Abstract
We investigated the pre-clinical utility of carbon monoxide form of PEGylated hemoglobin (PEG-Hb also named SANGUINATE™) in myocardial infarction (MI) and in particular the response of diabetic tissues to superimposed ischemia/reperfusion injury. SANGUINATE™ was evaluated in diabetic and normal mice subjected to 30 min of coronary artery ligation followed by either 48 h or 28 days of reperfusion. Our results demonstrate that SANGUINATE™ was effective in reducing infarct size when administered either prior to left anterior descending coronary artery (LAD) occlusion or during reperfusion. This finding is an important step in exploring the efficacy of a pharmacoinvasive strategy using SANGUINATE™ in patients with acute coronary syndromes.
Introduction
Hemoglobin-based oxygen carriers (HBOCs) have generated considerable interest as a viable blood substitute to alleviate shortage of blood supplies due to various reasons, including blood supplies infected with HIV and other blood-borne infectious agents. Some of these HBOCs have been approved for the treatment of acute surgical anemia in South Africa. Improvement in key aspects of stored blood safety (Dunne et al. Citation2002, Dunne et al. Citation2004), including long-term shelf life and elimination of the need to cross-match blood type, enabled applications of these HBOCs in military casualties, pre-hospital civilian hemorrhagic trauma, and other indications in which blood is not an option or immediately available. In recent years, HBOCs have been tested for their ability to rescue ischemic tissues after cardiac and vascular surgery.
A novel HBOC, that is, a carbon monoxide form of PEGylated bovine hemoglobin (PEG-COOH), termed SANGUINATE™, developed by Prolong Pharmaceuticals, has been shown to be effective in a rat model of stroke. Compared to vehicle-treated animals, rats treated with SANGUINATE™ displayed reduced infarct volume, reduced neurological deficits, and significantly improved blood flow as assessed by Laser Doppler imaging (Klaus et al. Citation2010). Transfusion of cell-free Hb into the plasma affords the opportunity to promote oxygenation in ischemic tissue. Historically, hypervolemic exchange transfusion of αα-cross-linked tetrameric Hb soon after the onset of experimental stroke was reported to reduce infarct volume in rats (Cole et al. Citation1997), but a clinical trial with this compound was not effective possibly because the time to initial treatment was as long as 18 h (Saxena et al. Citation1999). Furthermore, αα-cross-linked tetrameric Hb readily extravasates in the peripheral circulation where it can scavenge nitric oxide (NO) and cause hypertension (Tsai et al. Citation2006). Hypervolemic exchange transfusion of larger polymers of Hb that extravasate much less and do not impair endothelium-dependent dilation or cause hypertension (Matheson et al. Citation2002) has also been found to reduce infarct volume from experimental stroke (Bobofchak et al. Citation2003, Nemoto et al. Citation2006, Mito et al. Citation2009). Analogous to polymeric Hb, polyethylene glycol-conjugated Hb (PEG-Hb) has an increased molecular radius and does not readily extravasate or cause hypertension (Conover et al. Citation1996, Conover et al. Citation1997, Tsai et al. Citation2006, Young et al. Citation2007). An additional benefit is that conjugation of the Hb molecule surface with PEG reduces immunogenicity, increases oncotic pressure, and increases plasma viscosity (Nucci et al. Citation1996, Intaglietta Citation1999, Wettstein et al. Citation2003). Maintaining plasma viscosity is thought to be important for maintainivng shear stress-induced production of NO by the endothelium and thereby promoting vasodilation (Tsai et al. Citation2005). Therefore, PEG-Hb may provide several advantages over polymeric Hb. When stored in the carboxy state (PEG-COHb), this reduces autooxidation, increases the shelf life, and takes advantage of the pharmacological properties of CO. In addition to the efficacy of SANGUINATE™ as shown in multiple models, including in hypovolemic shock models in rats and dogs as well as in rat stroke, this agent has demonstrated no overt toxicity, no pathology, and no major adverse effects on clinical chemistry or renal function in extensive studies performed on rats, rabbits, and dogs. Furthermore, there was no evidence of immunogenicity after 12 weeks of repeated administration. The latter is a highly relevant consideration for diabetes therapies, as long-term administration may be envisioned in specific clinical settings such as in the heart and peripheral vascular system in which chronic low-level ischemia is pervasive.
Basal diabetic vasculopathy underlies augmented responses to superimposed ischemia/reperfusion injury (van den Oever et al. Citation2010). Diabetic blood vessels display increased oxidative stress, due to both increased production of reactive oxygen species and suppressed levels of antioxidant enzymes (Francia et al. Citation2009, Capellini et al. Citation2010, Cheng et al. Citation2011). We investigated the pre-clinical utility of SANGUINATE™ in myocardial infarction (MI) and, in particular, the response of diabetic tissues to superimposed ischemia/reperfusion injury. Results from our studies highlight novel therapeutic opportunities for this agent in MI and, most importantly, its potential to fill a critical gap in therapies for diabetic heart disease.
Methods
All procedures and treatments in mice were approved by the New York University Medical Center Animal Care and Use Committee. Mice (C57BL6) were rendered type 1 diabetic with streptozotocin (STZ). STZ or citrate buffer (vehicle) is injected at the age of 6 weeks for 5 consecutive days at 55 mg/kg IP; glucose is monitored via tail snip in awake animals (anesthesia increases blood glucose) and measured with a glucometer. Mice with blood levels of 250 mg/dL or higher were used in this study. Mice treated with vehicle were used as normal controls. Mean blood glucose level was 74.8 ± 15.3 mg/dL in non-diabetic control mice, whereas it was 336 ± 42.3 mg/dL in diabetic mice. All mice were on standard chow diet and water was provided ad libitum.
PEG-albumin and PEG-COHb were provided by Prolong Pharmaceuticals (South Plainfield, New Jersey). Surface lysine residues on purified bovine Hb were conjugated with 5000 molecular weight PEG (Nho et al., Citation1992). The PEG-Hb solution was bubbled with CO to convert greater than 80% of the PEG-Hb to PEG-COHb before storage. Solutions containing 4% protein were stored at 2–10°C in sterile blood bags.
Experimental design
Protocols for mouse MI
In vivo LAD ligation/re-occlusion was performed in a single survival surgery as published earlier (Aleshin et al. Citation2008). Mice were anesthetized using ketamine (95 mg/kg) and xylazine (5 mg/kg), and the LAD branch of the left coronary artery was tied with a suture (7–0 silk); effectiveness of this strategy was monitored by observation of myocardial blanching. Ischemia was induced for 30 min; following that time, the ligature was released. The chest cavity was closed, and mice weaned from the respirator. Animals were warmed and given intraperitoneal (i.p.) warmed 0.9% physiologic saline. Post-operative pain control was achieved by giving buprenorphine (0.05–0.1 mg/kg) subcutaneously 30 min before the completion and 24 h after surgery. Mice were sacrificed according to the time points indicated in . Infarct area was measured using TTC staining, and area at risk was measured using Evans blue. The heart is perfused with Evans blue in-situ and then removed, and coronal sections of the entire ventricular tissue are cut at approximately 1-mm intervals. The sections were photographed with a device providing scale, then embedded in the TTC solution at 37°C, and thereafter fixed for up to 2 h in 10% phosphate-buffered formalin. The sections were then re-photographed to determine the area of TTC staining (again with a scale). The first set of photographs provides the area of perfusion or non-perfusion (i.e., Evans blue negative); the second set shows the area of necrosis (i.e., TTC negative). Since all slices are approximately of the same thickness, we calculated the relative amount of necrosis in the non-perfused area as sum of ratios of the necrotic area divided by the non-perfused area of each slice (Aleshin et al. Citation2008).
Figure 1. Scheme depicting studies conducted in SANGUINATE™-treated and SANGUINATE™-untreated mice. (a) SANGUINATE™ was administered either prior to 30 min of LAD ligation and during 48 h of reperfusion or only during 48 h of reperfusion. (b) In the second set of experiments, mice were administered SANGUINATE™ only during 28 days of reperfusion after LAD ligation. Since PBS was used as a vehicle, in both (a) and (b), mice treated with PBS were used as the control group. (n = 12 in each group).
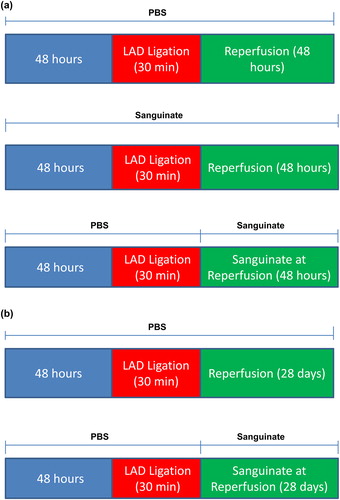
All mice underwent LAD ligation followed by reperfusion. Cardiac function was assessed by echocardiography prior to LAD ligation and at the end of reperfusion period. Plasma samples and hearts were collected and frozen at − 80°C for biochemical assays. The following studies shown in Figures 1a and 1b were conducted in SANGUINATE™-treated and SANGUINATE™-untreated mice.
Study I: SANGUINATE™ treatment prior to ischemia and during 48 h of recovery after reperfusion
Normal mice (NDM-PBS) (n = 12) received i.p. injections of an equivalent volume of vehicle used for SANGUINATE™ (20 ml/kg body weight in PBS buffer) prior to LAD ligation and during 48 h of recovery after reperfusion. All cohorts received a single dose of SANGUINATE™ or PBS administered daily for 2 days prior to LAD ligation and for 2 days during reperfusion.
Normal mice (NDM-Sang) (n = 12) received i.p. injections of SANGUINATE™ prior to LAD ligation and during 48 h of recovery after reperfusion.
Diabetic mice (DM-PBS) (n = 12) received i.p. injections of an equivalent volume of vehicle used for SANGUINATE™ (in PBS buffer) prior to LAD ligation and during 48 h of recovery after reperfusion.
Diabetic mice (DM-Sang) (n = 12) received i.p. injections of SANGUINATE™ prior to LAD ligation and during 48 h of recovery after reperfusion.
Study II: SANGUINATE™ treatment only during 48 h of recovery after initiating reperfusion
NDM-Sang (n = 12) and DM-Sang (n = 12) mice were treated with SANGUINATE™ only during the reperfusion period. The mice were treated with a single dose of SANGUINATE™ (20 ml/kg body weight in PBS buffer) at the start of reperfusion, during day 1 and during day 2 of recovery after reperfusion.
Study III: SANGUINATE™ treatment only during 28 days after the start of reperfusion
In these studies, all hearts were subjected to LAD ligation for 30 min followed by recovery for 28 days after reperfusion. The NDM-Sang (n = 12) and DM-Sang (n = 12) mice were treated daily for 28 days with a single dose of SANGUINATE™ (20 ml/kg body weight in PBS buffer), while the NDM-PBS and DM-PBS mice received daily injections of PBS.
Cardiac function was assessed using echocardiography as published earlier (Aleshin et al. Citation2008). Baseline echocardiograms were performed the day prior to surgery and then at the end of recovery after reperfusion for each study as described above. We used a high-resolution Vevo 2100 (Visualsonics) cardiography system for mice. Briefly, mice were anesthetized lightly with isoflurane in 100% oxygen and in vivo transthoracic echocardiography of the left ventricle using a 30-Mhz RMV scanhead interfaced with a Vevo 2100. This provides high-resolution, two-dimensional electrocardiogram-based kilohertz visualization. B mode images were acquired at a rate of 1,000 frames/s over 7 min. These images were used to measure left ventricular end-diastolic diameter (LVEDD) and left ventricular end-systolic diameter (LVES). The B mode images are used to calculate the left ventricular ejection fraction. Fractional shortening (FS) and fractional area change (FAC) were calculated from the aforementioned parameters as published earlier (Aleshin et al. Citation2008).
Plasma levels of troponin were measured at 48 h or 28 days after surgery as measures of cardiac necrosis and damage (Aleshin et al. Citation2008). ATP and malondialdehyde (MDA) were measured in tissue extracts as published by us earlier (Hwang et al. Citation2004, Ananthakrishnan et al. Citation2009).
Measurements were compared among the groups using ANOVA, and post hoc comparisons were performed using Tukey's or Dunnett's procedure. Data are presented as mean ± SD.
Results
Effect of pre- and post-ischemic treatment of SANGUINATE™ during ischemia/reperfusion
To determine whether SANGUINATE™ protects hearts subjected to ischemia/reperfusion, we subjected normal and diabetic mice to LAD ligation for 30 min followed by reperfusion for 48 h. Mice were treated for 2 days with SANGUINATE™ prior to LAD ligation and then followed by continued treatment during reperfusion. We measured, using TTC staining, area at risk and infarct area of all hearts at the end of the 48 h of reperfusion. Cardiac function in all hearts was measured prior to ligation and after 48 h of reperfusion. SANGUINATE™ treatment reduced the infarct area in both diabetic and normal hearts (). Total area at risk was similar in all hearts. Release of cardiac troponin-I, a marker of necrosis, in the plasma was attenuated by SANGUINATE™ treatment in the diabetic mice, but not in the normal mice (). Cardiac function was similar in SANGUINATE™-treated and SANGUINATE™-untreated hearts (). These data indicate that SANGUINATE™ treatment attenuated infarct size in both diabetic and normal mice.
Figure 2. Impact of myocardial infarction (MI) in SANGUINATE™-treated diabetic and normal mice prior to LAD occlusion and during 48 h of reperfusion. Hearts retrieved after LAD ligation followed by 48 h of reperfusion and subjected to TTC staining (a) for the analysis of total area at risk (b) and infarcted area (c) in the following groups of mice: normal PBS-treated (NDM-PBS), SANGUINATE™-treated NDM (NDM-Sang), diabetic PBS (DM-PBS), and SANGUINATE™-treated DM (DM-Sang). (d) Troponin-C release in plasma, marker of ischemic injury, was also determined in all the groups of mice during the 48 h of reperfusion. NDM-Sang and DM-Sang mice received i.p. injections of SANGUINATE™ prior to LAD ligation and during 48 h of reperfusion. NDM-PBS and DM-PBS mice received i.p. injections of an equivalent volume of PBS vehicle prior to LAD ligation and during 48 h of reperfusion. (n = 12 in each group).
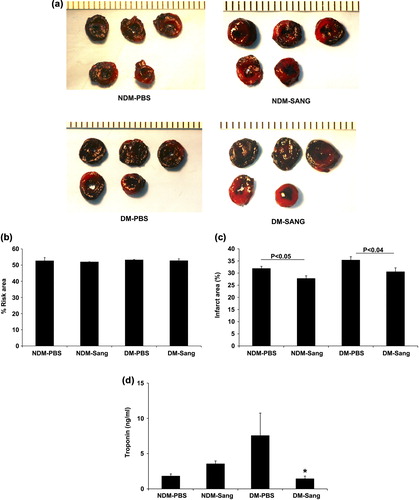
Table I. Cardiac Functional determined using echocardiography.
Effect of SANGUINATE™ when treated during recovery after initiation of reperfusion
Since patients arrive in the hospital after experiencing a MI, we investigated whether SANGUINATE™ treatment during the recovery phase would protect hearts from ischemia/reperfusion injury. In this study, we subjected diabetic and normal mice to LAD ligation for 30 min followed by recovery for 48 h after initiation of reperfusion in the presence of SANGUINATE™. The infarct area was significantly reduced in both the diabetic and normal hearts that were treated with SANGUINATE™ only during recovery phase (). Total area at risk was similar in all hearts. Release of cardiac troponin-I, a marker of necrosis, in the plasma was attenuated by SANGUINATE™ treatment in the diabetic mice, but not in the normal mice (). Cardiac function was similar in SANGUINATE™-treated and SANGUINATE™-untreated hearts (). These data indicate that SANGUINATE™ treatment reduces the infarct area in both diabetic and normal hearts when administered during recovery phase after initiating reperfusion.
Figure 3. Impact of MI in SANGUINATE™-treated diabetic and normal mice only during 48 h of reperfusion. Hearts retrieved after LAD ligation followed by 48 h of reperfusion and subjected to TTC staining (a) for the analysis of total area at risk (b) and infarcted area (c) in the myocardium were determined along with troponin-C (d) release in plasma during reperfusion. NDM-Sang and DM-Sang mice received i.p. injections of SANGUINATE™ only during 48 h of reperfusion. NDM-PBS and DM-PBS mice received i.p. injections of an equivalent volume of PBS vehicle during 48 h of reperfusion. (n = 12 in each group).
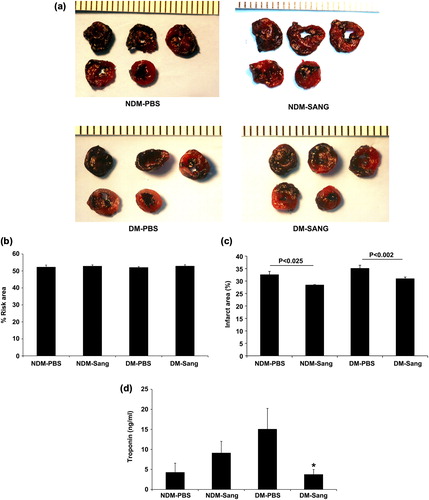
Effect of SANGUINATE™ during 28 days of recovery
Scarring of the tissue after MI is observed in patients who undergo revascularization procedures. To determine whether SANGUINATE™ affords long-term protection in the myocardium after MI, we subjected diabetic and normal mice to LAD ligation for 30 min followed by reperfusion recovery for 28 days with SANGUINATE™. Here, hearts were treated daily with single injection of SANGUINATE™ for 28 days. Significant reductions in the infarct area were observed in both the diabetic and normal hearts at 28 days in the SANGUINATE™ treatment groups (). Cardiac function was similar in SANGUINATE™-treated and SANGUINATE™-untreated hearts after 28 days of recovery reperfusion (). We did not observe any differences in troponin-C release in the saline-treated and SANGUINATE™-treated hearts at 28-day time point (data not shown). These data indicate that SANGUINATE™ affords long-term protection in diabetic and normal hearts when administered during recovery phase.
Figure 4. Impact of MI in SANGUINATE™-treated diabetic and normal mice only during 28 days of reperfusion. Hearts retrieved after LAD ligation followed by 28 days of reperfusion and subjected to TTC staining (a) for the analysis of total area at risk (b) and infarcted area (c) in the myocardium were determined. NDM-Sang and DM-Sang mice received i.p. injections of SANGUINATE™ only during 28 days of reperfusion. NDM-PBS and DM-PBS mice received i.p. injections of an equivalent volume of PBS vehicle during 48 h of reperfusion (n = 12 in each group).
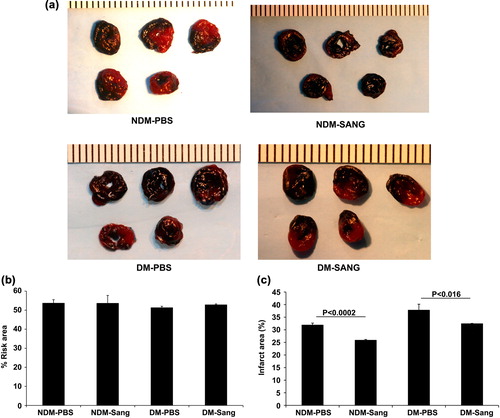
Effect of SANGUINATE™ on markers of oxidative stress and energy metabolism
To determine whether cardioprotection afforded by SANGUINATE™ is linked to reductions in oxidative stress, we measured the changes in malondialdehyde (MDA) levels in hearts at the end of 48 h of recovery. MDA levels were measured in hearts obtained at the end of the recovery period in Studies I, II, and III. In the hearts treated with PBS, MDA levels were significantly higher in DM hearts than in normal hearts (). SANGUINATE™ treatment significantly reduced MDA levels in DM hearts from Study I and Study II (). In the hearts from Study III (28 days of recovery), MDA levels were similar in the SANGUINATE™-treated and PBS-treated groups (data not shown). These data indicate that SANGUINATE™ treatment reduces oxidative stress during the early phase of reperfusion.
Figure 5. Impact of SANGUINATE™ on oxidative stress changes in diabetic and normal mice after LAD ligation followed by reperfusion. Oxidative stress marker, malondialdehyde (MDA), was measured in heart lysates from NDM-PBS, NDM-Sang, DM-PBS, and DM-Sang in Study I (a) and Study II. Hearts in Studies I and II were harvested at the end of recovery period of 48 h. In Study I, mice were treated with SANGUINATE™ throughout LAD ligation and during 48 h of reperfusion (a), whereas in Study II the mice were treated with SANGUINATE™ only during 48 h of recovery after initiating reperfusion (b). More details on the protocols for Study I and Study II are described in the methods section. The MDA levels were determined using commercially available kits from Oxis. Data represent means ± SD; n = 4–6 per group.
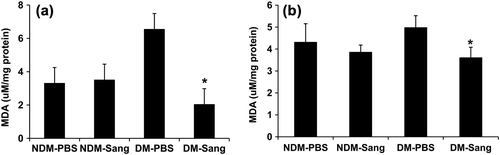
To determine whether cardioprotection by SANGUINATE™ is due to improved energy metabolism, we measured ATP levels in all hearts prior to LAD ligation and at the end of reperfusion. SANGUINATE™-treated and SANGUINATE™-untreated DM and NDM hearts had similar levels of ATP (15 to 21 μmoles/gram dry weight) prior to LAD ligation. ATP recovery during reperfusion (48 h and 28 days) was similar in all groups of hearts. These data indicate that the protection afforded by SANGUINATE™ is independent of improvement in energy metabolism.
Discussion
In the present study, we show for the first time that SANGUINATE™ treatment during the reperfusion period reduces infarct size and protects both diabetic and normal hearts from injury due to MI. Basal diabetic vasculopathy underlies augmented responses to superimposed ischemia/reperfusion injury (van den Oever et al. Citation2010). It is well known that diabetic blood vessels and tissue display increased oxidative stress, due to combined effects of increased generation of reactive oxygen species and reduced activity of antioxidant enzymes (Francia et al. Citation2009, Capellini et al. Citation2010, Cheng et al. Citation2011). We show that SANGUINATE™ affords cardioprotection under conditions of such high oxidative stress.
MI results in death of ischemic cardiac tissue followed by an inflammatory response and replacement of contractile tissue with a fibrotic scar. The protection afforded by SANGUINATE™ was independent of functional improvements in these MI studies. The reduction of infarct size in SANGUINATE™-treated mice could, in theory, result from a reduced propensity to generate an infarct or an increased capacity for healing and regeneration of healthy cardiac tissue. Our data on the SANGUINATE™-mediated reduction of infarct size without any functional improvement are similar to the recent findings with genetic myocardin-related transcription factor-A (MRTF-A) (Small et al. Citation2010). In those studies, genetic deletion of MRTFA reduced infarct size without any improvement in cardiac function. Furthermore, it was shown that the protective effect of MRTF-A deletion was associated with a reduction in the expression of fibrosis-associated genes, including collagen 1a2, a direct transcriptional target of SRF/MRTF-A.
Part of the cardiac protection from ischemia seen with SANGUINATE™ might be attributed to the release of CO. Combined pre-treatment and post-treatment with human PEG-COHb has been shown to protect the heart from coronary artery occlusion in rats (Vandegriff et al. Citation2008). In these rat studies, investigators observed cardioprotection with human PEG-COHb and not with an equivalent administration of oxy-form of PEG-Hb (Vandegriff et al. Citation2008). The vasoactive actions of CO have been recognized for some time (Motterlini et al. Citation2002, Sammut et al. Citation1998) and could contribute to improved perfusion. In addition, the creation of a ‘preconditioned” phenotype in myocardium by CO (Stein et al. Citation2005) evokes a number of potential cardioprotective actions, including activation of effector mechanisms such as K+ channel opening (Clark et al. Citation2003, Dong et al. Citation2007), stabilization of HIF-1α (Ockaili et al. Citation2005, Natarajan et al. Citation2006, Chin et al. Citation2007), and induction of cardioprotective proteins (Yellon and Downey Citation2003, Dawn and Bolli Citation2005, Bilban et al. Citation2008). The effects of SANGUINATE™, with regard to these potential mechanisms, are only speculative at this time.
Our results may have importance for clinical practice since SANGUINATE™ may prove effective in reducing infarct size when administered during reperfusion. Earlier studies have indicated that HBOCs may have long-term detrimental effects, including an increase in the risk of MI in patients undergoing major surgery or treatment of hemorrhagic shock (Cabrales et al. Citation2007, Natanson et al. Citation2008, Silverman and Weiskopf Citation2009). In the present study, using repeated long-term i.p SANGUINATE™ treatment did not reveal any adverse myocardial effects. Rather, our data demonstrated remarkable reduction in infarct area by SANGUINATE™ in the 28-day treatment study.
In summary, the present work provides a proof of principle that SANGUINATE™ has potential as an adjunct therapy in the treatment of diabetic and normal patients with evolving MI. Further work is required to determine the therapeutic time window of opportunity for administration in both type 1 and type 2 diabetics with evolving MI, the optimal infusion protocol and dose, long-term systemic and cardiac effects, and efficacy in a model of ischemic cardiomyopathy.
Declaration of interest
Radha Ananthakrishnan, Qing Li, Karen M. O’Shea, Nosirudeen Quadri, Lingjie Wang, Ann Marie Schmidt, and Ravichandran Ramasamy have no financial interest in Prolong Pharmaceuticals and report no conflict of interest. Abraham Abuchowski is CEO of Prolong Pharmaceuticals, which holds the IP for bovine PEG-Hb.
This work was supported by funds from Prolong Pharmaceuticals, Plainfield, NJ.
References
- Aleshin A, Ananthakrishnan R, Li Q, Rosario R, Lu Y, Qu W, et al. 2008. RAGE modulates myocardial injury consequent to LAD infarction via impact on JNK and STAT signaling in a murine model. Am J Physiol Heart Circ Physiol. 294:H1823–832.
- Ananthakrishnan R, Kaneko M, Hwang YC, Quadri N, Gomez T, Li Q, et al. 2009. Aldose reductase mediates myocardial ischemia- reperfusion injury in part by opening mitochondrial permeability transition pore. Am J Physiol Heart Circ Physiol. 296:H333–341.
- Bilban M, Haschemi A, Wegiel B, Chin BY, Wagner O, Otterbein LE. 2008. Heme oxygenase and carbon monoxide initiate homeostatic signaling. J Mol Med (Berl). 86:267–279.
- Bobofchak KM, Mito T, Texel SJ, Bellelli A, Nemoto M, Traystman RJ, et al. 2003. A recombinant polymeric hemoglobin with conformational, functional, and physiological characteristics of an in vivo O2 transporter. Am J Physiol Heart Circ Physiol. 285:H549–561.
- Cabrales P, Tsai AG, Intaglietta M. 2007. Deferoxamine lowers tissue damage after 80% exchange transfusion with polymerized hemoglobin. Antioxid Redox Signal. 9:375–384.
- Capellini VK, Celotto AC, Baldo CF, Olivon VC, Viaro F, Rodrigues AJ, Evora PR. 2010. Diabetes and vascular disease: basic concepts of nitric oxide physiology, endothelial dysfunction, oxidative stress and therapeutic possibilities. Curr Vasc Pharmacol. 8:526–544.
- Cheng X, Siow RC, Mann GE. 2011. Impaired redox signaling and antioxidant gene expression in endothelial cells in diabetes: a role for mitochondria and the nuclear factor-E2-related factor 2-Kelch-like ECH-associated protein 1 defense pathway. Antioxid Redox Signal. 14:469–487.
- Chin BY, Jiang G, Wegiel B, Wang HJ, Macdonald T, Zhang XC, et al. 2007. Hypoxia-inducible factor 1alpha stabilization by carbon monoxide results in cytoprotective preconditioning. Proc Natl Acad Sci USA. 104:5109–5114.
- Clark JE, Naughton P, Shurey S, Green CJ, Johnson TR, Mann BE, et al. 2003. Cardioprotective actions by a water-soluble carbon monoxide-releasing molecule. Circ Res. 93:e2–8.
- Cole DJ, Drummond JC, Patel PM, Reynolds LR. 1997. Hypervolemic-hemodilution during cerebral ischemia in rats: effect of diaspirin cross-linked hemoglobin (DCLHb) on neurologic outcome and infarct volume. J Neurosurg Anesthesiol. 9:44–50.
- Conover CD, Lejeune L, Shum K, Gilbert C, Shorr RG. 1997. Physiological effect of polyethylene glycol conjugation on stroma-free bovine hemoglobin in the conscious dog after partial exchange transfusion. Artif Organs. 21:369–378.
- Conover CD, Malatesta P, Lejeune L, Chang CL, Shorr RG. 1996. The effects of hemodilution with polyethylene glycol bovine hemoglobin (PEG-Hb) in a conscious porcine model. J Investig Med. 44:238–246.
- Dawn B, Bolli R. 2005. HO-1 induction by HIF-1: a new mechanism for delayed cardioprotection?Am J Physiol Heart Circ Physiol. 289:H522–524.
- Dong DL, Zhang Y, Lin DH, Chen J, Patschan S, Goligorsky MS, et al. 2007. Carbon monoxide stimulates the Ca2(+)-activated big conductance k channels in cultured human endothelial cells. Hypertension. 50:643–651.
- Dunne JR, Malone D, Tracy JK, Gannon C, Napolitano LM. 2002. Perioperative anemia: an independent risk factor for infection, mortality, and resource utilization in surgery. J Surg Res. 102:237–244.
- Dunne JR, Malone DL, Tracy JK, Napolitano LM. 2004. Allogenic blood transfusion in the first 24 hours after trauma is associated with increased systemic inflammatory response syndrome (SIRS) and death. Surg Infect (Larchmt). 5:395–404.
- Francia P, Cosentino F, Schiavoni M, Huang Y, Perna E, Camici GG, et al. 2009. p66(Shc) protein, oxidative stress, and cardiovascular complications of diabetes: the missing link. J Mol Med (Berl). 87:885–891.
- Hwang YC, Kaneko M, Bakr S, Liao H, Lu Y, Lewis ER, et al. 2004. Central role for aldose reductase pathway in myocardial ischemic injury. FASEB J. 18:1192–1199.
- Intaglietta M. 1999. Microcirculatory basis for the design of artificial blood. Microcirculation. 6:247–258.
- Klaus JA, Kibler KK, Abuchowski A, Koehler RC. 2010. Early treatment of transient focal cerebral ischemia with bovine PEGylated carboxy hemoglobin transfusion. Artif Cells Blood Substit Immobil Biotechnol. 38:223–229.
- Matheson B, Kwansa HE, Bucci E, Rebel A, Koehler RC. 2002. Vascular response to infusions of a nonextravasating hemoglobin polymer. J Appl Physiol. 93:1479–1486.
- Mito T, Nemoto M, Kwansa H, Sampei K, Habeeb M, Murphy SJ, et al. 2009. Decreased damage from transient focal cerebral ischemia by transfusion of zero-link hemoglobin polymers in mouse. Stroke. 40:278–284.
- Motterlini R, Clark JE, Foresti R, Sarathchandra P, Mann BE, Green CJ. 2002. Carbon monoxide-releasing molecules: characterization of biochemical and vascular activities. Circ Res. 90:E17–24.
- Natanson C, Kern SJ, Lurie P, Banks SM, Wolfe SM. 2008. Cell-free hemoglobin-based blood substitutes and risk of myocardial infarction and death: a meta-analysis. JAMA. 299:2304–2312.
- Natarajan R, Salloum FN, Fisher BJ, Kukreja RC, Fowler AA III. 2006. Hypoxia inducible factor-1 activation by prolyl 4-hydroxylase-2 gene silencing attenuates myocardial ischemia reperfusion injury. Circ Res. 98:133–140.
- Nemoto M, Mito T, Brinigar WS, Fronticelli C, Koehler RC. 2006. Salvage of focal cerebral ischemic damage by transfusion of high O2-affinity recombinant hemoglobin polymers in mouse. J Appl Physiol. 100:1688–1691.
- Nho K, Glower D, Bredehoeft S, Shankar H, Shorr R, Abuchowski A. 1992. PEG-bovine hemoglobin: safety in a canine dehydrated hypovolemic-hemorrhagic shock model. Biomater Artif Cells Immobilization Biotechnol. 20:511–524.
- Nucci ML, Shorr RGL, Abuchowski A. 1996. PEG-hemoglobin oxygen carrying blood substitute. Drugs Future. 21:29–32.
- Ockaili R, Natarajan R, Salloum F, Fisher BJ, Jones D, Fowler AA III, Kukreja RC. 2005. HIF-1 activation attenuates postischemic myocardial injury: role for heme oxygenase-1 in modulating microvascular chemokine generation. Am J Physiol Heart Circ |Physiol. 289:H542–548.
- Sammut IA, Foresti R, Clark JE, Exon DJ, Vesely MJ, Sarathchandra P, et al. 1998. Carbon monoxide is a major contributor to the regulation of vascular tone in aortas expressing high levels of haeme oxygenase-1. Br J Pharmacol. 125:1437–1444.
- Saxena R, Wijnhoud AD, Carton H, Hacke W, Kaste M, Przybelski RJ, et al. 1999. Controlled safety study of a hemoglobin-based oxygen carrier, DCLHb, in acute ischemic stroke. Stroke. 30:993–996.
- Silverman TA, Weiskopf RB. 2009. Hemoglobin-based oxygen carriers: current status and future directions. Transfusion. 49:2495–2515.
- Small EM, Thatcher JE, Sutherland LB, Kinoshita H, Gerard RD, Richardson JA, et al. 2010. Myocardin-related transcription factor-a controls myofibroblast activation and fibrosis in response to myocardial infarction. Circ Res. 107:294–304.
- Stein AB, Guo Y, Tan W, Wu WJ, Zhu X, Li Q, et al. 2005. Administration of a CO-releasing molecule induces late preconditioning against myocardial infarction. J Mol Cell Cardiol. 38:127–134.
- Tsai AG, Acero C, Nance PR, Cabrales P, Frangos JA, Buerk DG, Intaglietta M. 2005. Elevated plasma viscosity in extreme hemodilution increases perivascular nitric oxide concentration and microvascular perfusion. Am J Physiol Heart Circ Physiol. 288:H1730–1739.
- Tsai AG, Cabrales P, Manjula BN, Acharya SA, Winslow RM, Intaglietta M. 2006. Dissociation of local nitric oxide concentration and vasoconstriction in the presence of cell-free hemoglobin oxygen carriers. Blood. 108:3603–3610.
- Van den Oever IA, Raterman HG, Nurmohamed MT, Simsek S. 2010. Endothelial dysfunction, inflammation, and apoptosis in diabetes mellitus. Mediators Inflamm. 2010:792393.
- Vandegriff KD, Young MA, Lohman J, Bellelli A, Samaja M, Malavalli A, Winslow RM. 2008. CO-MP4, a polyethylene glycol-conjugated haemoglobin derivative and carbon monoxide carrier that reduces myocardial infarct size in rats. Br J Pharmacol. 154:1649–1661.
- Wettstein R, Tsai AG, Erni D, Winslow RM, Intaglietta M. 2003. Resuscitation with polyethylene glycol-modified human hemoglobin improves microcirculatory blood flow and tissue oxygenation after hemorrhagic shock in awake hamsters. Crit Care Med. 31:1824–1830.
- Yellon DM, Downey JM. 2003. Preconditioning the myocardium: from cellular physiology to clinical cardiology. Physiol Rev. 83: 1113–1151.
- Young MA, Malavalli A, Winslow N, Vandegriff KD, Winslow RM. 2007. Toxicity and hemodynamic effects after single dose administration of MalPEG-hemoglobin (MP4) in rhesus monkeys. Transl Res. 149:333–342.