Abstract
Mercury exposure in both humans and mice is associated with features of systemic autoimmunity. Murine HgCl2-induced autoimmunity (mHgIA) requires MHC Class II, CD4+ T-cells, co-stimulatory molecules, and interferon-γ (IFN-γ), similar to spontaneous models of systemic lupus erythematosus (SLE). β2-microglobulin (B2m) is required for functional MHC Class I molecules and the neonatal Fc receptor (FcRn). Deficiency of B2m in lupus-prone strains is consistently associated with reduced IgG levels, but with variable effects on other manifestations. Herein, we examined the role of B2m in mHgIA and show that in the absence of B2m, mercury-exposed mice failed to exhibit hypergammaglobulinemia, had reduced anti-nucleolar autoantibodies (ANoA), and had a lower incidence of immune complex deposits in splenic blood vessels, whereas IgG anti-chromatin autoantibodies and renal immune deposits were largely unaffected. Subclass analysis of the IgG anti-chromatin, however, revealed a significant reduction in the IgG1 subtype. Examination of IFNγ, IL-4, and IL-2 in exposed skin, draining lymph nodes, and spleen following mercury exposure showed reduced IL-4 in the spleen and skin in B2m-deficient mice, consistent with the lower IgG1 anti-chromatin levels, and reduced IFNγ expression in the skin. These findings demonstrate how a single genetic alteration can partially but significantly modify the clinical manifestations of systemic autoimmunity induced by exposure to xenobiotics.
Introduction
Exposure to mercury has been identified as an environmental trigger in the induction of autoimmunity in humans (Abréu Vélez et al., Citation2003; Cooper et al., Citation2004; Silva et al., Citation2004) including production of autoantibodies, pro-inflammatory cytokines (Gardner et al., Citation2010), and membranous nephropathy (Li et al., Citation2010). Animal model studies have contributed significantly to our understanding of the systemic autoimmunity induced by this environmental agent (Vas and Monestier, Citation2008; Schiraldi and Monestier, Citation2009; Pollard et al., Citation2010). The features of murine mercury-induced autoimmunity (mHgIA) include lymphadenopathy, hypergammaglobulinemia, humoral autoimmunity, and immune complex disease (Pollard and Hultman, Citation1997; Vas and Monestier, Citation2008; Schiraldi and Monestier, Citation2009; Pollard et al., Citation2010). HgIA is dependent on functional major histocompatibility (MHC) Class II, CD4+ T-helper cells (Hultman et al., Citation1995) and co-stimulatory molecules (Pollard et al., Citation2004). MHgIA is also dependent upon interferon (IFN)-γ (Kono et al., Citation1998) that is predominantly secreted by CD4+ and CD8+ T-cells and natural killer (NK) cells (Boehm et al., Citation1997). NK cell function is reduced following mercury exposure (Santarelli et al., Citation2006), but NKT cell activation exacerbates mHgIA (Vas et al., Citation2008) and CD8+ T-cells proliferate in response to HgCl2 (Jiang and Moller, Citation1995; Pollard and Landberg, Citation2003). However, it is unclear if absence of MHC Class I-restricted cells contributes to development of disease.
β2-microglobulin (B2m) is critical for cell surface expression of MHC Class I molecules (Zijlstra et al., Citation1989) and deficiency leads to a loss of several cell types that require Class I for development, including CD8+ T-cells and NK1.1+ T-cells (Zijlstra et al., Citation1990; Bendelac et al., Citation1994). B2m is also critical for neonatal Fc receptor (FcRn) function (Israel et al., Citation1995). FcRn facilitates the transfer of maternal IgG from mother to fetus in humans and from gut to blood in suckling rodents, and, in adults, protects IgG from degradation (Ahouse et al., Citation1993; Israel et al., Citation1995; Roopenian and Akilesh, Citation2007). Absence of FcRn in B2m-deficient mice results in increased catabolism of IgG (Israel et al., Citation1996) and consequently reduced half-life and serum levels of IgG (Ghetie et al., Citation1996; Christianson et al., Citation1997). It has been argued that FcRn inhibitors could be used to reduce autoantibody levels in autoimmune disease (Low and Mezo, Citation2009).
B2m deficiency prevents hypergammaglobulinemia in SLE (systemic lupus erythematosus)-prone mice, and reduces specific antibody responses to T-dependent and T-inde- pendent antigens (Christianson et al., Citation1997). Hypogammaglobulinemia was argued to be the result of the reduced half-life of IgG in B2m-deficient mice, rather than reduction in B-cells, CD8+ T-cells, or NK1.1+ cells (Christianson et al., Citation1997). A B2m deficiency, however, has produced variable effects on murine systemic autoimmunity. For example, NZB.B2m−/− mice showed no change in levels of anti-DNA autoantibodies, but the incidence of anti-erythrocyte autoantibodies was reduced and their onset delayed (Chen et al., Citation1997). In one study in MRL/Faslpr mice, this deficiency reduced all measured indicators of systemic autoimmunity (including hypergammaglobulinemia, autoantibodies and glomerulonephritis; Christianson et al., Citation1996), while another study showed no effect of B2m deficiency on autoantibody production (Ohteki et al., Citation1995). A lack of B2m in C57BL/6 mice carrying the lpr mutation decreased (Maldonado et al., Citation1995), increased (Giese and Davidson, Citation1995), or did not change (Mixter et al., Citation1995) autoantibody levels. The only consistent effect of a B2m deficiency in mice carrying the lpr mutation was a profound reduction in B220+CD4-CD8- T-cells (B220+ double negative [DN] T-cells), consistent with the derivation of most DN T-cells from the CD8+ T-cell subset (Koh et al., Citation1995). MHC Class I expression is important for their accumulation. In contrast, B2m deficiency exacerbated autoimmunity in NZBWF1 mice resulting in increased anti-DNA autoantibodies, more severe proteinuria, and decreased survival (Mozes et al., Citation2005). Thus, B2m appears to play different roles in lupus-like disease depending on background genetic susceptibility.
To define the role of B2m in xenobiotic induction of systemic autoimmune disease we have examined its contribution to mHgIA. In the absence of B2m, mercury exposure did not elicit the characteristic hypergammaglobulinemia and certain disease manifestations, including anti-nucleolar autoantibodies (ANoA) and vascular-immune deposits in the spleen, but not kidney immune deposits, were reduced. The IgG subclass response to chromatin and cytokine expression in skin and lymphoid tissues were also altered by B2m deficiency. Thus, B2m plays a significant disease-promoting role for some, but not all, of the autoimmune manifestations induced by mercury exposure.
Materials and methods
Mice
C57BL/6 mice with targeted disruption of the B2m gene (B6.129P2-B2mtm1Unc) were obtained from The Jackson Laboratory (Bar Harbor, ME). Subsequent breeding and maintenance were performed under specific-pathogen-free conditions at The Scripps Research Institute Animal Facility (La Jolla, CA). All studies involving animals were reviewed and approved by The Scripps Research Institute Animal Research Committee. Animal rooms were kept at 68–72°F and 60–70% humidity, with a 12/12 h light-dark cycle, and sterilized cages were replaced each week with fresh water and food to which the mice had access ad libitum.
H-2s haplotype B2m−/− mice were generated by first back-crossing homozygous knockout mice (H-2b haplotype) for two generations to B10.SeCD1 (H-2s) mice. The (B2m−/− × B10.S) F1 × B10.S N2 offspring were then typed for H-2s and the disrupted B2m gene. Mice, homozygous for H-2s and heterozygous for the B2m gene, were intercrossed to create +/+, +/−, and −/− B2m H-2s mice. H-2 typing was performed by PCR of genomic DNA using the D17Mit16 primers (Research Genetics, Huntsville, AL) that are poly-morphic for H-2b and H-2s haplotypes. B2m wild-type and knockout genes were typed by PCR of genomic DNA (35 cycles of 94°C/20 s, 60°C/45 s, 72°C/75 s). Three primers (Mβ2m-1, 3′-GAAAACCCCTCAAATTCAAGTATACTCA-5′; Mβ2m-2, 5′-GACGG-TCTTGGGCTCGGCCATACT-3′′; neomycin, 5′-TCGAATTCGCCAATGACAAGACGCT-3′) were used as previously described (Khare et al., Citation1996). Mβ2m-1 and Mβ2m-2 were used to detect the undisrupted gene (400 basepair fragment) and neomycin and β2m-1 the disrupted gene (280 basepair fragment).
Mercury treatment
Unless noted otherwise, mice were injected subcutaneously with 40 µg mercuric chloride (HgCl2; Mallinckrodt Baker Inc, Phillipsburg, NJ) twice per week for 2 or 4 weeks in phosphate-buffered saline (pH 7.4, PBS) as previously described (Pollard et al., Citation2004) and euthanized (by Halothane (Halocarbon Labs, River Edge, NJ) anesthesia prior to cervical dislocation) 3 days after the final injection in the 2 or 4 weeks regimens. Controls received PBS alone. Use of HgCl2 was approved by The Scripps Research Institute Department of Environmental Health Services.
Autoantibody determination
Anti-nuclear (ANA) and anti-nucleolar antibodies (ANoA)
ANA and ANoA were detected as previously described (Pollard et al., Citation2004) using HEp-2 cell slides (Bion Enterprises, Park Ridge, IL). Briefly, sera were diluted 100-fold in PBS containing 0.5% bovine serum albumin (BSA), 0.1% bovine gammaglobulin (BGG), 0.001% gelatin, and 0.05% Tween-20 prior to assay. Goat anti-mouse IgG-FITC (Caltag Laboratories, South San Francisco, CA), diluted 100-fold in PBS containing 0.5% BGG, 0.1% BSA, and 0.05% Tween-20, was used as a detecting reagent. Intensity of fluorescence was scored from 0 − 4+. ANoA in mHgIA are MHC-restricted and directed against components of the small nucleolar ribonucleoprotein particles (snoRNP) (Yang et al., Citation2001). Comparative titration of IgG anti-snoRNP antibodies was achieved by quadruple dilutions of serum starting at 1/40.
Anti-chromatin antibodies
Anti-chromatin antibodies were detected using ELISA (Burlingame and Rubin, Citation1990). Briefly, sera were diluted 100-fold prior to assay, and chromatin bound antibodies were detected with horseradish peroxidase (HRP)-conjugated goat anti-mouse IgG (Caltag Laboratories) diluted 2000-fold. Anti-chromatin monoclonal antibody 1D12 (Kotzin et al., Citation1984) was used as a positive control. Comparative titration of IgG anti-chromatin antibodies was achieved by doubling dilutions of serum starting at 1/100. The IgG subclass of anti-chromatin antibodies was determined using serum dilutions of 1/200 and a saturating (1/1000) dilution of subclass detecting reagent. HRP-Conjugated anti-IgG1 and IgG2b antibodies were purchased from Caltag Laboratories, anti-IgG2aa+b from PharMingen (San Diego, CA), and anti-IgG3 from Southern Biotechnology Associates (Birmingham, AL).
Serum immunoglobulin quantitation
Serum IgG1 and IgG2a levels were quantified using ELISA (Pollard et al., Citation1999). Briefly, ELISA plates were coated with 200 µL of 2 µg/mL goat anti-mouse kappa light chain antibody (Caltag Laboratories) diluted in PBS and incubated overnight at 4°C. The plates were then post-coated for 1 h with 0.1% gelatin in PBS followed by three washes with PBS-0.05% Tween-20. Sera were diluted in serum diluent (Bell et al., Citation1992) and then added (in duplicate) to dedicated wells on the plates; the plate was then incubated with shaking for 2.5 h, and then washed three times with PBS-0.05% Tween-20. HRP-Conjugated goat anti-mouse IgG1 or IgG2a antibodies (Caltag Laboratories) were then diluted in anti-Ig diluent and added to the wells; the plates were then incubated with shaking for 90 min. Following three washes with PBS-0.05% Tween-20 and four washes with PBS, ABTS substrate solution was added to each well and the associated optical density (OD) in each well measured at 405 nm in a Spectra Max Plus 384 plate reader (Molecular Devices, Sunnyvale, CA). Serum IgG1 and IgG2a concentrations were ultimately calculated by extrapolation from the linear portion of the standard curves generated with each plate using serial dilutions of polyclonal mouse reference sera containing predetermined levels of Ig isotypes (The Binding Site, Birmingham, UK).
Immunopathology
Sections of kidney and spleen were prepared and stained for direct immunofluorescence as previously described (Kono et al., Citation1998). Briefly, 4–5-µm thick cryostat sections were fixed in ethanol and incubated with serial dilutions of FITC-conjugated goat antibodies to IgG (γ chain-specific) and C3 (Southern Biotechnology Associates). The endpoint titer for immune deposits was defined as the highest dilution of antibody at which specific fluorescence could be detected. Vessel wall deposits were graded on a 0–4+ scale. The slides were examined without knowledge of treatment or other results.
In vivo cytokine responses
To measure cytokine production following exposure to B2m+/+ and B2m−/− mice were injected subcutaneously (SC) twice per week for 2 weeks with 40 µg HgCl2 in PBS or PBS alone. Post-mortem, the spleen, superficial cervical lymph nodes (draining lymph nodes), and a 1.2 cm diameter section of skin overlying the site of mercury injection was harvested from each mouse. Total RNA from these tissues was then isolated and purified using TRIzol reagent (Invitrogen Life Technologies, Carlsbad, CA). Contaminant DNA was removed using a DNase treatment (Amplification Grade DNase I, Invitrogen) at 25°C for 15 min. Final RNA content in each sample was then determined with a ND-100 Spectrophotometer (NanoDrop Technologies, Wilmington, DE). An aliquot of the RNA (2 µg) was then denatured at 65°C for 5 min, placed on ice, and reverse-transcribed (in a total volume of 21 µL) using random hexamers, dNTPs, RNase inhibitor (RNaseOUT; Invitrogen), and 200 U of SuperScript III reverse transcriptase (Invitrogen). The resulting cDNA was diluted 10-fold in nuclease-free water (Nuclease-Free Water, Ambion Inc. Austin, TX), and stored at −80°C.
Levels of interferon (IFN)-γ, interleukin (IL)-4, and IL-2 in samples were measured by real-time PCR using primer and probe sequences as previously described (Giulietti et al., Citation2001). Spleen and lymph node cDNA were analyzed as previously described and levels expressed rela-tive to cyclophilin A (Cauvi et al., Citation2007). Levels of cytokines in cDNA prepared from skin were analyzed using iQ SYBR-Green (Bio-Rad, Hercules, CA) and copy number related to total RNA concentration (Bustin, Citation2000). PCR amplifications were performed in a total volume of 20 µL containing 20 mM Tris-HCl, 50 mM KCl, 200 µM dNTPs, 0.50 U iTaq DNA polymerase, 3 mM MgCl2, and 10 nM SYBR-Green I fluorescein. The reaction conditions were 94°C for 5 min, followed by 55 cycles of 20 sec at 94°C and 30 sec at 60°C; the melt curve used 70 cycles of 10 sec at 60 + 0.5°C. All PCR reactions were performed using an iCycler iQ (Bio-Rad).
Flow cytometry
Flow cytometry was performed on single cell suspensions obtained from harvested spleens as previously described (Cauvi et al., Citation2007) using complete RPMI 1640 media (Whittaker M.A. Bioproducts, Walkersville, MD) containing 10% FCS (Hyclone Laboratories, Logan, UT), 2 mM L-glutamine (Sigma Chemical Co., St. Louis, MO), 10 mM HEPES (pH 7.2; Flow Laboratories, Irvine, Scotland), 1% non-essential amino acids (Irvine Scientific, Santa Ana, CA), 20 mM sodium carbonate (Fisher Scientific Company, Fair Lawn, NJ), and 20 µg/mL gentamicin sulfate (Irvine Scientific). After washing in complete RPMI 1640 and then PBS containing 0.04% horse serum (Gemini Bio-Products, Woodland, CA), cells were incubated in PBS/0.04% horse serum with Fc Block and then stained with combinations of anti-NK1.1-FITC, Thy1-PE, anti-B220-PerCP, anti-CD3-APC, anti-CD4-FITC, or anti-CD8α-PerCP for 30 min at 4°C, or appropriate isotype controls. All antibodies were from BD PharMingen (San Diego, CA). Cells were analyzed on a dual-laser FACScalibur flow cytometer (BD Biosciences, San Jose, CA). A minimum of 20,000 events was acquired for each sample; all data were analyzed using CellQuest Pro software (BD Biosciences).
Statistics
Unless otherwise noted, all data is expressed as mean ± SD. Groups were compared by two-tailed single-factor analysis of variance (ANOVA), unpaired t-test, or Mann-Whitney U test as appropriate. A p-value less than 0.05 was considered significant.
Results
Characteristics of B2m−/− mice
Mice used in this study were produced by mating H-2s/s (B10.SeCD1) mice heterozygous for the B2m gene. B2m+/+, B2m+/−, and B2m−/− littermates were genotyped by PCR and all offspring appeared healthy. Flow cytometric analyses of T-cells from various lymphoid organs revealed the expected absence of CD8+ T-cells in both spleen and lymph nodes of B2m−/− mice (data not shown). A B2m deficiency also resulted in a 1.8-fold reduction in serum IgG1 (p = 0.0043) and 7.5-fold reduction of IgG2a (p < 0.0001) compared to values associated with wild-type littermates ().
Table 1. Immunoglobulin levels and autoantibodies in B2m+/+, B2m+/−, and B2m−/− mice following 4 weeks of mercury exposurea.
Features of xenobiotic-induced systemic autoimmunity in B2m-deficient mice
Exposures to HgCl2 elicited hypergammaglobulinemia, ANoA and anti-chromatin autoantibodies, and immune complex deposits in wild-type mice (B2m+/+) as described previously (Kono et al., Citation1998; Pollard et al., Citation2004) and in B2m+/− mice ( and ). In contrast, the HgCl2 did not elicit a significant increase in hypergammaglobulinemia in B2m−/− mice and their levels of IgG1 (p < 0.0001) and IgG2a (p < 0.0001) were markedly lower than that of mercury-exposed wild-type mice or B2m+/− (). Strikingly, after mercury exposure, IgG1 serum concentrations were 6-fold lower in B2m−/− than in wild-type mice, and 10-fold lower for IgG2a. In contrast, B2m−/− mice had increased autoantibody responses to both ANoA and chromatin (). Indeed, all three groups of HgCl2-exposed mice showed significant and similar increases in IgG anti-chromatin autoantibodies (). However, although all mice developed IgG ANoA following mercury exposure (), the geometric mean of IgG ANoA titers was over 4-fold greater for B2m+/+ compared to B2m−/− mice (1540 vs. 340; p < 0.025) (). This reduction of ANoA in mercury-exposed B2m−/− mice was supported by comparison of the intensities of fluorescence for ANoA at a dilution of 1/100, which for B2m−/− and B2m+/+ mice was 1.3 (± 1.1) vs. 2.8 (± 0.6), respectively (p = 0.003, ).
Table 2. Immunopathological changes in B2m+/+, B2m+/−, and B2m−/− mice following 4 weeks of mercury exposurea.
Figure 1. ANA and ANoA in HgCl2-exposed B2m+/+ and B2m−/− mice. Mice were injected with HgCl2 (40 µg in 100 µL PBS) twice per week for 4 weeks before determination of serum IgG ANoA by indirect immunofluorescence on HEp-2 cells. (A) Endpoint titer of IgG ANoA in B2m+/+ and B2m−/− mice. The geometric mean (and range) of IgG ANoA titers differed significantly between B2m−/− and B2m+/+ mice (340 [160–640] vs. 1540 [160–2560]; p < 0.025). (B) Fluorescence intensity of IgG ANA and ANoA in B2m+/+ (n = 9), B2m+/− (n = 8) and B2m−/− (n = 8) mice at a serum dilution of 1/100. ANoA autoantibodies (as mean ± SEM) differed significantly between B2m−/− (n = 8) and B2m+/+ (n = 9) mice (1.3 (± 1.1) vs. 2.8 (± 0.56); p = 0.0027).
![Figure 1. ANA and ANoA in HgCl2-exposed B2m+/+ and B2m−/− mice. Mice were injected with HgCl2 (40 µg in 100 µL PBS) twice per week for 4 weeks before determination of serum IgG ANoA by indirect immunofluorescence on HEp-2 cells. (A) Endpoint titer of IgG ANoA in B2m+/+ and B2m−/− mice. The geometric mean (and range) of IgG ANoA titers differed significantly between B2m−/− and B2m+/+ mice (340 [160–640] vs. 1540 [160–2560]; p < 0.025). (B) Fluorescence intensity of IgG ANA and ANoA in B2m+/+ (n = 9), B2m+/− (n = 8) and B2m−/− (n = 8) mice at a serum dilution of 1/100. ANoA autoantibodies (as mean ± SEM) differed significantly between B2m−/− (n = 8) and B2m+/+ (n = 9) mice (1.3 (± 1.1) vs. 2.8 (± 0.56); p = 0.0027).](/cms/asset/78a05263-2cf0-47f9-888d-922e9162f6e0/iimt_a_583614_f0001_b.gif)
On pathologic examination, exposure to mercury resulted in significant and similar titers of IgG and C3 deposits in the glomeruli of B2m+/+, B2m+/−, and B2m−/− mice (). Similarly, IgG and C3 deposits in kidney vessels were found in all three groups, but with only one or two animals positive in any group. In contrast, B2m+/− and B2m−/− mice had reduced deposits of IgG and C3 in splenic vessels when compared to wild-type mice (). Thus, B2m deficiency affects some features of systemic autoimmunity induced by mercury exposure.
Titers and subclasses of anti-chromatin autoantibody in B2m-deficient mice
The presence of elevated autoantibody levels despite the lack of hypergammaglobulinemia in mercury-exposed B2m−/− mice was an unexpected finding (). To confirm this, the anti-chromatin autoantibody response was further analyzed by titration () and subclass content (). IgG anti-chromatin titration curves for B2m−/− and B2m+/+ mice were essentially identical (), suggesting that either chromatin specific IgG autoantibody levels and/or their affinity did not differ between the two groups. Measurement of IgG subclass specific anti-chromatin autoantibodies showed that although B2m−/− mice had a significantly lower IgG1 anti-chromatin autoantibody response compared to wild-type littermate controls (p = 0.0006), the levels of IgG2a, IgG2b, and IgG3 anti-chromatin autoantibodies were not significantly different between the two groups (). Thus, B2m is required for the IgG1, but not overall anti-chromatin antibody production induced by mercury.
Table 3. IgG subclass of anti-chromatin antibodies in B2m+/+and B2m−/− mice following 4 weeks of mercury exposure.
Figure 2. Titration of IgG anti-chromatin antibodies in HgCl2-exposed B2m+/+ and B2m−/− mice. Mice were injected with HgCl2 (40 µg in 100 µL PBS) twice per week for 4 weeks before determination of serum IgG anti-chromatin antibodies using ELISA. Titration consisted of serial 2-fold dilution of individual sera from 1/100 to 1/3200. OD405 values represent the geometric mean at each serum dilution for B2m+/+ (n = 9) and B2m−/− (n = 6) mice. B2m+/+ ▪ B2m−/− ▪.
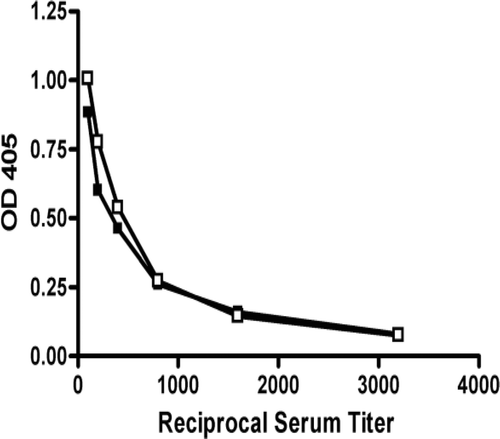
In vivo production of cytokines in B2m-deficient mice
Reduction of IgG1, but persistence of IgG2a, anti-chromatin autoantibodies in B2m−/− mice suggested that differential expression of Th1/Th2 cytokines might contribute to the humoral autoimmune response. To examine this possibility, cytokine expression was determined after 2 weeks of mercury exposure (when humoral responses are evident) by real-time PCR in the spleen, draining lymph nodes, and skin overlying the site of mercury injection ().
Figure 3. IFNγ, IL-2, and IL-4 expression in the (A) spleen, (B) draining lymph node, and (C) skin of HgCl2-exposed B2m+/+ and B2m−/− mice. Mice were injected subcutaneously with 40 µg HgCl2 in phosphate-buffered saline (PBS) (solid bars) or with PBS alone (open bars) for 2 weeks. Total RNA was prepared and quantitative real-time polymerase chain reaction was performed on reverse-transcribed RNA. The mRNA level was normalized using cyclophilin A for spleen and lymph node. For skin samples, cytokine mRNA levels were related to total RNA concentration. Values represent the mean and SEM of 4 mice per group.
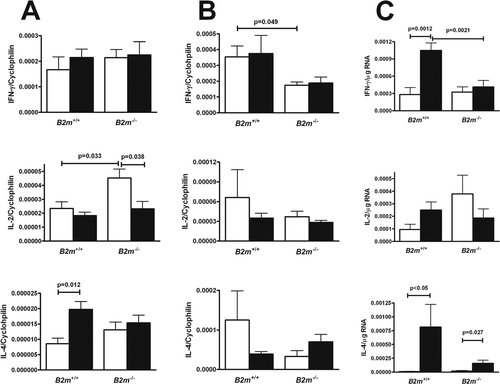
In the spleen, mercury exposure resulted in increased IL-4 (p = 0.012) in wild-type mice as described previously (Kono et al., Citation1998), but no changes in IL-2 or IFNγ (). In contrast, splenic IL-2 production was elevated in saline-treated B2m−/− mice compared to that in both wild-type mice (p = 0.033) and mercury-exposed B2m−/− (p < 0.038); however, there was no difference in IL-2 expression between the mercury-exposed wild-type and B2m−/− mice. In B2m−/− mice, levels of splenic IFNγ or IL-4 were not changed by mercury exposure.
In lymph nodes, B2m deficiency resulted in a significant reduction in IFNγ regardless of whether mice were exposed to mercury or PBS (p = 0.049). The levels of IL-2 and IL-4 were not significantly different in B2m−/− and wild-type mice, with or without mercury exposure ().
In the skin, there was a significant increase in IFNγ in wild-type mice following mercury exposure (p = 0.0012); in striking contrast, levels in B2m−/− mice did not increase and were significantly reduced compared to those in wild-type hosts (p = 0.0021) (). IL-4 levels were also significantly elevated in wild-type mice after mercury exposure (p < 0.05) and, to a lesser degree, increased in B2m−/− mice (p = 0.027). IL-2 expression was not significantly affected by mercury exposure in wild-type or B2m−/− mice. These findings indicate that B2m deficiency affects cytokine levels primarily in mercury-exposed skin where it is associated with significant reductions in both IL-4 and IFNγ.
Discussion
In this study, we investigated the role of B2m in xenobiotic-induced autoimmunity by comparing murine mercury-induced autoimmunity (mHgIA) in B2m−/− and wild-type mice. A B2m deficiency markedly reduced mercury-induced hypergammaglobulinemia, including that for IgG1 and IgG2a isotypes, lowered levels of ANoA (but not anti-chromatin), preferentially reduced levels of IgG1 isotype anti-chromatin, and reduced IL-4 and IFNγ cytokine expression at the site of mercury exposure; in contrast, this status did not affect the incidence and severity of glomerular immune complex deposits. Thus, B2m is required for some of the features of mHgIA.
Previous studies have shown that both B2m (Spriggs et al., Citation1992) and neonatal Fc receptor (FcRn) (Roopenian et al., Citation2003)-deficient mice have reduced levels of IgG subclasses and that this was associated with significantly shorter half-lives for all IgG subclasses, excepting IgG2b (Israel et al., Citation1996). In other studies, B2m- and FcRn-deficient mice were also shown incapable of vigorous IgG immune responses against foreign antigens. Thus, B2m-deficient mice exhibited reduced antibody responses for all IgG subclasses to vaccinia virus infection and TNP-KLH immunization (Spriggs et al., Citation1992) and FcRn deficiency substantially reduced the anti-DNP response irrespective of the IgG subclass (Roopenian et al., Citation2003). Since B2m is required for a functional FcRn (Roopenian and Akilesh, Citation2007), the lower immunoglobulin levels in both B2m- and FcRn-deficient mice are believed to be from the absence of FcRn-mediated recycling of IgG (Lencer and Blumberg, Citation2005). In our studies, defective FcRn function is also likely responsible for the overall reduction in serum IgG associated with B2m deficiency and may also explain the reduction in ANoA. Interestingly, however, total IgG anti-chromatin autoantibody levels after mercury exposure in B2m-deficient mice were, in contrast, not reduced and only the IgG1 isotype was significantly lowered despite dramatic reductions in both the polyclonal IgG1 and IgG2a serum concentrations. Furthermore, kidney immune complex deposits were not reduced in B2m−/− mice exposed to mercury despite significantly lower IgG concentrations. In MRL/Faslpr mice IgG1 is selectively dependent upon B2m (Christianson et al., Citation1996) and NZB.B2m−/− mice have reduced IL-4 (Chen et al., Citation1997) suggesting that the B2m-dependent reduction of IgG1 in mHgIA may reflect changes in cytokine expression.
Previous studies have shown that although the cytokine and autoantibody isotype profile in mHgIA is TH2-like with greatest elevations in IL-4, IgE, and IgG1, the development of humoral autoimmunity and tissue pathology in mHgIA paradoxically requires the presence of IFNγ, but not IL-4 (Pietsch et al., Citation1989; Ochel et al., Citation1991; Kono et al., Citation1998). Here we observed that B2m deficiency resulted in a significant reduction in anti-chromatin IgG1, but not other IgG isotypes, suggesting the possibility that the cytokine profile might be directed away from the characteristic TH2 response (Ochel et al., Citation1991). Consistent with this, significant increases in IL-4 mRNA in the spleen and exposed skin were seen in wild-type mice exposed to mercury, while B2m−/− mice had no or smaller increases. We also observed a marked increase in IFNγ mRNA in mercury-exposed wild-type mice, but only in exposed skin, while IFNγ did not increase in B2m−/− mice, supporting the notion that increased IFNγ is necessary for full expression of disease (Kono et al., Citation1998). Although the cells responsible for IFNγ expression were not determined in this study, they likely include MHC Class I-restricted T-cells and NK cells (Boehm et al., Citation1997; Sun and Lanier, Citation2008). Thus, B2m is necessary for full expression of IL-4 and IFNγ following mercury exposure and the predominant TH2 response. Its role in overall autoantibody production is less certain as B2m deficiency did not affect total IgG anti-chromatin titers or severity of kidney immune complex deposits.
While the absence of B2m and changes in cytokine expression can be argued to have contributed to the IgG subclasses of the autoantibody responses, they do not explain the persistence of the anti-chromatin, but reduction in the ANoA response. This difference suggests a role for an antigen specific mechanism in maintaining circulating anti-chromatin antibody. Both DNA and nucleosomes have been detected in the blood of mice (Björkman et al., Citation2003) and humans (Williams et al., Citation2001; Chen et al., Citation2007) with systemic autoimmunity, presumably a by-product of cell death (Pisetsky, Citation2004, Citation2007). The immune-complexes formed upon interaction of circulating nucleosomes with anti-chromatin autoantibodies would be well-suited to bind FcγR and activate immune effector cells through endocytosis of the DNA-containing complexes and engagement of nucleic acid-binding toll-like receptors (TLRs) (Nimmerjahn and Ravetch, Citation2008), thereby maintaining the anti-chromatin response. By contrast, the ANoA response may be less effective in generating immune complexes and FcγR-mediated activation, as the release from dying cells of soluble fibrillarin (the major antigenic component of ANoA) is accompanied by proteolytic cleavage and loss of antigenicity (Pollard et al., Citation1997, Citation2000). Less effective FcγR-mediated activation, in addition to increased IgG catabolism, might explain the selective reduction in the ANoA response.
Despite reduced immunoglobulin levels, B2m-deficient mice exposed to mercury retained glomerular immune deposits, but had reduced deposits in vessels of the kidney compared to wild-type mice. Other indices of kidney function (viz. nephritis, proteinuria) may be impacted by B2m as noted in studies with idiopathic models (Chan et al., Citation2001; Christianson et al., Citation1996); however, mercury toxicity can make such studies challenging. Even a single exposure to HgCl2 results in tubular necrosis and proteinuria (Rumbeiha et al., Citation2000) and exposure in C57BL/6 mice, which do not develop renal immune deposits (Hultman et al., Citation1992), leads to proteinuria (Piacenza et al., Citation2009). Deficient FcRn expression in the kidneys of B2m-deficient mice might have contributed to the glomerular pathology observed in mHgIA. It has been proposed that in glomerulonephritis, IgG may be deposited at the basolateral side of the podocyte (Haymann et al., Citation2000; Roopenian and Akilesh, Citation2007). The absence of FcRn in B2m-deficient mice might be expected to limit transcytosis of multimeric IgG containing complexes via podocytes, leading to immune deposits even in the presence of a reduced IgG half-life. A persistence of anti-chromatin autoantibodies may also contribute to glomerular deposition because of the binding of antibody-nucleosome complexes to glomerular basement components (Kramers et al., Citation1994; van Bruggen et al., Citation1997).
Single gene deletions can influence expression of mHgIA in different ways ranging from no effect to suppression of one or more features of disease, or suppression of all features of disease (Pollard et al., Citation2003, Citation2005). This study provides the additional observation that lack of B2m leads to modulation of the autoantibody profile, a feature that has been observed for single gene deficiencies in other models of autoimmunity. The most notable being that observed in lupus-prone mice deficient in Toll-like receptors resulting in preferential effects on IgG subclasses and autoantibodies against DNA or RNA containing autoantigens (Baccala et al., Citation2007; Marshak-Rothstein and Rifkin, Citation2007; Kono et al., Citation2009). Differences in autoantibody profiles have also been found in xenobiotic-induced autoimmunity following pristane injection of IL-6 deficient mice (Richards et al., Citation1998). In contrast to B2m deficiency, absence of IL-6 led to a lack of anti-chromatin but persistence of anti-snRNP antibodies. The discordance of autoantibody profiles in mercury-exposed B2m-deficient mice is further evidence that multiple molecular pathways influence the autoantibody profiles of systemic autoimmunity.
Conclusion
These studies are the first to demonstrate that B2m is required for the full development of xenobiotic-induced systemic autoimmunity, with mercury-exposed B2m-deficient mice having significantly lower levels of polyclonal IgG and certain autoantibodies, reduced expression of IL-4 and IFNγ, but yet similar serum concentrations of IgG anti-chromatin and amounts of kidney immune complex deposits. These studies show that B2m is a potential genetic modifier of xenobiotic-induced systemic autoimmunity and document the complex manner in which genetic variation can alter autoimmune responses.
Declaration of interest
This publication was made possible by National Institutes of Health grant numbers ES007511 and ES014847 to KMP, ES08666, AR053731, and AR42242 to DHK, and Swedish Research Council, Branch of Medicine grant number 09453 to PH, and the Stein Endowment of the Department of Molecular and Experimental Medicine. M-L Eskilsson provided expert help in the preparation of the tissue immunopathology. This is publication 17325-MEM from The Scripps Research Institute (La Jolla, CA).
References
- Abréu Vélez A. M., Warfvinge, G., Herrera, W. L., Abréu Vélez C. E., Montoya M, F., Hardy, D. M., Bollag, W. B., and Hashimoto, K. 2003. Detection of mercury and other undetermined materials in skin biopsies of endemic pemphigus foliaceus. Am J Dermatopathol 25:384–391.
- Ahouse, J. J., Hagerman, C. L., Mittal, P., Gilbert, D. J., Copeland, N. G., Jenkins, N. A. and Simister, N. E. 1993. Mouse MHC class I-like Fc receptor encoded outside the MHC. J Immunol 151:6076–6088.
- Baccala, R., Hoebe, K., Kono, D. H., Beutler, B. and Theofilopoulos, A. N. 2007. TLR-dependent and TLR-independent pathways of type I interferon induction in systemic autoimmunity. Nat Med 13:543–551.
- Bell, S. A., Hobbs, M. V. and Rubin, R. L. 1992. Isotype-restricted hyperimmunity in a murine model of the toxic oil syndrome. J Immunol 148:3369–3376.
- Bendelac, A., Killeen, N., Littman, D. R. and Schwartz, R. H. 1994. A subset of CD4+ thymocytes selected by MHC class I molecules. Science 263:1774–1778.
- Björkman, L., Reich, C. F. and Pisetsky, D. S. 2003. The use of fluorometric assays to assess the immune response to DNA in murine systemic lupus erythematosus. Scand J Immunol 57:525–533.
- Boehm, U., Klamp, T., Groot, M. and Howard, J. C. 1997. Cellular responses to interferon-γ. Annu Rev Immunol 15:749–795.
- Burlingame, R. W. and Rubin, R. L. 1990. Subnucleosome structures as substrates in enzyme-linked immunosorbent assays. J Immunol Methods 134:187–199.
- Bustin, S. A. 2000. Absolute quantification of mRNA using real-time reverse transcription polymerase chain reaction assays. J Mol Endocrinol 25:169–193.
- Cauvi, D. M., Cauvi, G. and Pollard, K. M. 2007. Reduced expression of decay-accelerating factor 1 on CD4+ T cells in murine systemic autoimmune disease. Arthritis Rheum 56:1934–1944.
- Chan, O. T., Paliwal, V., McNiff, J. M., Park, S. H., Bendelac, A. and Shlomchik, M. J. 2001. Deficiency in β(2)-microglobulin, but not CD1, accelerates spontaneous lupus skin disease while inhibiting nephritis in MRL-Fas(lpr) nice: An example of disease regulation at the organ level. J Immunol 167:2985–2990.
- Chen, J. A., Meister, S., Urbonaviciute, V., Rödel, F., Wilhelm, S., Kalden, J. R., Manger, K. and Voll, R. E. 2007. Sensitive detection of plasma/serum DNA in patients with systemic lupus erythematosus. Autoimmunity 40:307–310.
- Chen, S. Y., Takeoka, Y., Pike-Nobile, L., Ansari, A. A., Boyd, R. and Gershwin, M. E. 1997. Autoantibody production and cytokine profiles of MHC class I (β2-microglobulin) gene deleted New Zealand black (NZB) mice. Clin Immunol Immunopathol 84:318–327.
- Christianson, G. J., Blankenburg, R. L., Duffy, T. M., Panka, D., Roths, J. B., Marshak-Rothstein, A. and Roopenian, D. C. 1996. β2-microglobulin dependence of the lupus-like autoimmune syndrome of MRL-lpr mice. J Immunol 156:4932–4939.
- Christianson, G. J., Brooks, W., Vekasi, S., Manolfi, E. A., Niles, J., Roopenian, S. L., Roths, J. B., Rothlein, R. and Roopenian, D. C. 1997. β 2-microglobulin-deficient mice are protected from hypergammaglobulinemia and have defective antibody responses because of increased IgG catabolism. J Immunol 159:4781–4792.
- Cooper, G. S., Parks, C. G., Treadwell, E. L., St Clair, E. W., Gilkeson, G. S. and Dooley, M. A. 2004. Occupational risk factors for the development of systemic lupus erythematosus. J Rheumatol 31:1928–1933.
- Gardner, R. M., Nyland, J. F., Silva, I. A., Ventura, A. M., de Souza, J. M. and Silbergeld, E. K. 2010. Mercury exposure, serum antinuclear/antinucleolar antibodies and serum cytokine levels in mining populations in Amazonian Brazil: A cross-sectional study. Environ Res 110:345–354.
- Ghetie, V., Hubbard, J. G., Kim, J. K., Tsen, M. F., Lee, Y. and Ward, E. S. 1996. Abnormally short serum half-lives of IgG in β2-microglobulin-deficient mice. Eur J Immunol 26:690–696.
- Giese, T. and Davidson, W. F. 1995. In CD8+ T cell-deficient lpr/lpr mice, CD4+B220+ and CD4+B220- T cells replace B220+ double-negative T cells as the predominant populations in enlarged lymph nodes. J Immunol 154:4986–4995.
- Giulietti, A., Overbergh, L., Valckx, D., Decallonne, B., Bouillon, R. and Mathieu, C. 2001. An overview of real-time quantitative PCR: Applications to quantify cytokine gene expression. Methods 25:386–401.
- Haymann, J. P., Levraud, J. P., Bouet, S., Kappes, V., Hagège, J., Nguyen, G., Xu, Y., Rondeau, E. and Sraer, J. D. 2000. Characterization and localization of the neonatal Fc receptor in adult human kidney. J Am Soc Nephrol 11:632–639.
- Hultman, P., Bell, L. J., Eneström, S. and Pollard, K. M. 1992. Murine susceptibility to mercury. I. Autoantibody profiles and systemic immune deposits in inbred, congenic and intra-H-2 recombinant strains. Clin Immunol Immunopathol 65:98–109.
- Hultman, P., Johansson, U. and Dagnaes-Hansen, F. 1995. Murine mercury-induced autoimmunity: The role of T-helper cells. J Autoimmun 8:809–823.
- Israel, E. J., Patel, V. K., Taylor, S. F., Marshak-Rothstein, A. and Simister, N. E. 1995. Requirement for a β2-microglobulin-associated Fc receptor for acquisition of maternal IgG by fetal and neonatal mice. J Immunol 154:6246–6251.
- Israel, E. J., Wilsker, D. F., Hayes, K. C., Schoenfeld, D. and Simister, N. E. 1996. Increased clearance of IgG in mice that lack β2-microglobulin: Possible protective role of FcRn. Immunology 89:573–578.
- Jiang, Y. and Möller, G. 1995. In vitro effects of HgCl2 on murine lymphocytes. I. Preferable activation of CD4+ T cells in a responder strain. J Immunol 154:3138–3146.
- Khare, S. D., Hansen, J., Luthra, H. S. and David, C. S. 1996. HLA-B27 heavy chains contribute to spontaneous inflammatory disease in B27/human β2-microglobulin (β2m) double transgenic mice with disrupted mouse β2m. J Clin Invest 98:2746–2755.
- Koh, D. R., Ho, A., Rahemtulla, A., Fung-Leung, W. P., Griesser, H. and Mak, T. W. 1995. Murine lupus in MRL/lpr mice lacking CD4 or CD8 T cells. Eur J Immunol 25:2558–2562.
- Kono, D. H., Balomenos, D., Pearson, D. L., Park, M. S., Hildebrandt, B., Hultman, P. and Pollard, K. M. 1998. The prototypic Th2 autoimmunity induced by mercury is dependent on IFN-γ and not Th1/Th2 imbalance. J Immunol 161:234–240.
- Kono, D. H., Haraldsson, M. K., Lawson, B. R., Pollard, K. M., Koh, Y. T., Du, X., Arnold, C. N., Baccala, R., Silverman, G. J., Beutler, B. A. and Theofilopoulos, A. N. 2009. Endosomal TLR signaling is required for anti-nucleic acid and rheumatoid factor autoantibodies in lupus. Proc Natl Acad Sci USA 106:12061–12066.
- Kotzin, B. L., Lafferty, J. A., Portanova, J. P., Rubin, R. L. and Tan, E. M. 1984. Monoclonal anti-histone autoantibodies derived from murine models of lupus. J Immunol 133:2554–2559.
- Kramers, C., Hylkema, M. N., van Bruggen, M. C., van de Lagemaat, R., Dijkman, H. B., Assmann, K. J., Smeenk, R. J. and Berden, J. H. 1994. Anti-nucleosome antibodies complexed to nucleosomal antigens show anti-DNA reactivity and bind to rat glomerular basement membrane in vivo. J Clin Invest 94:568–577.
- Lencer, W. I. and Blumberg, R. S. 2005. A passionate kiss, then run: Exocytosis and recycling of IgG by FcRn. Trends Cell Biol 15:5–9.
- Li, S. J., Zhang, S. H., Chen, H. P., Zeng, C. H., Zheng, C. X., Li, L. S. and Liu, Z. H. 2010. Mercury-induced membranous nephropathy: Clinical and pathological features. Clin J Am Soc Nephrol 5:439–444.
- Low, S. C. and Mezo, A. R. 2009. Inhibitors of the FcRn:IgG protein-protein interaction. AAPS J 11:432–434.
- Maldonado, M. A., Eisenberg, R. A., Roper, E., Cohen, P. L. and Kotzin, B. L. 1995. Greatly reduced lymphoproliferation in lpr mice lacking major histocompatibility complex class I. J Exp Med 181:641–648.
- Marshak-Rothstein, A. and Rifkin, I. R. 2007. Immunologically active autoantigens: The role of toll-like receptors in the development of chronic inflammatory disease. Annu Rev Immunol 25:419–441.
- Mixter, P. F., Russell, J. Q., Durie, F. H. and Budd, R. C. 1995. Decreased CD4-CD8- TCR-α β + cells in lpr/lpr mice lacking β2-microglobulin. J Immunol 154:2063–2074.
- Mozes, E., Lovchik, J., Zinger, H. and Singer, D. S. 2005. MHC class I expression regulates susceptibility to spontaneous autoimmune disease in (NZBxNZW)F1 mice. Lupus 14:308–314.
- Nimmerjahn, F. and Ravetch, J. V. 2008. Fcgamma receptors as regulators of immune responses. Nat Rev Immunol 8:34–47.
- Ochel, M., Vohr, H. W., Pfeiffer, C. and Gleichmann, E. 1991. IL-4 is required for the IgE and IgG1 increase and IgG1 autoantibody formation in mice treated with mercuric chloride. J Immunol 146:3006–3011.
- Ohteki, T., Iwamoto, M., Izui, S. and MacDonald, H. R. 1995. Reduced development of CD4-8-B220+ T cells but normal autoantibody production in lpr/lpr mice lacking major histocompatibility complex class I molecules. Eur J Immunol 25:37–41.
- Piacenza, F., Malavolta, M., Cipriano, C., Costarelli, L., Giacconi, R., Muti, E., Tesei, S., Pierpaoli, S., Basso, A., Bracci, M., Bonacucina, V., Santarelli, L. and Mocchegiani, E. 2009. l-Arginine normalizes NOS activity and zinc-MT homeostasis in the kidney of mice chronically exposed to inorganic mercury. Toxicol Lett 189:200–205.
- Pietsch, P., Vohr, H. W., Degitz, K. and Gleichmann, E. 1989. Immunological alterations inducible by mercury compounds. II. HgCl2 and gold sodium thiomalate enhance serum IgE and IgG concentrations in susceptible mouse strains. Int Arch Allergy Appl Immunol 90:47–53.
- Pisetsky, D. S. 2004. The immune response to cell death in SLE. Autoimmun Rev 3:500–504.
- Pisetsky, D. S. 2007. The role of nuclear macromolecules in innate immunity. Proc Am Thorac Soc 4:258–262.
- Pollard, K. M., Arnush, M., Hultman, P. and Kono, D. H. 2004. Costimulation requirements of induced murine systemic autoimmune disease. J Immunol 173:5880–5887.
- Pollard, K. M. and Hultman, P. 1997. Effects of mercury on the immune system. Met Ions Biol Syst 34:421–440.
- Pollard, K. M., Hultman, P. and Kono, D. H. 2003. Using single-gene deletions to identify checkpoints in the progression of systemic autoimmunity. Ann N Y Acad Sci 987:236–239.
- Pollard, K. M., Hultman, P. and Kono, D. H. 2005. Immunology and genetics of induced systemic autoimmunity. Autoimmun Rev 4:282–288.
- Pollard, K. M., Hultman, P. and Kono, D. H. 2010. Toxicology of autoimmune diseases. Chem Res Toxicol 23:455–466.
- Pollard, K. M. and Landberg, G. P. 2001. The in vitro proliferation of murine lymphocytes to mercuric chloride is restricted to mature T cells and is interleukin 1 dependent. Int Immunopharmacol 1:581–593.
- Pollard, K. M., Lee, D. K., Casiano, C. A., Bluthner, M., Johnston, M. M. and Tan, E. M. 1997. The autoimmunity-inducing xenobiotic mercury interacts with the autoantigen fibrillarin and modifies its molecular and antigenic properties. J Immunol 158:3521–3528.
- Pollard, K. M., Pearson, D. L., Blüthner, M. and Tan, E. M. 2000. Proteolytic cleavage of a self-antigen following xenobiotic-induced cell death produces a fragment with novel immunogenic properties. J Immunol 165:2263–2270.
- Pollard, K. M., Pearson, D. L., Hultman, P., Hildebrandt, B. and Kono, D. H. 1999. Lupus-prone mice as models to study xenobiotic-induced acceleration of systemic autoimmunity. Environ Health Perspect 107 Suppl 5:729–735.
- Richards, H. B., Satoh, M., Shaw, M., Libert, C., Poli, V. and Reeves, W. H. 1998. Interleukin 6 dependence of anti-DNA antibody production: Evidence for two pathways of autoantibody formation in pristane-induced lupus. J Exp Med 188:985–990.
- Roopenian, D. C. and Akilesh, S. 2007. FcRn: The neonatal Fc receptor comes of age. Nat Rev Immunol 7:715–725.
- Roopenian, D. C., Christianson, G. J., Sproule, T. J., Brown, A. C., Akilesh, S., Jung, N., Petkova, S., Avanessian, L., Choi, E. Y., Shaffer, D. J., Eden, P. A. and Anderson, C. L. 2003. The MHC class I-like IgG receptor controls perinatal IgG transport, IgG homeostasis and fate of IgG-Fc-coupled drugs. J Immunol 170:3528–3533.
- Rumbeiha, W. K., Fitzgerald, S. D., Braselton, W. E., Roth, R. A., Pestka, J. J. and Kaneene, J. B. 2000. Augmentation of mercury-induced nephrotoxicity by endotoxin in the mouse. Toxicology 151:103–116.
- Santarelli, L. Bracci, M. and Mocchegiani, E. 2006. In vitro and in vivo effects of mercuric chloride on thymic endocrine activity, NK and NKT cell cytotoxicity, cytokine profiles (IL-2, IFN-γ, IL-6): Role of the nitric oxide-L-arginine pathway. Int Immunopharmacol 6:376–389.
- Schiraldi, M. and Monestier, M. 2009. How can a chemical element elicit complex immunopathology? Lessons from mercury-induced autoimmunity. Trends Immunol 30:502–509.
- Silva, I. A., Nyland, J. F., Gorman, A., Perisse, A., Ventura, A. M., Santos, E. C., Souza, J. M., Burek, C. L., Rose, N. R. and Silbergeld, E. K. 2004. Mercury exposure, malaria and serum antinuclear/antinucleolar antibodies in Amazon populations in Brazil: A cross-sectional study. Environ Health 3:11.
- Spriggs, M. K., Koller, B. H., Sato, T., Morrissey, P. J., Fanslow, W. C., Smithies, O., Voice, R. F., Widmer, M. B. and Maliszewski, C. R. 1992. β2-microglobulin-, CD8+ T-cell-deficient mice survive inoculation with high doses of vaccinia virus and exhibit altered IgG responses. Proc Natl Acad Sci USA 89:6070–6074.
- Sun, J. C. and Lanier, L. L. 2008. Cutting edge: Viral infection breaks NK cell tolerance to “missing self”. J Immunol 181:7453–7457.
- van Bruggen, M. C., Walgreen, B., Rijke, T. P., Tamboer, W., Kramers, K., Smeenk, R. J., Monestier, M., Fournie, G. J. and Berden, J. H. 1997. Antigen specificity of anti-nuclear antibodies complexed to nucleosomes determines glomerular basement membrane binding in vivo. Eur J Immunol 27:1564–1569.
- Vas, J., Mattner, J., Richardson, S., Ndonye, R., Gaughan, J. P., Howell, A. and Monestier, M. 2008. Regulatory roles for NKT cell ligands in environmentally induced autoimmunity. J Immunol 181:6779–6788.
- Vas, J. and Monestier, M. 2008. Immunology of mercury. Ann N Y Acad Sci 1143:240–267.
- Williams, R. C. Jr, Malone, C. C., Meyers, C., Decker, P. and Muller, S. 2001. Detection of nucleosome particles in serum and plasma from patients with systemic lupus erythematosus using monoclonal antibody 4H7. J Rheumatol 28:81–94.
- Yang, J. M., Baserga, S. J., Turley, S. J. and Pollard, K. M. 2001. Fibrillarin and other snoRNP proteins are targets of autoantibodies in xenobiotic-induced autoimmunity. Clin Immunol 101:38–50.
- Zijlstra, M., Bix, M., Simister, N. E., Loring, J. M., Raulet, D. H. and Jaenisch, R. 1990. β2-microglobulin deficient mice lack CD4-8+ cytolytic T cells. Nature 344:742–746.
- Zijlstra, M., Li, E., Sajjadi, F., Subramani, S. and Jaenisch, R. 1989. Germ-line transmission of a disrupted β2-microglobulin gene produced by homologous recombination in embryonic stem cells. Nature 342:435–438.