. The proper experimentation was conducted 24 h after the final training trial. In order to establish the impact of the test compounds on animals’ motor coordination, 60 min before the rotarod test the mice were ip pretreated with each compound and then they were tested on the rotarod apparatus revolving at 6, 18 and 24 rpm. Motor impairments, defined as the inability to remain on the rotating rod for 1 min, were measured at each speed and the mean time spent on the rod was counted in each experimental group.
Data analysis
Data analysis of the results obtained in behavioral tests was provided by GraphPad Prism Software (ver. 5.0, La Jolla, CA). The results were statistically evaluated using one-way analysis of variance (ANOVA), followed by Dunnett’s post hoc comparison. p < 0.05 was considered significant.
To establish ED50 value for each compound the log-probit method was appliedCitation23. In PTZ test, ED50 was defined as the dose of the investigated compound that prolonged the latency to first clonus by 50%.
Results and discussion
Chemistry
Synthesis of desired thiazole compounds consists of two steps. In the first step, cyclopentanecarbaldehyde thiosemicarbazone 2 was synthesized through reaction of cyclopentanecarbaldehydeCitation1 with thiosemicarbazide in absolute ethanol in the presence of catalytic amount of glacial acetic acid and under reflux with 78% yield. In the next step, Hantzsch cyclization reaction of thiosemicarbazone 2 with appropriate para-substituted bromoacetophenones, ethyl 4-chloroacetoacetate, ethyl 2-chloroacetoacetate, chloroacetone and 1-adamantyl bromomethyl ketone afforded the corresponding thiazoles 3a–3m with good yield (38–87%) and with high chemical purity. The reaction pathway has been summarized in Scheme 1.
All obtained thiazoles were purified on silica gel column chromatography, and in all cases we obtained only pure, most stable (E)-isomersCitation24,Citation25. All compounds were fully characterized spectroscopically using 1H and 13C NMR, FAB(+)-MS, HRMS and elemental analyses. The mass spectra of all compounds are fully consistent with assigned structures. The 1H NMR spectrum of 2 presents typical three proton signals of NH2 and NH groups at 7.41, 7.94 and 10.99 ppm, respectively. These three signals are due to the exchange of H between the terminal NH2 group and sulfur atom.
1H NMR spectra of azole derivatives showed singlet at δ (6.27–7.62) due to thiazole-5H and singlet at δ (11.17–12.05) indicating the presence of hydrazide NH proton, which confirms the conversion of substrates into the expected products. The proton of CH=N group occurs in the spectra around 7.24–7.39 ppm. The elemental analyses values are in good agreement with the calculated values confirming the formation of analytically pure products.
All reactions were repeated at least two times and are fully reproducible.
In vivo pharmacology
PTZ-induced seizures
In this screening test, 13 compounds (3a–3m) were tested for their ability to prolong latency to the onset of the first clonic seizure episode and to reduce the number of seizure episodes. For all of the compounds, except for 3c, 3i and 3j, median effective doses (ED50 values) were established (). Noteworthy, ED50 values obtained for the anticonvulsant active compounds were significantly lower than that reported for ETX (147.8 mg/kg)Citation26.
Table 1. Anticonvulsant potencies of the test compounds 3a–3m and the reference drug (ETX) in the PTZ model expressed as ED50.
The test compounds also influenced the number of seizure episodes induced by PTZ administration. As shown in , compounds 3a (30 mg/kg), 3b (25, 30 mg/kg), 3d (20 mg/kg), 3f (30 mg/kg), 3g (300 mg/kg) and 3h (100 mg/kg) decreased the mean number of seizure episodes in a statistically significant manner (p < 0.05).
Figure 1. Influence of the test compounds 3a–3g (a) and 3h–3m (b) on the number of seizure episodes in PTZ-induced seizure test. Statistical analysis: ANOVA, followed by Dunnett’s post hoc comparison. Significance versus vehicle-treated group: *p < 0.05.
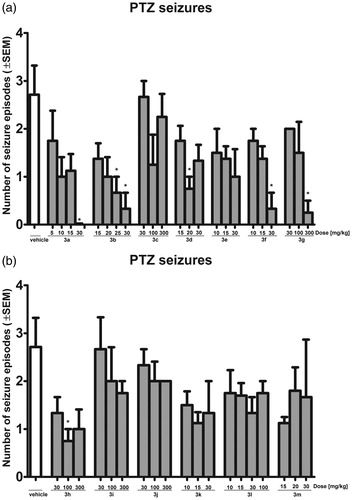
In this assay, ETX (100 mg/kg, ip) reduced the mean number of seizure episodes by 64% (significant versus vehicle-treated mice at p < 0.05).
In the PTZ model, the mortality rate was affected by the test compounds to various degrees. In vehicle-treated mice, the mean mortality rate was 75%. ETX (100 mg/kg), 3a, 3d, 3e, 3j (30 mg/kg), 3b (20, 25 and 30 mg/kg), 3f (15 and 30 mg/kg), 3g (300 mg/kg), and 3h (30, 100 and 300 mg/kg) protected all animals from death. The influence of the test compounds on animals’ mortality is shown in .
MES test
None of the investigated compounds within the tested dose range showed anticonvulsant activity in this model. Tonic hind limb extension was noted in experimental animals, which indicates that the investigated compounds 3a–3m do not protect animals from electrically induced seizures.
Pilocarpine-induced seizures
For extended studies on the anticonvulsant activity in the mouse model of status epilepticus the compound 3a at the dose of 30 mg/kg was chosen. Previously, in the PTZ model this dose not only significantly delayed the seizure onset, but it also decreased the number of seizure episodes and protected all experimental animals from death. In pilocarpine-induced seizures, the compound 3a was not effective. Compared with vehicle-treated mice it did not prolong the latency to status epilepticus (288 s ± 15) for the control group and 292 s ± 14 for 3a-treated mice). Latency to death was not affected either (369 s ± 34 and 368 s ± 11, respectively).
Rotarod test
All compounds at the highest dose that was previously tested in the PTZ model were additionally assessed for their influence on animals’ motor coordination in the rotarod test. The results obtained in this test demonstrated that none of the investigated compounds induced motor deficits in experimental animals (.
Figure 3. Influence of the investigated compounds 3a–3m on motor coordination of experimental animals measured in the rotarod test revolving at 6 rpm (a), 18 rpm (b) and 24 rpm (c). Results are shown as the mean time spent on the rotating rod (±SEM). Statistical analysis: ANOVA, followed by Dunnett’s post hoc test: 6 rpm – F[13,52] = 0.6279, p > 0.05; 18 rpm – F[13,52] = 0.6168, p > 0.05; 24 rpm – F[13,52] = 0.6874, p > 0.05.
![Figure 3. Influence of the investigated compounds 3a–3m on motor coordination of experimental animals measured in the rotarod test revolving at 6 rpm (a), 18 rpm (b) and 24 rpm (c). Results are shown as the mean time spent on the rotating rod (±SEM). Statistical analysis: ANOVA, followed by Dunnett’s post hoc test: 6 rpm – F[13,52] = 0.6279, p > 0.05; 18 rpm – F[13,52] = 0.6168, p > 0.05; 24 rpm – F[13,52] = 0.6874, p > 0.05.](/cms/asset/b12d8ecd-1e87-4881-b29f-519ad740cbff/ienz_a_1158172_f0003_b.jpg)
The search for new AEDs with better pharmacological efficacy and tolerability is still an important goal in the anticonvulsant drug discovery. This process strongly relies on the implementation of preclinical animal models before the anticonvulsant active agents are tested in clinical trials in humansCitation2.
In the present research, three distinct mouse models of seizures were implemented in the in vivo tests. Two of them, i.e. MES test and PTZ test are regarded as simple seizure models that allow to test large numbers of compounds for their potential anticonvulsant activity in relatively short timeCitation2. In view of this, these easy-to-perform assays were used for the anticonvulsant screening of compounds 3a–3m to predict their potential clinical activity.
Noteworthy, these screening assays are not only used to identify novel AEDs, but they also enable to predict the efficacy of AEDs against different types of seizures in humansCitation2. The PTZ model of clonic seizures generally refers to nonconvulsive (absence or myoclonic) seizures in humans, whereas MES test is predictive for generalized tonic–clonic seizuresCitation2. The efficacy of the test compounds in the PTZ model and the lack of anticonvulsant protection demonstrated in MES test suggest that these compounds might be potentially effective in absence seizures in humans but not in grand mal epilepsy.
The observed distinct efficacy of the compounds tested in PTZ test and MES test might also suggest a potential mechanism of their action. Drugs acting by blockade of voltage-gated sodium and to a lesser degree calcium channels (with the exception of ETX) are effective in MES test, and they show no anticonvulsant protection in the PTZ model, whereas numerous GABAergic AEDs and the T-type calcium channel blocker ETX are effective in the PTZ model. Mixed AEDs are active in both tests which is thought to be due to their multi-target profile of the anticonvulsant activityCitation2,Citation3. Bearing that in mind, it can be concluded that the influence of the test compounds on ion channels as a mechanism underlying their anticonvulsant activity is rather implausible. It should be however noted that the elucidation of the precise mechanism of action of compounds 3a–3m requires further extended studies. In view of the chemical similarity of these compounds to well-known MAO inhibitorsCitation9,Citation10, this potential mechanism of the observed in vivo activity should be thoroughly considered.
Similarly to the PTZ model, pilocarpine-induced seizure test is also an animal model using a chemoconvulsant agent to induce seizures. In contrast to PTZ, pilocarpine evokes spontaneous recurrent seizures that mimic status epilepticus in humans and is regarded as a very useful tool to study drug-resistant epilepsyCitation1. In this model, the compound 3a was not effective.
Motor coordination deficits are one of the most frequent adverse effects of AEDs and this effect might be a serious limitation of antiepileptic pharmacotherapy. We demonstrated that the test compounds at a dose range which effectively reduces seizure episodes do not cause motor deficits in experimental animals.
Conclusion
In summary, we have developed an efficient and economic method for the synthesis of 2-(cyclopentylmethylene)-hydrazinyl-1,3-thiazoles. In vivo pharmacological studies of the synthesized compounds have shown that the type of substituent in the para-position of benzene ring is very important for their anticonvulsant activity.
The results of the anticonvulsant screening reveal that seven compounds: 3a, 3b, 3d, 3e, 3f, 3k and 3m containing F-, Cl-, Br-, CF3-, CH3- and adamantyl substituents demonstrated the most significant anticonvulsant activity in the PTZ model, respectively. No effect was observed in electrically induced seizures, and no protection was shown in pilocarpine-induced seizures, either. Noteworthy, none of these compounds impaired animals’ motor skills in the rotarod test. Since the compounds 3a and 3b in a statistically significant manner delayed the onset of clonic seizures and reduced the number of seizure episodes in the PTZ test, they can be regarded as interesting novel lead structures in the search for new AEDs.
Declaration of interest
This study was supported by the Nicolaus Copernicus University (project no. 786/2014) and by the grant from the National Science Centre DEC-2012/05/B/NZ7/02705. The authors confirm that this article content has no conflicts of interest.
References
- Blanco MM, dos Santos JG Jr, Perez-Mendes P, et al. Assessment of seizure susceptibility in pilocarpine epileptic and nonepileptic Wistar rats and of seizure reinduction with pentylenetetrazole and electroshock models. Epilepsia 2009;50:824–31
- Löscher W. Critical review of current animal models of seizures and epilepsy used in the discovery and development of new antiepileptic drugs. Seizure 2011;20:359–68
- Löscher W, Schmidt D. Modern antiepileptic drug development has failed to deliver: ways out of the current dilemma. Epilepsia 2011;52:657–78
- Pasqualotto AC, Thiele KO, Goldani LZ. Novel triazole antifungal drugs: focus on isavuconazole, ravuconazole and albaconazole. Curr Opin Investig Drugs 2010;11:165–74
- Das J, Chen P, Norris D, et al. 2-Aminothiazole as a novel kinase inhibitor template. Structure-activity relationship studies toward the discovery of N-(2-chloro-6-methylphenyl)-2-((6-(4-(2-hydroxyethyl)-1- piperazinyl))-2-methyl-4-pyrimidinyl]amino))-1,3-thiazole-5-carboxamide (Dasatinib, BMS-354825) as a potent pan-Src kinase inhibitor. J Med Chem 2006;49:6819–32
- Zeldin RK, Petruschke RA. Pharmacological and therapeutic properties of ritonavir-boosted protease inhibitor therapy in HIV-infected patients. J Antimicrob Chemother 2004;53:4–9
- Fox LM, Saravolatz LD. Nitazoxanide: a new thiazolide antiparasitic agent. Clin Infect Dis 2005;40:1173–80
- Rehman MZ, Anwar CJ, Ahmad S. An efficient synthesis of 2-alkyl-4-hydroxy-2H-1,2-benzothiazine-3-carboxamide-1,1-dioxides. Bull Korean Chem Soc 2005;26:1771–5
- Chimenti F, Secci D, Bolasco A, et al. Synthesis and selective inhibition of human monoamine oxidases of a large scaffold of (4,5-substituted-thiazol-2-yl)hydrazones. Med Chem Commun 2010;1:61–72
- Chimenti F, Secci D, Bolasco A, et al. Synthesis, semipreparative HPLC separation, biological evaluation, and 3D-QSAR of hydrazothiazole derivatives as human monoamine oxidase B inhibitors. Bioorg Med Chem 2010;18:5063–70
- Siddiqui N, Ahsan W. Triazole incorporated thiazoles as a new class of anticonvulsants: design, synthesis and in vivo screening. Eur J Med Chem 2010;45:1536–43
- Ahangar N, Ayati A, Alipour E, et al. 1-((2-Arylthiazol-4-yl)methyl)azoles as a new class of anticonvulsants: design, synthesis, in vivo screening, and in silico drug-like properties. Chem Biol Drug Des 2011;78:844–52
- Bineshmarvasti M, Sharifzadeh M, Jalilian AR, et al. Synthesis and anticonvulsant activity of N4-substituted triazoly thiazoles. DARU 2003;11:74–8
- Łączkowski KZ, Misiura K, Świtalska M, et al. Synthesis and in vitro antiproliferative activity of thiazole-based nitrogen mustards. The hydrogen bonding interaction between model systems and nucleobases. Anti-Cancer Agents Med Chem 2014;14:1271–81
- Łączkowski KZ, Misiura K, Biernasiuk A, et al. Synthesis, in vitro biological screening and molecular docking studies of novel camphor-based thiazoles. Med Chem 2014;10:600–8
- Łączkowski KZ, Motylewska K, Baranowska-Łączkowska A, et al. Synthesis, antimicrobial evaluation and theoretical prediction of NMR chemical shifts of thiazole and selenazole derivatives with high antifungal activity against Candida spp. J Mol Struct 2016;1108:427–37
- Łączkowski KZ, Misiura K, Biernasiuk A, et al. Synthesis and antimicrobial activities of novel 6-(1,3-thiazol-4-yl)-1,3-benzoxazol-2(3H)-one derivatives. Heterocycl Commun 2014;20:41–6
- Łączkowski KZ, Misiura K, Biernasiuk A, et al. Synthesis, antimicrobial activities and molecular docking studies of novel 6-hydroxybenzofuran-3(2H)-one based 2,4-disubstituted 1,3-thiazoles. Lett Drug Des Discov 2013;10:798–807
- Łuszczki JJ, Czernecki R, Dudra-Jastrzębska M, et al. Influence of agmatine on the protective action of numerous antiepileptic drugs against pentetrazole-induced seizures in mice. Pharmacol Rep 2009;61:252–60
- Sałat K, Podkowa A, Kowalczyk P, et al. Anticonvulsant active inhibitor of GABA transporter subtype 1, tiagabine, with activity in mouse models of anxiety, pain and depression. Pharmacol Rep 2015;67:465–72
- Wilhelm EA, Jesse CR, Roman SS, et al. Anticonvulsant effect of (E)-2-benzylidene-4-phenyl-1,3-diselenole in a pilocarpine model in mice. Life Sci 2010;87:620–7
- Sałat K, Kulig K, Gajda J, et al. Evaluation of anxiolytic-like, anticonvulsant, antidepressant-like and antinociceptive properties of new 2-substituted 4-hydroxybutanamides with affinity for GABA transporters in mice. Pharmacol Biochem Behav 2013;110:145–53
- Litchfield JT, Wilcoxon E. A simplified method of evaluating dose-effect experiments. J Pharmacol Exp Ther 1949;96:99–13
- Łączkowski KZ, Misiura K, Biernasiuk A, Malm A. Discovery and evaluation of efficient selenazoles with high antifungal activity against Candida spp. Med Chem 2015;11:118–27
- Cirilli R, Ferretti R, La Torre F, et al. High-performance liquid chromatographic separation of enantiomers and diastereomers of 2-methylcyclohexanone thiosemicarbazone, and determination of absolute configuration and configurational stability. J Chromatogr A 2007;1172:160–9
- Kamiński K, Zagaja M, Łuszczki JJ, et al. Design, synthesis, and anticonvulsant activity of new hybrid compounds derived from 2-(2,5-dioxopyrrolidin-1-yl)propanamides and 2-(2,5-dioxopyrrolidin-1-yl)butanamides. J Med Chem 2015;58:5274–86