Abstract
Exposure to environmental agents can compromise numerous immunological functions. Immunotoxicology focuses on the evaluation of the potential adverse effects of xenobiotics on immune mechanisms that can lead to harmful changes in host responses such as: increased susceptibility to infectious diseases and tumorigenesis; the induction of hypersensitivity reactions; or an increased incidence of autoimmune disease. In order to assess the immunosuppressive response to short-term exposure to some commonly used pesticides, the studies here focused on the response of mice after exposures to the organochlorine pesticide methoxychlor, the organophosphorus pesticide parathion, or the agricultural insecticide synergist piperonyl butoxide. In these studies, 7-week-old mice were orally administered (by gavage) methoxychlor, parathion, or piperonyl butoxide daily for five consecutive days. On Day 2, all mice in each group were immunized with sheep red blood cells (SRBC), and their SRBC-specific IgM responses were subsequently assessed. In addition, levels of B-cells in the spleen of each mouse were also analyzed via surface antigen expression. The results of these studies indicated that treatments with these various pesticides induced marked decreases in the production of SRBC-specific IgM antibodies as well as in the expression of surface antigens in IgM- and germinal center-positive B-cells. Based on these outcomes, it is concluded that the short-term exposure protocol was able to detect potential immunosuppressive responses to methoxychlor, parathion, and piperonyl butoxide in situ, and, as a result, may be useful for detecting other environmental chemical-related immunotoxicities.
Introduction
Exposure to environmental agents can compromise numerous immunological functions. In the United States alone, 20,000 pesticide products are on the market, and 1 billion pounds of active ingredients are applied annually for agricultural, industrial, and residential pest control (EPA, Citation2003). Against this background, studies in animals and humans have indicated that the immune system is a potential target, and that damage to this system can be associated with increased morbidity and even mortality. Immunotoxicologic analyses can evaluate the potential adverse effects of xenobiotics (e.g. chemicals, pesticides, drugs, biotechnology-derived products) on host immune mechanisms. Many of these effects can lead to harmful changes in host responses, including increased susceptibility to infectious diseases and tumorigenesis, the induction of hypersensitivity reactions, or an increased incidence of autoimmune disease (Herzyk and Holsapple, Citation2007).
Immunotoxicological testing has emerged in recent years as an important adjunct to routine safety evaluations of environmental chemicals and newly-developed pharmaceuticals, and has been incorporated into the guidelines issued by several regulatory authorities, including the Environmental Protection Agency (EPA. Citation1998), Food and Drug Administration (FDA, Citation2002), the European Medicines Agency (EMEA) (CPMP, Citation2000), and the International Conference on Harmonization (ICH, Citation2006). The most recent immunotoxicology guidance documents recommend T-dependent antigen response (TDAR) tests, primarily because this assay represents a comprehensive evaluation of immune function based on an assessment of various components of the immune system (e.g., antigen-presenting cells, T-helper lymphocytes, and B-lymphocytes) involved in an antigen-specific antibody response (White et al., Citation2010).
Originally this test was configured as an IgM/complement dependent ex vivo plaque-forming cell (PFC) assay in mice, with sheep red blood cells (SRBC) as both the immunogen and the target for complement-mediated lysis. Subsequently, an enzyme-linked immunosorbent assay (ELISA)-based format was developed using SRBC as both the immunogen and antigen in the antibody detection system (Temple et al., Citation1993). SRBC-based TDAR assays (PFC and ELISA) have also been used in rats, and have ultimately gained acceptance as validated tests for detecting the immunosuppressant activity of drugs and chemicals (Ladics et al., Citation1998).
The above guidelines generally recommend using repeated doses, 28-day exposures, and adult animals to detect immunotoxicity caused by environmental chemicals. The use of long-term exposure offers the advantage of being able to compare results with those obtained from general toxicity tests. However, long-term exposure is time-consuming, costly, and may lead to immunotoxic drug resistance because the immune system is highly sensitive to the toxic effects of several types of chemicals (Fukuyama et al., Citation2011a); such a change would distort estimates of advanced immunotoxicity. Therefore, new short-term exposure protocols are needed to detect immunotoxicity. Previously, in the first stage of our studies, we developed a short-term method (administration via oral gavage for 3 days) for detecting thymocyte apoptosis induced by a typical immunosuppressant, methoxychlor (Fukuyama et al., Citation2010, Citation2011a). Indeed, methoxychlor induced prominent increases in several parameters indicative of induced thymocyte apoptosis, including Annexin V-FITC+ cells, caspase (3/7, 8, and 9) activities, and DNA fragmentation. This type of dysregulation of apoptosis in the thymus is known to lead to various immune disorders, including immunodeficiency, tumorigenesis, allergies, and autoimmunity (Zhang et al., Citation2005). Our previous results demonstrated that short-term exposure has the potential to detect the immunosuppression caused by chemicals present in the environment.
In light of these previous results, the aim of this study was to develop a new short-term immunotoxicology protocol using several immunologic endpoints that tested short-term exposure to the organochlorine pesticide methoxychlor, the organophosphorus pesticide parathion, and the agricultural insecticide synergist piperonyl butoxide. These three chemicals were chosen on the basis of previous studies; parathion markedly inhibits antigen-specific-IgM production (Casale et al., Citation1984), and we previously showed that methoxychlor exposure results in atrophy of CD4+CD8+ T-lymphocytes in the thymus (Takeuchi et al., Citation2002, Citation2004; Fukuyamaet al., Citation2011b). Piperonyl butoxide is an agricultural insecticide synergist used mainly with pyrethroids (Carsonet al., 1988; Mitsumori et al., Citation1996; Emerson et al., Citation2001). Recent evidence suggests that piperonyl butoxide administration depletes T-lymphocytes in the spleen and thymus, induces hypoplasia of the bone marrow, and inhibits T-lymphocyte proliferation in lymphoid tissues (Mitsumori et al., Citation1996; Diel et al., Citation1999; Battaglia et al., Citation2010).
Materials and methods
Chemicals
Standard parathion (C10H14NO5PS, 99.5% pure), standard methoxychlor (C16H15Cl3O2, > 97% pure), standard piperonyl butoxide (C19H30O5, > 98% pure), dimethyl sulfoxide (DMSO), and acetone were purchased from Wako Pure Chemical Industries, Ltd. (Osaka, Japan). Corn oil was purchased from Hayashi Chemicals (Tokyo, Japan). For in vitro studies, parathion was dissolved in DMSO to 0.1% (w/v). Methoxychlor and piperonyl butoxide were dissolved in acetone to 0.1% (w/v).
Three concentrations (10, 100, and 1000 nmol/ml) of these pesticides were selected for use in the in vitro portions of these studies on the basis of results from preliminary cell viability analyses (data not shown); the exact concentrations chosen were based on the ability of each given agent to substantially inhibit cell viability but not cause 100% lethality.
For the in vivo portions of these studies, oral administration was used to introduce parathion, methoxychlor, and piperonyl butoxide (diluted in corn oil to a fixed final volume) into murine hosts. Based on the EPA Immunotoxicity Guidelines established in 1998, the highest dose level used in a host should ‘not produce significant stress, malnutrition, or fatalities’. Accordingly, in this study, the maximum doses used were selected to be < 1/3 of the LD50 (dose at which ≥ 50% of animals would be expected to die) and concurrently to avoid the induction of clear systemic toxicity (i.e. changes in appearance, posture, behavior, respiration, consciousness, neurologic status, body temperature, excretion, etc.). The actual doses used are presented in
Table 1. Chemical doses used.
Note, there is no overt relationship between the doses used in the in vivo and in vitro portions of these studies. The endpoints to be measured in each respective series of studies are distinct, and the information gleaned from the in vitro studies were only meant to be used for helping to explain outcomes (related to immunomodulation, etc.) that might appear in the agent-treated mice.
Animals
Female C3H/HeN (6-weeks-old) mice were purchased from Charles River Japan Laboratories (Atsugi, Kanagawa, Japan) and housed individually under controlled lighting (lights on from 07:00 to 19:00 h), temperature (22 ± 3°C), humidity (55% ± 15%), and ventilation (at least 10 complete fresh-air changes hourly). Based on the EPA guideline (1998), mice are a model species recommended for use in immunotoxicity studies that test effects of agricultural chemicals (see as was done in Casale et al., Citation1984; Diel et al., Citation1999; Battaglia et al., Citation2010). Furthermore, for the immunotoxicity study, only one gender needed to be evaluated; in general, female animals are considered to yield more consistent outcomes than are male counterparts during evaluation of effects from test articles on host humoral immune responses. Therefore, in this study, female C3H/HeN were used. These particular hosts were also selected as our laboratory has historical immunotoxicity study data on this strain (data not shown). Food (Certified Pellet Diet MF, Oriental Yeast Co., Tokyo, Japan) and water were available ad libitum. This study was conducted in accordance with the Code of Ethics for Animal Experimentation of the Institute of Environmental Toxicology.
Cell culture
The human acute T-cell leukemia cell line Jurkat E6.1 was obtained from DS Pharma Biomedical Co., Ltd. (Tokyo, Japan). Jurkat E6.1 cells were cultured in 70-ml EasYFlasks (Nalge Nunc International K.K., Tokyo, Japan) in 5 ml of RPMI 1640 (Gibco, Tokyo, Japan) supplemented with 10% heat-inactivated fetal calf serum (FCS; Gibco) at 37°C in a 5% CO2 atmosphere. The medium was changed three times per week.
Chemical treatment of the cells
The Jurkat E6.1 cells were seeded into flasks at 8 × 105 cells/ml. Quadruplicate flasks per dose per agent were then treated with 0, 10, 100, and 1000 nmol/ml of each test agent. Control cell cultures received vehicle-containing medium only. In all cases, the flasks were incubated for 30 min to 24 h at 37°C under 5% CO2. At the end of the exposure time, the culture medium (containing all cells that were originally seeded, i.e. both live and dead) from each flask was transferred to a 5 ml tube and used in apoptosis assays. In general, to assess the extent of apoptosis, caspase-3/7, -8, and -9 activities as well as the proportions of AnnexinV-FITC+ and caspase-3+ cells were quantified.
Cell viability and caspase (3/7, 8, and 9) activity
Cell viability was determined in cultured cells by quantitation of ATP, an endpoint that is indicative of metabolically-active cells. Cell viability, caspase-3/7, -8, and -9 activities were measured at 30 min, 1, 2, 4, and 24 h after initiation of exposure to the chemicals by using a luciferin-luciferase system (CellTiter-Glo™ Luminescent Cell Viability Assay and Caspase-Glo™ assay, Promega, Tokyo, Japan). From each cell suspension, 3 × 104 cells were seeded in duplicate into opaque-walled 96-well plates (Corning Japan K.K., Tokyo, Japan). A microplate luminometer (Spectra MAX L, Molecular Devices, Osaka, Japan) was used to measure the caspase activities in relative light units (RLU).
Annexin-V and caspase-3 cell staining and flow cytometric analysis
Flow cytometric analysis of apoptotic cells was performed at 1 and 4 h after initiation of exposure by staining cells with fluorescein isothiocyanate (FITC)-conjugated Annexin-V, FITC-conjugated caspase-3, and propidium iodide. To assay the cells, an Annexin-V:FITC Apoptosis Detection Kit and an FITC Active Caspase-3 Apoptosis Kit (BD Pharmingen, San Diego, CA) was used, in accordance with manufacturer protocols. All samples were subsequently analyzed using a FACSCaliber flow cytometer (BD Pharmingen) and its accompanying Cell Quest program. For each sample, 10,000 events were collected and analyzed for antigen expression.
Chemical exposure of mice
After a 1-week acclimatization period, C3H/HeN mice (7-weeks-old) were allocated randomly to groups (n = 6 mice/group) for dosing, vehicle control, and no treatment (naïve group). Chemical dosages were as follows: methoxychlor, 3, 30, and 300 mg/kg/day; parathion, 0.015, 0.15, and 1.5 mg/kg/day: or piperonyl butoxide, 3, 30, and 300 mg/kg/day. On Days 1–5, mice were given an oral dose (by gavage, without anesthesia) of the test solution (methoxychlor, parathion, or piperonyl butoxide) or vehicle. On Day 2, a solution of sheep red blood cells (SRBC, 6 × 107 cells/animal; Nippon Bio-Supp. Center, Tokyo, Japan) was injected via the tail vein into all test and control mice for immunization.
One day after the last oral administration (i.e. on Day 6 of study), all mice were anesthetized and sacrificed by over-anesthesization with diethyl ether. Blood samples were taken from the inferior vena cava, and serum samples were assayed for SRBC-specific serum IgM. Each animal’s spleen was removed and pooled in phosphate-buffered saline (PBS; Gibco). Single-cell suspensions of splenocytes in 5 ml of modified Eagle medium supplemented (MEM; Gibco) containing 5% heat-inactivated FCS were prepared by passage through a stainless-steel screen and sterile 70-µm nylon cell strainers (Falcon, Tokyo, Japan). The number of lymphocytes in the spleen preparation was then determined using a Coulter counter Z2 (Beckman Coulter, Tokyo, Japan).
SRBC-specific IgM responses in serum
Levels of SRBC-specific serum IgM were determined using a modified version of the method of Temple et al. (Citation1993). In brief, SRBC-membrane antigen was extracted with Tris-HCL and 0.1% SDS in PBS. The samples were then extensively dialyzed for 2 days against PBS. The protein content of each conjugated sample was determined by the method of Lowry et al. (Citation1951). Specific serum IgM was then measured by means of an ELISA using flat-bottomed microplates (Nalge Nunc) whose wells had been coated with SRBC-membrane antigen (2 µg/ml in coating buffer; BD Pharmingen) during an overnight incubation at 4°C. Following washing of each well five times with wash buffer (BD Pharmingen), and blocking of potential non-specific binding by incubation with assay diluent (BD Pharmingen) for 2 h at room temperature (RT), dilutions of each mouse serum sample (in assay diluent, from 1:4 to 1:16,384) was added to each well and the plates incubated for 2 h at RT. After gentle rinsing with wash buffer to remove all unbound materials, peroxidase conjugated anti-mouse IgM (secondary antibody; Rochland Inc. PA, dilution 1:15,000) was added to each well and the plate incubated for 2 h at RT. The wells were then rinsed again to remove non-adherent anti-mouse IgM. Finally, to quantify the amount of bound antibodies in each well, tetramethylbenzidine (TMB; 100 µl/well) substrate was added to each well and the plate incubated in the dark at RT for 30 min. Optical density measurements were then made at 450 nm in a Spectra MAX 190 microplate reader (Molecular Devices, Osaka, Japan).
IgM plaque-forming cell response to SRBC in splenocytes
The IgM plaque-forming cell (PFC) response to SRBC was determined by using a modified version of the methods of Cunningham (Citation1965) and Jerne and Nordin (Citation1963). Briefly, 1 × 106 cells were incubated with 1% SRBC and a 1:30 dilution of guinea pig complement (Denka Seiken Co., Tokyo) for 10 min at 4°C. The cells were applied to a Cunning-ham chamber (Takahashi Giken Glass Co., Ltd, Tokyo, Japan), and incubated for 1.5 h at 37°C in a 5% CO2 atmosphere. The number of plaques in each sample was then counted using a stereo-microscope.
Flow cytometric analysis
Isolated splenocytes were stained with fluorescein isothiocyanate (FITC)-conjugated peanut agglutinin (Vector Laboratories, Inc., Burlingame, CA), FITC-conjugated rat anti-mouse IgM (R6-60.2; BD), phycoerythrin (PE)-conjugated rat anti-mouse IgD (clone 11–26c.2a; BD), PE-cyano dye (Cy)5–conjugated rat anti-mouse CD45R/B220 (clone RA3-6B2; BD), and/or peridinin chlorphyll protein (PerCP)-Cy5.5–conjugated rat anti-mouse CD19 (clone 1D3; BD) to perform the flow analyses. To avoid non-specific binding, 1 × 106 cells were incubated with 20% normal goat serum (Sigma, St. Louis, MO) for 10 min at 4°C; this was followed by incubation with FITC-, PE-, PE-Cy5-, and PerCP-Cy5.5–conjugated monoclonal antibodies for 30 min at 4°C in the dark. The cells were washed twice with FCS-MEM, re-suspended at 1 × 106 cells per tube in 1 ml of PBS, and then analyzed with the FACSCaliber flow cytometer and its Cell Quest program. For each sample, 10,000 events were collected and analyzed for antigen expression.
Statistical analysis
The data were transformed logarithmically to equalize the variance, and analysis of variance (ANOVA) was used to evaluate the results. For statistically significant ANOVA, the differences between groups were assessed by using Dunnett’s multiple comparison test. A p-value < 0.05 was considered to indicate statistical significance.
Results
Preliminary in vitro study (confirmation of apoptosis)
In vitro analyses were performed to evaluate the extent of apoptosis resulting from parathion, methoxychlor, or piperonyl butoxide treatment. Caspase-3/7 activities (,, and ) increased significantly relative to control levels in the methoxychlor (1–24 h: 1000 nmol), parathion (2–24 h: 1000 nmol), and piperonyl butoxide (0.5–24 h: 1000 nmol) treatment groups. Caspase-8 activity (, , and ) increased significantly relative to control values in the methoxychlor (4 h: 1000 nmol) and piperonyl butoxide (2–4 h: 1000 nmol) treatment groups. Caspase-9 activity (, , and ) increased significantly relative to control levels in the methoxychlor (2–4 h: 1000 nmol, 24 h: 100 and 1000 nmol) and piperonyl butoxide (2–24 h: 1000 nmol) treatment groups.
Figure 1. Caspase-3/7, -8, and -9 activities in Jurkat T-cells treated with test chemicals for 10 min to 24 h. Caspase-3/7 (a: Methoxychlor; d: Parathion; g: Piperonyl butoxide), Caspase-8 (b: Methoxychlor; e: Parathion; h: Piperonyl butoxide), and Caspase-9 (c: Methoxychlor; f: Parathion; i: Piperonyl butoxide). All activities are expressed as mean (RLU) ± SD. Value significantly differs from control at * p < 0.05 and ** p < 0.01.
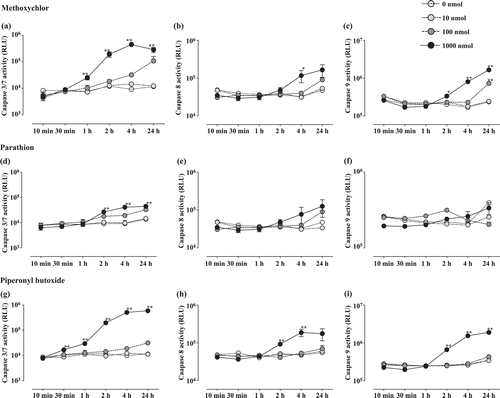
Flow cytometric analysis was used to evaluate the extent of apoptosis resulting from methoxychlor, parathion, or piperonyl butoxide treatment. The analyses revealed that the proportion of Annexin-V+PI− cells () increased significantly relative to the same values for the controls in the methoxychlor (1 h: 1000 nmol, 4 h: 100 and 1000 nmol), parathion (1 and 4 h: 1000 nmol), and piperonyl butoxide (1 and 4 h: 1000 nmol) treatment groups. The proportion of caspase-3+ cells () increased significantly relative to those seen in the controls as a result of the methoxychlor (1 h: 1000 nmol, 4 h: 100 and 1000 nmol), parathion (4 h: 100 and 1000 nmol), and piperonyl butoxide (4 h: 1000 nmol) treatments.
Figure 2. Annexin V-FITC positive cells among Jurkat T-cells treated with test chemicals for 1 or 4 h. (a) Methoxychlor; (b) Parathion; and (c) Piperonyl butoxide. Annexin V+-FITC+ cells are expressed as the mean (proportion of total, %) ± SD. Value significantly differs from control at * p< 0.05 and ** p < 0.01.
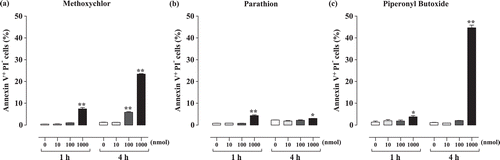
In vivo study (confirmation of anti-SRBC IgM responses in mice)
The SRBC-specific IgM responses in serum are shown in The 30 and 300 mg methoxychlor/kg, as well as the 1.5 mg parathion/kg, treatments caused a significant decrease in responses relative to those seen with the vehicle controls. The piperonyl butoxide treatments cause a decreasing trend, but this was mild; no significant differences from control were noted.
Figure 4. Anti-SRBC IgM response in C3H/He female mice treated with test chemicals. (a) SRBC-specific IgM response in serum. (b) Splenocyte IgM plaque-forming cell responses to SRBC. Values (titer dilution and cell counts) are expressed as mean ± SD. Value significantly differs from control at * p < 0.05 and ** p < 0.01.
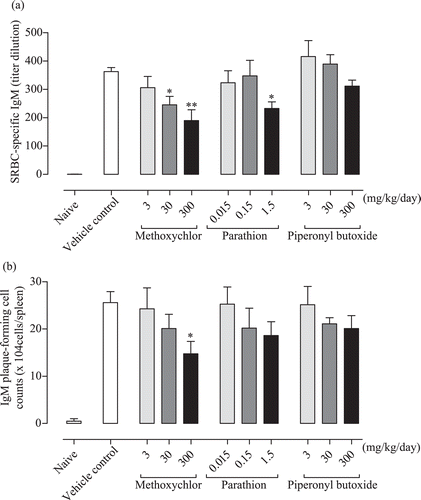
The IgM plaque-forming cell (PFC) responses to SRBC in splenocyte are shown in . The 300 mg methoxychlor/kg treatment caused a significant decrease in this parameter relative to the value seen with the vehicle controls. Both the parathion and piperonyl butoxide treatments led to a decreasing trend in this measure, but these decreases were mild and the final values did not significantly differ from the control values.
In vivo study (flow cytometric analysis)
To evaluate the activation of B-lymphocytes in the spleen following methoxychlor, parathion, and piperonyl butoxide treatment, flow cytometric analysis was performed using the lymphocytes stained with anti-CD19, -CD45R/B220, -IgD, -IgM, and -peanut agglutinin antibodies. Total cell counts in the spleen are shown in . The piperonyl butoxide 300 mg/kg treatment caused a significant decrease in this value relative to that in the vehicle controls. The methoxychlor treatments led to a decreasing trend as well, but no significant differences were noted. The numbers and ratio of IgM-positive B-lymphocytes (B220+IgD+IgM+) are shown in and . The 300 mg methoxychlor/kg, 1.5 mg parathion/kg, and the 30 and 300 mg piperonyl butoxide/kg treatments led to a significant decrease relative to values seen in the corresponding cells from vehicle control hosts. The numbers and ratio of germinal center B-lymphocytes (CD19+PNA+) are shown in and . The treatments with 3, 30, and 300 mg methoxychlor/kg, 1.5 mg parathion/kg, and 3, 30, and 300 mg piperonyl butoxide/kg caused significant decreases in this parameter relative to the values noted with the vehicle controls.
Figure 5. Flow cytometric analyses of splenocytes from C3H/He female mice treated with test chemicals. (a) Total cell counts; (b) germinal center-positive B-lymphocyte counts (CD19+PNA+); (c) germinal center-positive B-lymphocyte counts (CD19+PNA+, proportion of total, %); (d) IgM+ B-lymphocyte counts (B220+IgD+IgM+); and (e) IgM+ B-lymphocyte counts (B220+IgD+IgM+, proportion of total, %) in spleen. Counts are expressed as mean ± SD. Value significantly differs from control at * p < 0.05 and ** p < 0.01.
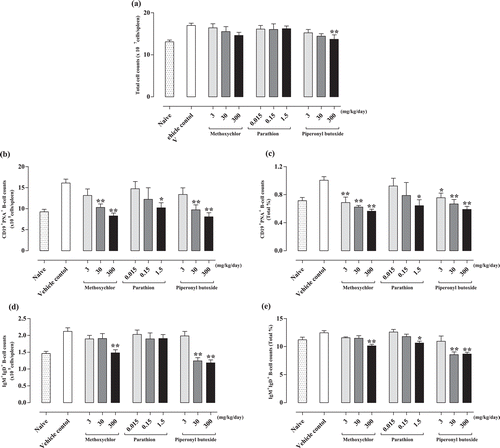
Discussion
Our primary objective in this study was to improve upon the current method of detecting environmental chemical-related immunotoxicity. To that end, we exposed mice by using a short-term exposure protocol (i.e. 5 days) to commonly used immunosuppressive chemicals, namely the organochlorine agent methoxychlor, the organophosphorus agent parathion, and the agricultural insecticide synergist piperonyl butoxide. We then assessed the effects of this short-term exposure via several types of detection methods, including induction of lymphocyte apoptosis in Jurkat T-cells, anti-SRBC IgM responses in serum and spleen, and numbers of IgM- and germinal center-positive B-lymphocytes in the spleen. Our results showed that methoxychlor, parathion, and piperonyl butoxide each could modulate the apoptosis of Jurkat T-cells in vitro. To assess apoptosis, caspase activities (3/7, 8, and 9), and the proportion of Annexin V- and caspase-3+ cells were examined. In mice, chemical-related immunotoxicity was detected by using our short-term exposure protocol. Indeed, all three chemicals induced prominent immuno-suppressive responses, including reducing the anti-SRBC IgM response (SRBC-specific IgM levels in serum and the IgM PFC response to SRBC in splenocyte), and the numbers of IgM- and germinal center-positive B-lymphocytes in the spleen.
Apoptosis is an essential process underlying multi-cellular organism development and function. In the immune system, apoptosis is required for lymphocyte development and homeostasis. Dysregulation of apoptosis leads to a variety of immune disorders, including immunodeficiency, tumorogenesis, allergies, and autoimmunity (Zhang et al., Citation2005). Detection of chemical-induced thymocyte apoptosis in vivo is difficult, however, because of the rapid clearance of apoptotic cells by phagocytes (Savill and Haslett, Citation1995; Kamath et al., Citation1997; Pryputniewicz et al., Citation1998). Therefore, in the current study, we first confirmed the immunosuppressive effects of methoxychlor, parathion, and piperonyl butoxide by assessing changes in the occurrence of apoptosis in a human leukemia cell line, Jurkat E6.1.
We observed a significant increase in caspase activities (3/7, 8, and 9) and in the proportion of Annexin V+ and caspase-3+ cells that resulted from exposure to the test chemicals (). Annexin V+ staining is reported to detect apoptosis at an early stage, allowing visualization of alterations in the cell membrane that occur when phosphatidylserine residues move to the external leaflet of the plasma membrane (Morris et al., Citation1984; Vermeset al., 1995). Caspases are required in the apoptotic pathway; therefore, measurement of caspases-3/7, -8, and -9 reliably indicates caspase-dependent apoptosis (Gurtu et al., Citation1997). Caspase-3 and -7 were the first caspases linked to apoptosis, and, together with caspase-8 and -9, act upstream of DNA fragmentation in the pathway. Our findings suggest that methoxychlor and piperonyl butoxide induced T-lymphocyte apoptosis in vitro via several caspase pathways. In contrast, parathion induced T-lymphocyte apoptosis via mainly the caspase-3 pathway. The immunomodulation demonstrated in vitro in this study may reflect what could occur in situ, i.e. these types of reactions in a growing fetus might predispose the highly sensitive fetal immune system to a loss of tolerance to self-antigens and lead to a subsequent increased risk for autoimmune disease and allergies as the offspring develops after birth.
On the basis of our preliminary in vitro data, we then performed our primary study using female C3H/He mice (7-weeks-old). To detect environmental chemical-related immunotoxicity, we focused on short-term exposure (i.e., 5 days) and several immune endpoints. Among them, we used a T-dependent antigen response (TDAR) test primarily and measured the antigen (SRBC)-specific IgM response using an ELISA and a PFC assay. There is no doubt that the antigen-specific IgM antibody plays a key role in all types of immune response to immunotoxic chemicals (Anderson et al., Citation2006). The SRBC-specific IgM ELISA utilizes solubilized, hemoglobin-free SRBC membranes, and measures SRBC-specific antibodies in serum that are generated by all antibody-producing tissues (i.e. spleen, lymph nodes, bone marrow), reflecting the systemic humoral immune response (Temple et al., Citation1993). On the other hand, the PFC assay has been well characterized across multiple labs, and is likely the most validated endpoint in immunotoxicology.
Combining the use of these two assays allows for studies to evaluate mechanisms of action of xenobiotic-induced immunotoxicity (Herzyk and Holsapple, Citation2007). In our study, methoxychlor, parathion, and piperonyl butoxide induced a prominent decrease both in antigen-specific serum IgM levels and IgM PFC counts compared to the vehicle controls. Generally speaking, these chemicals have immunosuppressive effects, and our preliminary study suggests that apoptosis was induced in Jurkat T-cells. Therefore, this down-regulation demonstrates that our protocol and methods were effective for identifying environmental chemical-related immunotoxicity.
In addition to the serum and spleen antigen-specific IgM responses, we analyzed the IgM- and IgD-expressing B-cell populations in spleen by use of flow cytometry. The first antibodies to be produced in a humoral immune response are always IgM, because IgM can be expressed without isotype switching. Surface IgM- and IgD-expressing B-cells are necessary for IgM production (Janeway et al., Citation2004). According to our results, significant decreases in the IgM-expressing B-lymphocyte population were observed in both the methoxychlor- and piperonyl butoxide-treated groups (). This down-regulation of IgM-expressing B-lymphocytes would thus be a useful endpoint for identifying chemical-related immunotoxicity.
Some B-cells are activated at the T-/B-lymphocyte border and migrate to form a germinal center within a primary follicle. Germinal centers are sites of rapid B-lymphocyte proliferation and differentiation (Janeway et al., Citation2004). Therefore, the germinal center and germinal center B-lymphocyte development represent major responses to exposure to T-lymphocyte-dependent antigen (Vieira and Rajewsky, Citation1990; Takahashi et al., Citation1998). We used PNA as a representative germinal center B-lymphocyte surface antigen because all germinal center B-lymphocytes bind PNA, beginning at the earliest stages of germinal center formation (Shinall et al., Citation2000). We observed dose-dependent and significant decreases in the PNA+ B-lymphocyte population in each chemical-treated group (). These results support our belief that this short-term exposure protocol could be used to detect immune suppression followed by chemical treatment.
Conclusions
Our protocol detected environmental chemical (i.e. methoxychlor, parathion, and piperonyl butoxide)-induced immunotoxic responses, such as increased apoptosis in lymphocytes in vitro, decreased antigen-specific IgM responses, and decreased IgM- and germinal center-positive B-lymphocyte counts. Additional studies to confirm these results should be expanded to include other parallel changes in cellular function that can occur in response to chemical exposure, as well as immunologic or histologic markers. Our ongoing studies continue to focus on the detection of weak immunotoxic reactions using this short-term exposure protocol. However, our results and protocols have yet to be formally validated, which is a pre-requisite for inclusion in guidelines endorsed by regulatory authorities. Validation experiments therefore represent the next important task for us to undertake.
Acknowledgments
The authors wish to thank Dr Y. Shutoh, Dr H. Fujie, Dr A. Motomura, and Dr Y. Komatsu of the Institute of Environmental Toxicology (Ibaraki, Japan) for their technical assistance. This work was supported by a research Grant-in-Aid from the Ministry of Health, Labor and Welfare of Japan.
Declaration of interest
The authors report no conflicts of interest. The authors alone are responsible for the content and writing of the paper.
References
- Anderson, S. E., Munson, A. E., Meade, B. J. 2006. Analysis of immunotoxicity by enumeration of antibody-producing B cells. In: Current Protocols in Toxicology. Unit 18.11. (Bus, J. S., Costa, L. G., Hodgson, E., Lawrence, D. A.Reed, D., Eds.). Hoboken, NJ: John Wiley and Sons, pp. 1–18.
- Battaglia, C. L., Gogal, R. M. Jr., Zimmerman, K., Misra, H. P. 2010. Malathion, lindane, and piperonyl butoxide, individually or in combined mixtures, induce immunotoxicity via apoptosis in murine splenocytes in vitro. Int. J. Toxicol. 29:209–220.
- Carson, D. S., Tribble, P. W., Weart, C. W. 1988. Pyrethrins combined with piperonyl butoxide (RID) vs. 1% permethrin (NIX) in the treatment of head lice. Am. J. Dis. Child. 142:768–769.
- Casale, G. P., Cohen, S. D., DiCapua, R. A. 1984. Parathion-induced suppression of humoral immunity in inbred mice. Toxicol. Lett. 23:239–247.
- Committee on Proprietary Medicinal Products. 2000. Note for Guidance on Repeat-Dose Toxicity, CPMP/SWP/1042/99. Available online at: http://www.emea.eu.int.
- Cunningham, A. J. 1965. A method of increased sensitivity for detecting single antibody-forming cells. Nature 207:1106–1107.
- Diel, F., Horr, B., Borck, H., Savtchenko, H., Mitsche, T., Diel, E. 1999. Pyrethroids and piperonyl-butoxide affect human T-lymphocytes in vitro. Toxicol. Lett. 107:65–74.
- Emerson, M. R., Biswas, S., LeVine, S. M. 2001. Cuprizone and piperonyl butoxide, proposed inhibitors of T-cell function, attenuate experimental allergic encephalomyelitis in SJL mice. J. Neuroimmunol. 119:205–213.
- EPA. 1998. United States Environmental Protection Agency. Health Effects Test Guidelines: Immunotoxicity. 1998, OPPTS 870.7800. Available online at:http://www.epa.gov/opptsfrs/publications.
- EPA. 2003. United States Environmental Protection Agency. Office of Research and Development. Human Health Research Strategy (PUB EPA/600/R-02/050). Washington, DC: US EPA, 2003.
- FDA.2002. United States Food and Drug Administration. Guidance for Industry: Immunotoxicology Evaluation of Investigational New Drugs. Available online at:http://www.fda.gov/cder/guidance.
- Fukuyama, T., Kosaka, T., Tajima, Y., Hayashi, K., Shutoh, Y., Harada, T. 2011a. Detection of thymocytes apoptosis in mice induced by organochlorine pesticides methoxychlor. Immunopharmacol. Immunotoxicol. 33:193–200.
- Fukuyama, T., Tajima, Y., Ueda, H., Hayashi, K., Kosaka, T. 2011b. Prior exposure to immunosuppressive organophosphorus or organochlorine compounds aggravates the TH1- and TH2-type allergy caused by topical sensitization to 2,4-dinitrochlorobenzene and trimel-litic anhydride. J. Immunotoxicol. 8:170–182.
- Fukuyama, T., Tajima, Y., Ueda, H., Hayashi, K., Shutoh, Y., Harada, T., Kosaka, T. 2010. Apoptosis in immunocytes induced by several types of pesticides. J. Immunotoxicol. 7:39–56.
- Gurtu, V., Kain, S. R., Zhang, G. 1997. Fluorometric and colorimetric detection of caspase activity associated with apoptosis. Anal. Biochem. 251:98–102.
- Herzyk, D. J., Holsapple, M. 2007. Immunotoxicity evaluation by immune function tests: Focus on the T-dependent antibody response (TDAR). J. Immunotoxicol. 4:143–147.
- ICH. 2006. International Conference on harmonization of technical requirements for registration of pharmaceuticals for human use. ICH Harmonised Tripartite Guideline Immunotoxicity Studies For Human Pharmaceuticals S8. Switzerland. ICH.
- Janeway, C. A., Travers, P., Walport, M., Shlomchik, M. J. (Eds.) 2004. Immunobiology, 6th Edition. New York: Garland Science.
- Jerne, N. K., Nordin, A. A. 1963. Plaque formation in agar by single antibody-producing cells. Science 140:405.
- Kamath, A. B., Xu, H., Nagarkatti, P. S., Nagarkatti, M. 1997. Evidence for the induction of apoptosis in thymocytes by 2,3,7,8-tetrachlorodibenzo-p-dioxin in vivo. Toxicol. Appl. Pharmacol. 142:367–377.
- Ladics, G. S., Smith, C., Elliott, G. S., Slone, T. W., Loveless, S. E. 1998. Further evaluation of incorporation of an immunotoxicological functional assay for assessing humoral immunity for hazard identification purposes in rats in a standard toxicology study. Toxicology 126:137–152.
- Lowry, O. H., Rosebrough, N. J., Farr, A. L., Randall, R. J. 1951. Protein measurement with the Folin phenol reagent. J. Biol. Chem. 193:265–275.
- Mitsumori, K., Takegawa, K., Shimo, T., Onodera, H., Yasuhara, K., Takahashi, M. 1996. Morphometric and immunohistochemical studies on atrophic changes in lymphohematopoietic organs of rats treated with piperonyl butoxide or subjected to dietary restriction. Arch. Toxicol. 70:809–814.
- Morris, R. G., Hargreaves, A. D., Duvall, E., Wyllie, A. H. 1984. Hormone-induced cell death. 2. Surface changes in thymocytes undergoing apoptosis. Am. J. Pathol. 115:426–436.
- Pryputniewicz, S. J., Nagarkatti, M., Nagarkatti, P. S. 1998. Differential induction of apoptosis in activated and resting T-cells by 2,3,7,8-tetrachlorodibenzo-p-dioxin (TCDD) and its repercussion on T-cell responsiveness. Toxicology 129:211–226.
- Registry of Toxic Effects of Chemical Substances NIOSH CD-ROM (2003).
- Savill, J., Haslett, C. 1995. Granulocyte clearance by apoptosis in the resolution of inflamemation. Semin. Cell Biol. 6:385–933.
- Shinall, S. M., Gonzalez-Fernandez, M., Noelle, R. J., Waldschmidt, T. J. 2000. Identification of murine germinal center B-cell subsets defined by the expression of surface isotypes and differentiation antigens. J. Immunol. 164:5729–5738.
- Takahashi, Y., Dutta, P. R., Cerasoli, D. M., Kelsoe, G. 1998. In situ studies of the primary immune response to (4-hydroxy-3-nitrophenyl)acetyl. V. Affinity maturation develops in two stages of clonal selection. J. Exp. Med. 187:885–895.
- Takeuchi, Y., Kosaka, T., Hayashi, K., Ishimine, S., Ohtsuka, R., Kuwahara, M., Yoshida, T., Takahashi, N., Takeda, M., Maita, K., Harada, T. 2004. Alterations in the developing immune system of the rat after perinatal exposure to methoxychlor. J. Toxicol. Pathol. 17:165–170.
- Takeuchi, Y., Kosaka, T., Hayashi, K., Takeda, M., Yoshida, T., Fujisawa, H., Teramoto, S., Maita, K., Harada, T. 2002. Thymic atrophy induced by methoxychlor in rat pups. Toxicol. Lett. 135:199–207.
- Temple, L., Kawabata, T. T., Munson, A. E., White, K. L. Jr. 1993. Comparison of ELISA and plaque-forming cell assays for measuring the humoral immune response to SRBC in rats and mice treated with benzo[a]pyrene or cyclophosphamide. Fundam. Appl. Toxicol. 21:412–419.
- Vermes, I., Haanen, C., Steffens-Nakken, H., Reutelingsperger, C. 1995. A novel assay for apoptosis. Flow cytometric detection of phosphatidylserine expression on early apoptotic cells using fluorescein-labeled Annexin V. J. Immunol. Meth. 184:39–51.
- Vieira, P., Rajewsky, K. 1990. Persistence of memory B-cells in mice deprived of T cell help. Int. Immunol. 2:487–494.
- White, K. L., Musgrove, D. L., Brown, R. D. 2010. The sheep erythrocyte T-dependent antibody response (TDAR). Methods Mol. Biol. 598:173–184.
- Zhang, N., Hartig, H., Dzhagalov, I., Draper, D., He, Y. W. 2005. The role of apoptosis in the development and function of T-lymphocytes. Cell Res. 15:749–769.