Abstract
Context: Endocrinological factors have been recently described to affect respiratory mechanics.
Objective: To review recent literature data, most of all obtained by the end-inflation occlusion method, describing the effects of molecules of endocrinological interest such as endothelin, erythropoietin and renin-angiotensin, on respiratory mechanics parameters.
Methods: The papers considered in this review were found by inserting in Pubmed/Medline the following indexing terms: hormones, endothelin, erythropoietin, angiotensin and respiratory mechanics.
Results: It was found that the above cited molecules, beside their well known physiological main effects, exhibit influences on respiratory mechanics, most of all on the airflow resistance, which was described to be increased by endothelin and angiotensin, and decreased by erythropoietin.
Conclusions: A number of molecules of biological interest exhibit unexpected influences on respiratory mechanics. The clinical effects depend on the consequences of modified inspiratory pressure values the respiratory muscles have to perform for a given breathing pattern.
Introduction
Recent experimental findings described some interesting effects on respiratory mechanics of a number of hormones. This aspect of endocrinology has been scarcely studied, and the purpose of this report is to review some of the experimental data pertaining to the effects of erythropoietin, endothelin and angiotensin. Recent data have been obtained by the end-inflation occlusion method applied on the rat, which allows to investigate the main aspects of respiratory mechanics; in particular, the effects of the above cited hormones on the respiratory system elastance, the airway resistance to the inspiratory flow and the additional visco-elastic resistance reflecting stress relaxation may be easily measuredCitation1–5.
The effects of these molecules on respiratory mechanics have practical clinical consequences because, depending on the pressure amount which is requested to inflate the respiratory system in different conditions, respiratory muscles have to produce different values of inspiratory pressure during spontaneous breathing. The pressure amount is directly determined by the mechanical characteristics of the system, and will be increased because of increased resistance and/or elastance: high-inspiratory pressure values imply increased mechanical work of breathing, thus leading to the risk of respiratory failure development on a mechanical basis. In fact, the inspiratory muscle capacity to perform mechanical work is obviously limited even in the normal respiratory system, and respiratory muscle failure because of ensuing fatigue may thus develop.
The end-inflation occlusion method
The end-inflation occlusion method has been widely applied to measure respiratory mechanicsCitation1–5.
The respiratory system is inflated by a constant flow ventilator or pump with a definite inflation volume (VT), and the inflation flow (F) is interrupted as rapidly as possible at end inflation. The airway pressure during the inflation and after its interruption is monitored and recorded (), so that the relevant parameters of respiratory mechanics may be determined. The total resistive pressure drop (Pmax,rs), the sudden pressure drop at flow interruption (Pmin,rs), and the visco-elastic pressure component (Pvisc,rs) may be measured on adequately magnified tracings. Pmax,rs is calculated as the difference between the maximum value of pressure at end inflation (Pdyn,max) and Pel,rs, the static elastic equlibrium pressure. Pmin,rs is measured as the difference between Pdyn, max and P1, the pressure value immediately after flow interruption (. Pmin,rs represents the nearly instantaneous, Newtonian resistive pressure drop due to the “ohmic” resistance that theoretically occurs at infinite breathing frequency. Pmin,rs does not include the visco-elastic pressure drop that results from stress-relaxation. The visco-elastic pressure drop describing stress-relaxation is instead calculated as Pvisc,rs = Pmax,rs – Pmin,rs = P1 – Pel,rs. The measurements of these pressure components allows to calculate respiratory system mechanics parameters: the “ohmic” inspiratory resistance to airflow offered by the airways and the resistance due to the movement of respiratory system tissues (Rmin,rs = Pmin,rs/F), and the visco-elastic resistance resulting from the effect of stress-relaxation (Rvisc,rs = Pvisc,rs/F). Respiratory system elastance may also be calculated as Pel,rs/VT.
Figure 1. Example of tracing recorded upon constant flow inflation arrest. The maximum pressure achieved at end inflation (Pdyn,max), the pressure drop due to frictional forces in the airway (Pmin,rs) and the overall resistive pressure drop (Pmax,rs), including Pmin,rs and the nearly exponential pressure dissipation due to viscoelasticity (Pvisc,rs), are shown. P1: pressure value immediately after flow arrest, Pel,rs elastic static pressure.
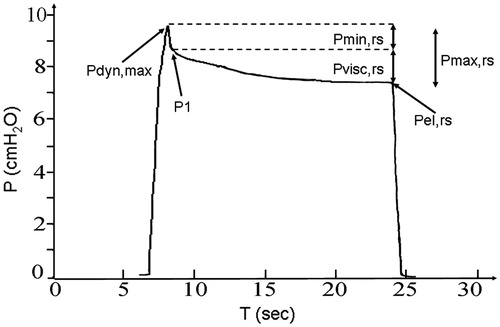
Most biological tissues, including respiratory system tissue, exhibit a typical visco-elastic behavior called stress-relaxation. Due to their visco-elastic properties, the tissues do not maintain constant stress under constant deformation. Rather, the stress slowly relaxes and falls to a lower value.
Most previous studies investigated this aspect using the end-inflation occlusion method: constant-flow respiratory system inflations were imposed, and the slow, nearly exponential, pressure decays following the flow interruption, describing stress-relaxation, were analyzed and quantified. The tissue visco-elastic characteristics can thus be identified accounting for experimental data from end-inflation occlusion method. In detail, this experimental method highlights the typical energy dissipative behavior of visco-elastic systems.
However, the molecular basis and the exact mechanisms of stress-relaxation phenomena are still partially only elucidated. Visco-elasticity combines solid-like and liquid-like characteristics of the respiratory system tissue, and the chief mechanisms responsible for the macroscopic visco-elastic behavior have been proposed to involve the micro-structural rearrangement and interaction processes of collagen and elastin fibers, such as reciprocal sliding, also in relation to interstitial liquid movementsCitation6.
This working hypothesis found a confirmation from observations describing influences on stress-relaxation phenomena of biophysical and biochemical factors which may be expected to affect the lung elastin, collagen and interstitial fluids properties and interactionsCitation7.
It has been well recognized that the stress relaxation-linked pressure drop is a very slow and long lasting process that follows a power law with a small negative power of timeCitation7. Thus, any in vivo recording cannot measure it in its completeness. This limitation holds for the studies cited in the present review, because they focus only on the short time during which stress relaxation can be practically measured by in vivo measurements. However, this represents a small limitation only. In fact, due to the slow exponential time course of stress relaxation, the relatively short temporal windows which were considered () allow to account for the almost entire and anyway quantitatively relevant part of the pressure decay, and accordingly to calculate visco-elastic resistance.
The effect of erythropoietin on respiratory mechanics
Erythropoietin (EPO) is a well known stimulating agent of early erythroid precursor proliferation, but non-hematopoietic effects have also been shown. Just as indicated by the expression of EPO and its receptors in normal healthy lung tissuesCitation8, these effects may affect the physiology of the respiratory system. EPO has, for example, been shown to augment pulmonary ventilation by increasing both the breathing rate and the tidal volume in hemodialized patientsCitation9.
Some investigators have, moreover, reported that EPO exhibits effects on respiratory mechanics parameters such as airway resistance. It has, in fact, been shown to inhibit carbachol-induced broncho-constriction in miceCitation10, and to induce a significant increment in forced vital capacity and in peak expiratory flow rate in humansCitation9, both indicative of EPO-induced decrements in airway resistance.
Similar effects may be the consequence of the protective influences provoked by EPO on inflamed lung tissues by counteracting, for example, the increment in airway resistance caused by inflammatory cytokines such as IL-61.
Data regarding EPO’s influence on vascular smooth muscle tone indicate that it has relaxing effectsCitation11, which may be connected to airway smooth muscle tone. These effects have, in fact, been shown to be associated with increased nitric oxide (NO) productionCitation12, known to reduce airway smooth muscle tone and resistanceCitation13.
However, the sole study directly investigating EPO’s effect on respiratory mechanics parameters and airway resistance in healthy mammals has been only recently publishedCitation14. In this study, respiratory system mechanics were measured in rats using the end-inflation occlusion method before and after EPO administration. The aim of this study was to investigate if spontaneous variations in EPO plasma levels occurring in conditions characterized by blood loss or hypoxia can induce modifications in respiratory system mechanics parameters.
A bronchodilator activity of EPO was demonstrated. In fact, it was found that the intraperitoneal administration of 1000 U/Kg EPO dissolved in PBS significantly reduced Rmin,rs after 20 and 30 min from the injection, an effect which was not seen on control animals receiving PBS only. No significant changes in other respiratory mechanics parameters were observed.
These results may help to clarify the mechanism(s) underlying the decrement in airway resistance observed in rats exposed to chronic hypoxiaCitation15 and/or to hemorrhagic shockCitation16. An increment in EPO production which could contribute, at least in part, to the reduction observed in airway resistance can, in fact, be expected in both conditions.
These findings suggest that EPO mediated physiological responses to hypoxia represent a complex, variable scenario which includes an increment in hematocrit values, an increase in pulmonary ventilationCitation9 further counteracting arterial hypoxia, peripheral vasodilatation increasing tissue oxygen deliveryCitation11,Citation12, and a decrease in respiratory system airway resistanceCitation14 which may help to maintain increased pulmonary ventilation, counteracting deleterious increments in energy dissipation during breathing.
The effects of endothelin on respiratory mechanics
Endothelin is an endothelium-derived peptide which is well known to act on the smooth muscle cells of blood vessels wall inducing a potent vasoconstrictor response.
However, endothelin has also been shown to affect lung mechanics, most of all as a result of airway and parenchimal smooth muscle activation, similarly to the stimulation of the vascular wall smooth muscle. An increment in airway resistance and lung elastance were demonstrated in open-chest guinea-pigsCitation4 as an effect of the stimulation of both ETA and ETB receptorsCitation4. The increased airway resistance was shown to depend on effects on both small and large airways, at least in ratsCitation4. Experiments in open-chest mouseCitation4 and ratsCitation4 confirmed these effects on airway resistance and lung elastance. The contractile properties of endothelin on airway smooth muscle cells were also demonstrated in human isolated bronchial ringsCitation17.
Recent experimental findings obtained in the rat by the end inflation occlusion method showed that the elastance of the whole respiratory system, including chest wall (Est,rs), decreased significantly with respect to control data after 5 and 20 minutes from intraperitoneal endothelin administrationCitation4.
This result may appear in contrast with previously reported results in open-chest animals indicating an increment of lung elastanceCitation4. The reasons for this discrepancy are probably linked to the effects of thoracotomy, which is known to obviously alter respiratory mechanics. More detailed argumentations about this point may be found elsewhereCitation4.
In addition, the same paperCitation4 reported no significant changes for Rvisc,rs, while significant increments of Rmin,rs were observed starting 10 minutes after endothelin administration. This finding of increased Rmin,rs after endothelin is in good agreement with previous results, and confirms that endothelin may stimulate smooth muscle cells contraction not only in the vascular walls, reducing mean airway diameter also. As a consequence, endothelin is expected to increase the resistive work of breathing where the respiratory muscles have to develop for a given breathing pattern.
The effects of renin-angiotensin system on respiratory mechanics
The angiotensin plasma concentration is increased and potentiates bronchoconstriction in asthmatic subjectsCitation18,Citation19. Angiotensin has also been shown to cause bronchoconstriction in guinea pigsCitation20 and ratsCitation21,Citation22, and bronchial hyper-responsiveness to metacholine in human asthmatic subjectsCitation19,Citation23 and guinea pigsCitation20.
Furthermore, the inhibition of angiotensin receptors of type 1, but not of type 2, has been shown to prevent in a dose-related manner the metacholine-induced bronchoconstriction in normalCitation20 and inhalating antigen guinea pigsCitation24. Angiotensin-elicited bronchoconstriction was also shown to be inhibited by type 1 angiotensin receptor antagonismCitation21 and/or angiotensin converting enzyme (ACE) inhibition in ratsCitation22.
Some data suggest that angiotensin may exert its biological effects in normal healthy subjects in basal conditions also. For example, it is well known that low dosages of the ACE inhibitor captopril show their hypotensive effects in normal subjectsCitation25. This suggests that ACE inhibition might influence bronchial smooth muscle tone of normal subjects also. Moreover, angiotensin has been shown to cause contraction of bronchial rings taken from normal ratsCitation21,Citation22, and bronchoconstriction in healthy guinea pigsCitation20.
However, Jamison and GloverCitation26 observed no effect of ACE inhibition on basal airway mechanics on hypocapnic hyperventilation-induced bronchoconstriction in normal subjects. Moreover, ACE inhibition has been demonstrated to leave unaltered baseline lung function in normal subjectsCitation27, and basal airway resistance and bradychinine-induced bronchoconstriction in guinea pigsCitation28. Type 1 angiotensin receptor antagonism did not alter airway resistance in normal guinea pigsCitation24. In addition, greater decreases of pulmonary conductance caused by substance P were observed in captopril-treated guinea pigs than in control animals, suggesting that angiotensin may even exert a bronchodilator effectCitation29.
Thus, the reported effects of angiotensin and/or of ACE or angiotensin receptors inhibition on the airway resistance of normal healthy mammals are conflicting. Data describing the possible effects of ACE inhibition, hence indirectly of basal angiotensin plasma concentration, on respiratory system mechanics parameters, including visco-elastic resistance due to stress relaxation, became recently availableCitation5. They showed that angiotensin inhibition by captopril in rats caused a significant reduction in Rmin,rs, and an almost significant decrement of Rvisc,rs alsoCitation5. Est,rs did not changeCitation5.
These findings of reduced Rmin,rs as an effect of ACE inhibition confirm that angiotensin exerts a bronchoconstrictor effect even in basal conditionsCitation20–22, suggesting it may play a role in the modulation of airway calibre in healthy mammals.
The observed decrement of Rmin,rs is agreement with most previously published results (see above), but in contrast with other experiments. However, the authors of the latter used clinically hypotensive low dosages of ACE inhibitors different from captoprilCitation26,Citation27, or tested much lower captopril dosagesCitation28.
By applying the end-inflation occlusion method, it was also possible to measure the overall inflation inspiratory pressure dissipation including the visco-elastic effects due to stress relaxation. It has been shown that ACE inhibition significantly reduces the overall respiratory system resistance to inflation, hence the overall resistive inspiratory work of breathing.
Thus, these experimental data suggest that ACE inhibition may determinate useful pharmacological effects not only in the vascular bed, but also in the respiratory system.
Conclusion
The intraperitoneal administration of erythropoietin, endothelin and captopril is a well established procedureCitation4,Citation5,Citation14.
In the effects on Rmin,rs are summarized that were observed using the end-inflation occlusion method in the ratsCitation4,Citation5,Citation14.
Table 1. Mean values (±SE) of Rmin,rs (cm H2O/ml sec−1) in rats before (control) and after (time in minutes) intraperitoneal injections of erythropoietin, endothelin and captopril.
It appears that molecules of endocrinological interest may affect respiratory mechanics parameters. These findings indicate a complex biological scenario, in which endocrinological factors show unexpected biological influences in addition to their well known physiological effects.
Beside the interest for basic and physiological research, the relevant aspects from a clinical point of view include the followings: (a) in many pathological conditions, modifications of the plasmatic concentration of molecules of biological interest such as different hormones are expected; hence, it becomes necessary to consider possible influences on respiratory mechanics. (b) These influences may have important consequences. As an example, because of impaired mechanical properties of the respiratory system, i.e. increased values of resistance and/or elastance, the inflation pressure the inspiratory muscles have to develop for a given pattern of breathing will increase; the performance of the inspiratory muscles is obviously limited in time and strength, so that muscle fatigue and ventilatory failure may ensue. (c) The above described influences on respiratory mechanics may be object of therapeutic and useful pharmacological manipulation.
Declaration of interest
The authors report no conflicts of interest. The authors alone are responsible for the content and writing of this article.
References
- Rubini A. IL-6 increases airway resistance in the rat. Cytokine 2010;51:266–73
- Rubini A, Porzionato A, Zara S, et al. The effect of acute exposure to hyperbaric oxygen on respiratory system mechanics in the rat. Lung 2013;191:459–66
- Rubini A, El-Mazloum D, Morra F, Bosco G. The effect of body cooling on respiratory system mechanics and hysteresis in rats. Respir Physiol Neurobiol 2013;189:52–8
- Rubini A, Morra F, El-Mazloum D. The effects of endothelin on respiratory system hysteresis and mechanics measured by the end-inflation occlusion method in the rat. Minerva Pneumol 2014;53:35–42
- Rubini A, Redaelli M, Parmagnani A. The effect of angiotensin converting enzyme inhibition by captopril on respiratory mechanics in healthy rats. J Enzyme Inhib Med Chem 2012;27:854–60
- Suki B, Barabasi AL, Lutchen KR. Lung tissue viscoelasticity: a mathematical framework and its molecular basis. J Appl Physiol 1994;76:2749–59
- Rubini A, Carniel EL. A review of recent findings about stress relaxation in the respiratory system tissues. Lung 2014;192:833–9
- Yasuda Y, Hara S, Hirohata T, et al. Erythropoietin-responsive sites in normal and malignant human lung tissues. Anat Sci Int 2010;85:204–13
- Ucok K, Gokbel H, Yeksan M, et al. The effects of rHuEPO administration on pulmonary functions in haemodialysis patients. Int J Artif Organs 1996;19:336–8
- Tyagi MG, Goyal S, Sathyakumar K, Subbana PK. Influence of erythrocyte function-enhancing drugs on the bronchoprotective actions of chemokine receptor blockers in mice. Med Sci Monit 2006;12:BR279–82
- Shafi NI, Andresen J, Marrelli SP, Bryan RM. Jr. Erythropoietin potentiates EDHF-mediated dilations in rat middle cerebral arteries. J Neurotrauma 2008;25:257–65
- Santhanam AV, D'Uscio LV, Katusic ZS. Cardiovascular effects of erythropoietin an update. Adv Pharmacol 2010;60:257–85
- Rubini A. The Effect of N(G)-nitro-L-arginine methyl ester, a nitric oxide synthase inhibitor, on respiratory mechanics in rats. Respiration 2011;82:468–75
- Rubini A, Del Monte D, Catena V. Erythropoietin acutely decreases airway resistance in the rat. Regul Pept 2012;178:76–9
- Habre W, Jànosi TZ, Fontao F, et al. Mechanisms for lung function impairment and airway hyperresponsiveness following chronic hypoxia in rats. Am J Physiol Lung Cell Mol Physiol 2010;298:L607–14
- Bayat S, Albu G, Layachi S, et al. Acute hemorrhagic shock decreases airway resistance in anesthetized rats. J Appl Physiol 2011;111:458–64
- Clayton RA, Naily JE, Thomson NC, McGrath JC. Interactions between endothelin-I-induced contractions and bronchodilators in human isolated bronchi. Clin Sci (London) 1997;93:527–33
- Millar EA, Angus RM, Hulks G, et al. Activity of the renin-angiotensin system in acute severe asthma and the effect of angiotensin II on lung function. Thorax 1994;49:492–5
- Millar EA, Nally JE, Thomson NC. Angiotensin II potentiates methacholine-induced bronchoconstriction in human airway both in vitro and in vivo. Eur Respir J 1995;8:1838–41
- Watanabe K, Myou S, Fujimura M, et al. Importance of the angiotensin type 1 receptor in angiotensin II-induced bronchoconstriction and bronchial hyperresponsiveness in the guinea pig. Exp Lung Res 2004;30:207–21
- Veerappan A, Reid AC, Estephan R, et al. Mast cell renin and a local renin-angiotensin system in the airway: role in bronchoconstriction. Proc Natl Acad Sci USA 2008;105:1315–20
- Krop M, Ozunal ZG, Chai W, et al. Mast cell degranulation mediates bronchoconstriction via serotonin and not via renin release. Eur J Pharmacol 2010;640:185–9
- Chalmers GW, Millar EA, Little SA, et al. Effect of infused angiotensin II on the bronchoconstrictor activity of inhaled endothelin-1 in asthma. Chest 1999;115:352–6
- Myou S, Fujimura M, Kurashima K, et al. Type 1 angiotensin II receptor antagonism reduces antigen-induced airway reactions. Am J Respir Crit Care Med 2000;162:45–9
- Gainer JV, Morrow JD, Loveland A, et al. Effect of bradykinin-receptor blockade on the response to angiotensin-converting-enzyme inhibitor in normotensive and hypertensive subjects. N Engl J Med 1998;339:1285–92
- Jamison JP, Glover PJ. Effect of angiotensin converting enzyme inhibition on airway conductance during hypocapnic hyperventilation in normal subjects. Pulm Pharmacol 1995;8:49–51
- Bauer KG, Brunel P, Nell G, et al. Benazepril, an angiotensin converting enzyme inhibitor: drug interaction with salbutamol and bronchial response to histamine in normal subjects. Br J Clin Pharmacol 1997;44:573–5
- Greenberg R, Osman GH Jr, O'Keefe EH, Antonaccio MJ. The effects of captopril (SQ 14,225) on bradykinin-induced bronchoconstriction in the anesthetized guinea pig. Eur J Pharmacol 1979;57:287–94
- Shore SA, Stimler-Gerad MP, Coats SR, Drazen JM. Substance P-induced bronchoconstriction in the guyinea pig. Enhancement by inhibitors of neutral metalloendopeptidase and angiotensin-converting enzyme. Am Rev Resp Dis 1988;137:331–6