Abstract
The lymphatic system works in close collaboration with the cardiovascular system to preserve fluid balance throughout the body and is essential for the trafficking of antigen-presenting cells and lymphocytes to lymphoid organs. Recent findings have associated lymphatic dysfunction with the pathogenesis of cardiovascular-related diseases such as atherosclerosis, inflammation and obesity. Whether lymphatic dysfunction is a cause or a consequence of these diseases, as well as how, is under intensive investigation. Extracellular vesicles (EVs) are submicron vesicles released by diverse cell types upon activation or apoptosis and are considered important biomarkers for several inflammatory diseases. Thus, it is critical to characterize the presence of EVs in various biological tissues and fluids to delineate their origins and, subsequently, their functions. In the past few years, new techniques allowing the quantitative and qualitative analysis of EVs have emerged, thus facilitating the onset of studies bridging these vesicles to the lymphatic system. Using several state-of-the-art approaches, this article reports the presence of diverse EVs inclusively derived from red blood cells and platelets in lymph of healthy animals. Our results suggest that lymph from atherosclerotic mice displays a higher concentration of EVs, bringing forward the concept that EVs contained in lymph could either be a biomarker for lymphatic dysfunction or, conversely, for inflammatory disease progression.
The lymphatic system is the principal route of transport from tissues for antigen and immune cells (Citation1). It is necessary to maintain fluid balance, fight infection, absorb dietary fat from the intestine and then transport it to the liver. Recently, dysfunction of this ubiquitous network has been linked to the pathogenesis of cardiovascular diseases, like ageing (Citation2), hypercholesterolemia (Citation3–Citation6) and atherosclerosis (Citation7). Our own work (Citation7) and that of others (Citation8, Citation9) maintains that absence or obstruction of lymphatic vessels may accelerate inflammation occurring mostly in the intimal layer of the artery. Whereas inflammatory cell accumulation in peripheral tissues has been suggested to be responsible for the subsequent lymphatic transport impairment in obesity (Citation10), it is still not clear whether lymphatic dysfunction is a cause or a consequence of other pathologies such as atherosclerosis, nor how.
The lymphatic network consists of an open, unidirectional and low-pressure vascular system. Plasma ultrafiltrate in peripheral tissues is first absorbed through absorptive blind-ended initial lymphatic vessels. Lymph subsequently moves into lymphatic collecting vessels, the entities responsible for maintaining lymph flow through the contraction of units called lymphangions. Bileaflet valves are present between the contractile units to prevent backflow (Citation11), and the lymphatic endothelial cells (LEC) feature continuous “zipper-like” cell–cell junctions and a discontinuous smooth muscle cell (SMC) layer. Once they reach the lymph nodes, the afferent collecting vessels become the efferent collecting vessels, ultimately converging into the thoracic duct where lymph collected from all organs drains into the blood circulation at the subclavian veins through the lymphovenous (LV) junction (Citation12). Platelets have a unique role in lymphatic vessel development and maintenance, and they protect both the LV junction and the thoracic duct from backward flow. Furthermore, this platelet-dependent process is required throughout life to prevent retrograde blood flow and to maintain lymphatic function (Citation13).
The composition of lymph is analogous to that of plasma, as it contains white blood cells, notably lymphocytes, but is essentially absent of red blood cells (RBCs), poorer in nutrients and richer in waste. A broad array of cytokines, proteins and growth factors are contained within lymphatic fluid, which play an important role in metabolism and cell proliferation (Citation14). Whereas studies have confirmed the presence of extracellular vesicles (EVs) of diverse origins in circulating blood (Citation15), it is not clear yet whether these EVs can also be found in lymph per se. EVs are derived from cells upon activation and/or apoptosis (Citation15, Citation16) and are considered important biomarkers and key players in several inflammatory settings including atherosclerosis and rheumatic disease (Citation15, Citation17–Citation21). Physiologically, EVs also constitute a heterogeneous population, differing in cellular origin, number, size, antigenic composition and functional properties (Citation22). They comprise exosomes, vesicles stored in multivesicular bodies (50–150 nm), and microvesicles (100–1,000 nm) produced by cytoplasmic-membrane budding and shedding. EVs generated from apoptotic cells are called apoptotic bodies (>1 µm). EVs are suggested to play key roles in intercellular communication by transporting messenger RNA, microRNA and proteins (Citation23); they can interact with neighbouring cells, causing structural and functional changes in tissues like the vascular wall, thereby affecting the endothelium (Citation24) and myeloid cells such as macrophages (Citation25) and neutrophils (Citation26). As one of the major roles of the lymphatic network is to orchestrate the clearance of cells and molecules from peripheral tissues, it is immunologically essential to maintain proper lymphatic vessel integrity throughout life. Recently, through in vivo near-infrared imaging, exosomes have been shown to be rapidly transported within minutes from the periphery to the lymph node by lymphatics (Citation27). Using the most advanced complementary methods to date, we herein sought to qualitatively and quantitatively report the presence of EVs in healthy and atherosclerotic mouse lymph collected in vivo. Our results suggest that EVs derived inclusively, but not exclusively, from RBCs and platelets circulate in the lymph of healthy animals and prevail in atherosclerosis.
Methods
Animals
Adult healthy 8-week old female wild-type (WT) C57BL/6 mice fed on a regular chow diet and age- and sex-matched low-density lipoprotein receptor -/- (LDLR) mice also on a C57BL/6 background were purchased from Jackson Laboratory (Maine (ME), USA). LDLR-/- mice were fed with a high fat diet Harlan Teklad 88137 (Indianapolis (IN), USA) for 8 weeks to ensure the atherosclerotic phenotype. Animals were housed in a pathogen-free environment under a 12-hour light–dark cycle with free access to water and food. All experiments were performed in accordance with the Canadian Council on Animal Care guidelines and approved by the Montreal Heart Institute Animal Care Committee.
Thoracic duct cannulation
Mice were anesthetized with isoflurane (4% for induction, 2–3% for maintenance). The anesthetized animal was positioned on its right side and a cannula was inserted into the thoracic lymph duct above the cisterna chyli between the transverse lumbar artery and the diaphragm. Lymph was collected continuously, on average for 45 minutes to 1 hour, with a tube attached to a syringe coated with EDTA 0.1 M. Collected lymph was centrifuged at 1,200 g for 10 minutes at 4°C to remove most traces of whole cells, while being cautious not to create EVs due to high-speed centrifugation. Following that, 5% sucrose was added to each sample to improve preservation of the sample, which was then placed in a freezer at −80°C for further batch analysis. Although our technique ensures extreme care during collection, the absence of contaminating cells in lymph isolated from the thoracic duct was verified using a PC-based automated cell counter (Cellometer Auto X4, equipped with a 470/535 nm optic module; Nexcelom (Massachusetts (MA), USA)) that allowed bright field and fluorescent cell counting using acridine orange (AO), a nuclear-staining cell-permeable dye that stains all nucleated cells to generate green fluorescence. RBCs were considered as AO− cells larger than 5 µm.
Cryo-transmission electron microscopy of lymph samples
Prior to electron microscopy (EM) analysis, lymph samples were labelled with 10 nm gold particles conjugated with annexin V as follows. An 8-µL lymph aliquot was mixed with 1 µL annexin-5-conjugated gold particles at 1017 particles/L and 1 µL 20 mM Ca2+. After 30 minutes’ incubation at ambient temperature, 4-µL aliquots of lymph samples were deposited on EM grids coated with a perforated carbon film. After draining the excess liquid with a filter paper, grids were quickly plunged into liquid ethane and transferred to cryo-boxes and stored under liquid nitrogen. For cryo-EM observation, grids were mounted onto a Gatan 626 cryoholder and transferred in a Tecnai F20 (FEI Company, Eindhoven, Netherlands) microscope operated at 200 kV. Images were recorded with an USC1000-SSCCD camera (California (CA), United States).
Extracellular vesicle labelling
Lymph samples were rapidly thawed, which is considered optimal to maintain the EV integrity. Lymph (5 µL) containing D-phenylalanyl-L-prolyl-L-arginine chloromethyl ketone (PPACK, at 10 µM), an anticoagulant with no effect on Ca+2 dynamics, was diluted in a total volume of 100 µL filtered (0.2 µM) annexin V buffer 1X (BD Biosciences, San Jose, CA, USA) containing V450 conjugated annexin V (BD Biosciences, 1.5 µL) following the manufacturer's instructions. PPACK was included because addition of calcium was found to trigger the formation of micro-aggregates in some biological fluids, which are confounders in flow cytometry analysis (EB, NT and AB, data not shown). Anti-CD41 antibodies conjugated with allophycocyanin (APC) (Biolegend (California (CA), United States), 5 µg/mL) and APC conjugated anti-Ter-119 (Biolegend, 2.5 µg/mL) were added, in 2 different labelling cocktails, to identify EVs from platelets and RBCs, respectively. EVs were incubated in labelling cocktails for 30 minutes at room temperature, and the presence of esterase in EVs was established by the addition of carboxyfluorescein succinimidyl ester (CFSE, eBioscience California (CA), United States) at 5 µM for the last 15 minutes of incubation. Cocktails were diluted by the addition of 400 µL of filtered (0.2 µM) annexin V buffer 1X prior analyses by flow cytometry. As controls, antibodies conjugated with the same fluorochromes but directed against human antigens were used to position the negative flow cytometry gates, whereas 50 µM EDTA or detergent Triton X-100 (0.05%) were respectively added to cocktails in order to verify the specificity of annexin V staining and the membrane moiety of EVs. Only for the EDTA control, the whole labelling process was done in PBS 1X instead of annexin V buffer 1X.
Flow cytometer sample analysis
To analyse EVs using flow cytometry, we used an improved high sensitivity flow cytometer (hs-FCM) Canto II special order product equipped with a small particle option (BD Biosciences) (Citation28). The forward scatter (FSC) on this dedicated equipment is coupled to a photomultiplier tube (PMT) with a 488 nm solid-state, 100 mW output blue laser (rather than the conventional 20 mW) and includes a 633 nm HeNe, 20 mW output red laser and a 405 nm solid state diode, 50 mW output violet laser. The hs-FCM is equipped with FSC-PMT and a Fourier optical transformation unit, reducing the background/noise and increasing the angle of diffusion, thus enhancing the detection of small diameter particles. The hs-FCM performance tracking was performed daily before all analyses using the BD cytometer setup and tracking beads (BD Biosciences). Size estimation was determined using silica beads of 100, 500 and 1,000 nm (Kisker Biotech GmbH & Co., Steinfurt, Germany), whereas volume quantification was performed by adding a known number of 3 µM dim polystyrene microspheres to each sample (BD Biosciences).
Statistics
Data are expressed as the mean±SEM. Statistical differences were assessed using a 2-tailed non-parametric Student's t-test, with p<0.05 reported as statistically significant, using Prism software, version 6.0c (GraphPad California (CA), United States). All experiments contained 3 or more replicate mice per experimental parameter.
Results and discussion
Heterogeneous populations of EVs are present in lymph
Recently, emerging small-particle option analysing systems have been optimized to fully characterize EVs, and access to these tools awakens interest in a better understanding of the evident role of lymph as a route for EVs. Herein, we sought to assess their presence in circulating lymph using 3 different and complementary functional assays. First, to assess the purity of the lymph samples and the absence of possible contamination of blood that might occur during lymph collection (a), the number of AO− cells larger than 5 µM (e.g. RBCs, leukocytes) was determined in the lymph and compared to that of whole blood. We verified that, in contrast to blood, the lymph contained only a negligible trace of RBCs (0.11% compared to whole blood), thus confirming the high purity of the lymph preparations used for this study (b). In addition, by counting the number of nucleated (AO+) cells in lymph before and after centrifugation, we demonstrated that centrifugation eliminated the vast majority of the cells in lymph samples (c). These observations validate our assertion that our pre-analytical conditions were optimal for the assessment of EVs in lymph.
Fig. 1. Assessment of lymph purity following collection and processing. (a) Lymph was collected from the thoracic duct (dotted circle) on EDTA (0.1 M); following sample collection, cell counting was performed with a Nexcelom Auto X4 cell counter before (total lymph) and after (supernatant) centrifugation. Cells were stained with acridine orange (AO), a nuclear staining (nucleic acid binding) fluorescent dye that stains all nucleated cells, in whole blood and total lymph. The number of AO− cells larger than 5 µM in diameter (i.e. red blood cells) was assessed using brightfield and fluorescence imaging for AO. (b) Total lymph was purposely contaminated at different concentrations (10−1, 10−2, 10−3) with whole blood (WB) and our sample of interest (lymph) was then standardized to WB and compared to all concentrations and lymph supernatant (control). (c) Total number of AO+ cells was detected in total lymph and lymph supernatant using fluorescence imaging for AO. (n=4 per group; *p<0.05, **p<0.001).
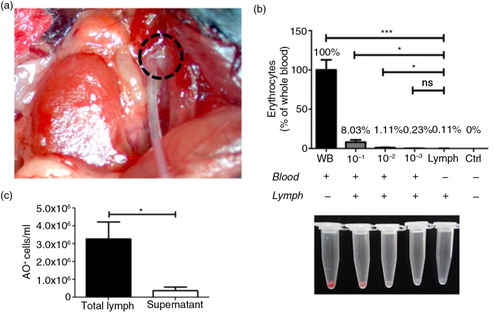
In the first technique using cryo-EM, we detected spherical EVs with a diameter ranging from 50 to 800 nm (). As compared to the plasma samples (Citation29), the lymph samples seemed to contain a significantly higher amount of EVs. Hence, although cryo-EM is not a quantitative method, the fact that 2 or more EVs were frequently observed at close distance (d) is in marked contrast with what is observed with pure plasma, which further excludes the impact of potential contamination of blood in the lymph analyses. Of interest, most EVs were not labelled by annexin V conjugated with gold nanoparticles, indicating that most do not expose phosphatidylserine (PS) on their outer surface (a through c). In addition to spherical EVs, some exhibiting multilayered structures (c) were also observed. Furthermore, another class of objects was abundant in the lymph samples. These objects present a mostly circular shape and a uniform greyness by cryo-EM. The absence of a lipid bilayer at their periphery enabled them to be easily distinguished from EVs (b and f); they are referred to hereafter as lipoproteins. Surprisingly, lipoproteins were observed not only isolated in the lymph medium (f) but also encapsulated within EVs (c). The size of the lipoproteins varied from 100 nm to several 100 nm, yet smaller lipoproteins may well be present in lymph but are not detected here. Thus, EVs of various dimensions are abundant in lymph, and a fraction of them expose PS.
Fig. 2. Representative cryo-electron microscopy images of lymph extracellular vesicles (EVs). (a through c) EVs devoid of annexin V gold nanoparticles indicate the absence of phosphatidylserine (PS) on their outer membrane surface. (d through f) EVs are labelled by annexin V gold nanoparticles, with the exception of the small unlabelled EVs marked with a black arrow. (b, d) The black asterisks point to circular shapes devoid of a lipid bilayer, which could most likely be lipoproteins. (a, c through e) The white asterisks point to areas of the supporting perforated carbon net. EVs devoid of annexin V gold nanoparticles (g) and EVs labelled by annexin V gold nanoparticles in the lymph of atherosclerotic LDLR-/- mice. Scale bars: 100 nm.
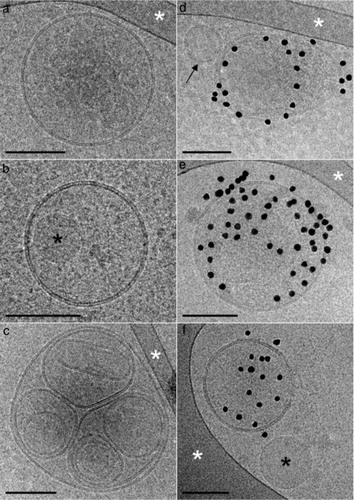
A second approach we used was hs-FCM adapted with a small particle option. Silica beads are more appropriate than polystyrene beads to obtain an EV size estimation in flow cytometry because their refraction index is closer to that of EVs (Citation30). Thus we used silica beads of known dimensions and intact platelets to determine the lower and upper limits of our EV gate, respectively (a through c). To prudently assess EVs in complex fluids, we defined EVs as membrane cargo, containing active esterases (CFSE+), and positive for at least one surface marker such as PS or protein receptor (e.g. CD41). Although we recognize that extracellular esterases, complexed with lipoproteins or lipoprotein-like particles, might also be labelled with CFSE, such a combination of complementary markers greatly enhances the specificity of the approach. Having confirmed the specificity of our hs-FCM measurements using calcium chelation to prevent annexin V recognition of PS and of detergent to dissolve EVs (d through g), we found profuse amounts of CFSE+ particles in lymph, half of them exposing PS, suggesting that they were indeed EVs. Although these observations contrast with those made using cryo-EM, which revealed the preponderance of EVs lacking surface PS, they also suggest that a proportion of PS- EVs remains undetected by hs-FCM, consistent with the lower limit of the EV gate, which we had intentionally set at approximately 100 nm accordingly to the silica beads.
Fig. 3. Detection of EVs in lymph using flow cytometry. (a through e) A Canto II flow cytometer modified with a “small particle” FSC PMT-H option was used to detect and quantify silica microspheres of 100 nm (red), 500 nm (green) and 1,000 nm (violet) mean diameter and EVs. (a, b) An EV gate was designed for the detection of small particles from 100 to 1,000 nm in diameter, based on the silica microspheres sizes (FSC PMT-H). (c) CD41+ mouse platelets are excluded from the EV gate based on their size (FSC PMT-H). Representative flow cytometry dot plot of lymph (d) total CFSE+ and (e) CFSE+PS+ EVs in control conditions or treated with 0.05% Triton or 50 M EDTA. Sensitivity to (f) Triton or (g) EDTA of EVs.
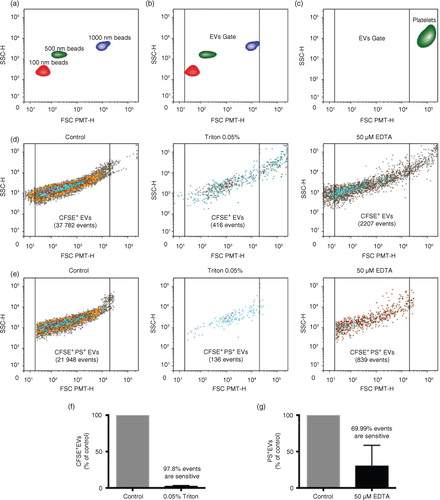
EV levels are modulated in lymph during atherosclerosis
One of the main roles of the lymphatic system is to transport immune cells from peripheral tissues to lymphoid organs (Citation1). Inflammatory cell accumulation in peripheral tissues drives several chronic inflammatory diseases such as atherosclerosis (Citation31) and obesity (Citation32), in which lymphatic transport has been reported as being defective (Citation7, Citation10). A growing number of studies are reporting that EVs are active pro-inflammatory players also present in inflammatory sites, becoming important biomarkers in several pathological settings. Altogether, these recent studies converge toward a key role of the lymphatic network in the clearance and production of EVs, thus explaining why EVs are more numerous in chronic inflammatory diseases. However, to our knowledge, this hypothesis has never been directly addressed.
Lymph composition is different than that of plasma or serum, and its content also varies according to the pathological setting. Studies have revealed that cholesterol-fed animals have higher phospholipid content, cholesterol-to-protein ratio and free cholesterol levels in their circulating lymph (Citation33, Citation34). In a first set of experiments, we sought to investigate whether the morphology and origin of EVs vary in WT versus atherosclerotic LDLR-/- mice. Size of nanoparticles was estimated using hydrodynamics, which does not however distinguish EVs from aggregated proteins and lipoproteins. Although this approach might not be optimal for the examination of polydisperse EV populations, it successfully revealed that, compared to WT animals, the main population of nanoparticles in lymph in atherosclerotic mice displayed a modest increase in diameter (175±55 nm vs. 133±41 nm). In addition, a second population of smaller nanoparticles (56±10 nm) could be observed in these animals (). Whereas lymph composition appears altered in our atherosclerotic mouse model, further investigations are needed to determine whether this effect is due to hypercholesterolemia per se.
Fig. 4. Dynamic light scattering size characterization of particles present in lymph of wild-type (WT) and LDLR-/- mice. Dimensions of the particles present in lymph from WT and LDLR-/- mice were determined with a Zetasizer Nano S, Malvern Instruments, UK Experiments were performed with 3 mice per experimental group.
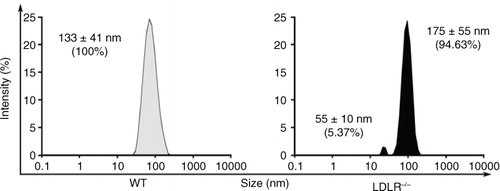
The most studied and abundant EV populations recognized in blood are platelets and RBCs (Citation29, Citation35). In a normal physiological state, lymph is relatively free of RBCs. However, lymphatic transport impairment has been associated with improper lymphatic integrity and linked to the presence of RBC in lymph due to a defective LV junction (Citation13). Platelets are key players in that process, mainly through the binding of C-type lectin-like receptor 2 (CLEC2) to LEC podoplanin. CLEC2 is constitutively expressed on the surface of blood platelets, and its interaction with podoplanin initiates a complex formed by fibrin-containing platelet thrombi that protects lymph from backward flow. Therefore, we hypothesized that EVs derived from both platelets and RBCs could accumulate in the lymph of mice displaying a defective lymphatic transport, as their lymphatic vessel integrity could be impaired.
To test our hypothesis, we used our adapted hs-FCM technique to measure platelet- and RBC-derived EVs. Overall, EV concentrations are increased in atherosclerosis (a). Indistinguishably from PS exposure, EVs from both platelet (CD41+) and RBC (Ter119+) origin are more abundant in the lymph of atherosclerotic animals (c and e), thus confirming our hypothesis. We suggest that the presence of RBC-derived EVs is due to an inappropriate backward flow from the LV or to a defective lymphatic endothelial cell-to-cell junction, thus reflecting increased vascular permeability. Whether the large accumulation of platelet-derived EVs in atherosclerotic lymph is a cause or a consequence of lymphatic dysfunction remains to be tested. We bring forward new concepts that could explain the presence of platelet-derived EVs in lymph. Therefore, we hypothesize that the stagnation of platelet EVs in lymph could either reflect (a) the incapacity of the lymphatic vessels to propel lymph down the road and thus to efficiently clear the interstitial space or (b) the incapacity of platelets to bind to LEC at the LV, which could lead to their shedding and backward flow in lymph. These hypotheses are currently under intensive investigation. Whereas PS+ EVs from various origins were clearly increased, PS- EVs were not significantly higher in sick animals (b, d, and f), reflecting that the impaired clearance of apoptotic cells in chronic inflammation and atherosclerosis is also observed in lymph.
Fig. 5. Characterization of EVs in lymph of atherosclerotic mice. Flow cytometry was used to identify (a) total CFSE+ EVs based on their (b) PS expression. (c) CFSE+CD41+ and (e) CFSE+ Ter119+ EVs were analysed based on their (d, f) PS expression. Experiments were performed with 3 mice per experimental group. (*p<0.05, **p<0.01, ***p<0.001).
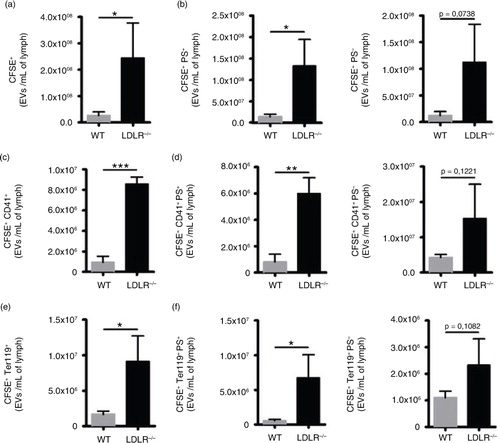
Although the functional consequences of the presence of EVs in lymph remain to be established, this study reports that EVs, of diverse sources, are transported in lymph. EVs are involved in numerous physiological processes, and vesicles from both non-immune and immune cells have important roles in immune regulation. Whereas several stimuli, such as cytokines, lead to EV release from macrophages and monocytes in the atherosclerotic lesion (Citation36), EVs produced by blood endothelial cells (BEC) can also be found in blood (Citation37). As pro-inflammatory cytokines and nitric oxide can trigger BEC-derived EVs production (Citation38), it would be of interest to test whether these players involved in lymphatic function could also promote LEC-derived EVs in atherosclerosis-related collecting lymphatic dysfunction (Citation39). Albeit the full assessment of EV origin (e.g. immune cells) and subtypes (i.e. exosomes, microvesicles or apoptotic cells) using complementary biochemical approaches (Citation40) would have been of interest, the volume of lymph collected was not suitable to perform these additional experiments and other studies will characterize them without doubt in the near future. At present, we speculate that lymph contains both exosomes and microvesicles and that the CD41+ and Ter1119+ EVs, with their size and markers, correspond mainly to microvesicles derived from platelets (or megakaryocytes) and RBCs, respectively. We observed that a major proportion of EVs did not harbour surface PS. Considering that PS is implicated in the rapid clearance of EVs (Citation22), PS- EVs may predominantly accumulate in the lymphatic system where they might interact with other cells and lymphoid organs. Whereas the routes and mechanisms of EV uptake have been described extensively in the past few years (Citation41), our results complement these findings and bring forth a possible direct role of the lymphatic network in the transport and also the production of EVs. Our results suggest that EVs derived from RBCs and platelets, inclusively but not exclusively, circulate in the lymph of healthy animals and are morphologically different and abundant in atherosclerosis. Whether increased concentrations of EVs in lymph in atherosclerosis and other chronic inflammatory diseases reflect a cause or a consequence of lymphatic dysfunction remains under intensive investigation.
Conflict of interest and funding
The authors have no other relevant affiliations or financial involvement with any organization or entity with a financial interest in or financial conflict with the subject matter or materials discussed in the manuscript apart from those disclosed.
Authors’ contributions
AM, NT, ÉB and CM designed and carried out the experiments, analysed the data and wrote the manuscript. ST and AB contributed to the experimental design, revised the manuscript and analysed the data.
Acknowledgements
We thank Tania Lévesque and François Dallaire for their technical help. This work was partially supported by the Montreal Heart Institute Foundation (CM), the Banting Research Foundation (CM) and a Canadian Institutes of Health Research (CIHR) Foundation grant (EB). EB is the recipient of a CIHR new investigator award, CM is the recipient of a FRQS Research Scholars Career Award - Junior 1 and NT of a fellowship from the Fonds de Recherche sur les Maladies Rhumatismales de l'Université Laval.
References
- Tammela T, Alitalo K. Lymphangiogenesis: molecular mechanisms and future promise. Cell. 2010; 140: 460–76.
- Zolla V, Nizamutdinova IT, Scharf B, Clement CC, Maejima D, Akl T, etal. Aging-related anatomical and biochemical changes in lymphatic collectors impair lymph transport, fluid homeostasis, and pathogen clearance. Aging Cell. 2015; 14: 582–94.
- Blum KS, Karaman S, Proulx ST, Ochsenbein AM, Luciani P, Leroux JC, etal. Chronic high-fat diet impairs collecting lymphatic vessel function in mice. PLoS One. 2014; 9: e94713.
- Lim HY, Rutkowski JM, Helft J, Reddy ST, Swartz MA, Randolph GJ, etal. Hypercholesterolemic mice exhibit lymphatic vessel dysfunction and degeneration. Am J Pathol. 2009; 175: 1328–37.
- Vuorio T, Nurmi H, Moulton K, Kurkipuro J, Robciuc MR, Ohman M, etal. Lymphatic vessel insufficiency in hypercholesterolemic mice alters lipoprotein levels and promotes atherogenesis. Arterioscler Thromb Vasc Biol. 2014; 34: 1162–70.
- Angeli V, Llodra J, Rong JX, Satoh K, Ishii S, Shimizu T, etal. Dyslipidemia associated with atherosclerotic disease systemically alters dendritic cell mobilization. Immunity. 2004; 21: 561–74.
- Martel C, Li W, Fulp B, Platt AM, Gautier EL, Westerterp M, etal. Lymphatic vasculature mediates macrophage reverse cholesterol transport in mice. J Clin Invest. 2013; 123: 1571–9.
- Eliska O, Eliskova M, Miller AJ. The absence of lymphatics in normal and atherosclerotic coronary arteries in man: a morphologic study. Lymphology. 2006; 39: 76–83.
- Miller AJ, DeBoer A, Palmer A. The role of the lymphatic system in coronary atherosclerosis. Med Hypotheses. 1992; 37: 31–6.
- Torrisi JS, Hespe GE, Cuzzone DA, Savetsky IL, Nitti MD, Gardenier JC, etal. Inhibition of inflammation and iNOS improves lymphatic function in obesity. Sci Rep. 2016; 6: 19817.
- Baluk P, Fuxe J, Hashizume H, Romano T, Lashnits E, Butz S, etal. Functionally specialized junctions between endothelial cells of lymphatic vessels. J Exp Med. 2007; 204: 2349–62.
- Zawieja D. Lymphatic biology and the microcirculation: past, present and future. Microcirculation. 2005; 12: 141–50.
- Hess PR, Rawnsley DR, Jakus Z, Yang Y, Sweet DT, Fu J, etal. Platelets mediate lymphovenous hemostasis to maintain blood-lymphatic separation throughout life. J Clin Invest. 2014; 124: 273–84.
- Zaleska M, Olszewski WL, Durlik M, Miller NE. Signaling proteins are represented in tissue fluid/lymph from soft tissues of normal human legs at concentrations different from serum. Lymphat Res Biol. 2013; 11: 203–10.
- Gyorgy B, Szabo TG, Pasztoi M, Pal Z, Misjak P, Aradi B, etal. Membrane vesicles, current state-of-the-art: emerging role of extracellular vesicles. Cell Mol Life Sci. 2011; 68: 2667–88.
- Colombo M, Raposo G, Thery C. Biogenesis, secretion, and intercellular interactions of exosomes and other extracellular vesicles. Annu Rev Cell Dev Biol. 2014; 30: 255–89.
- Diamant M, Tushuizen ME, Sturk A, Nieuwland R. Cellular microparticles: new players in the field of vascular disease?. Eur J Clin Invest. 2004; 34: 392–401.
- Buzas EI, Gyorgy B, Nagy G, Falus A, Gay S. Emerging role of extracellular vesicles in inflammatory diseases. Nat Rev Rheumatol. 2014; 10: 356–64.
- Boilard E, Blanco P, Nigrovic PA. Platelets: active players in the pathogenesis of arthritis and SLE. Nat Rev Rheumatol. 2012; 8: 534–42.
- Loyer X, Vion AC, Tedgui A, Boulanger CM. Microvesicles as cell-cell messengers in cardiovascular diseases. Circ Res. 2014; 114: 345–53.
- Van Der Pol E, Boing AN, Gool EL, Nieuwland R. Recent developments on the nomenclature, presence, isolation, detection and clinical impact of extracellular vesicles. J Thromb Haemost. 2016; 14: 48–56.
- Boilard E, Duchez AC, Brisson A. The diversity of platelet microparticles. Curr Opin Hematol. 2015; 22: 437–44.
- Pitt JM, Kroemer G, Zitvogel L. Extracellular vesicles: masters of intercellular communication and potential clinical interventions. J Clin Invest. 2016; 126: 1139–43.
- Laffont B, Corduan A, Ple H, Duchez AC, Cloutier N, Boilard E, etal. Activated platelets can deliver mRNA regulatory Ago2*microRNA complexes to endothelial cells via microparticles. Blood. 2013; 122: 253–61.
- Laffont B, Corduan A, Rousseau M, Duchez AC, Lee CH, Boilard E, etal. Platelet microparticles reprogram macrophage gene expression and function. Thromb Haemost. 2016; 115: 311–23.
- Duchez AC, Boudreau LH, Bollinger J, Belleannee C, Cloutier N, Laffont B, etal. Platelet microparticles are internalized in neutrophils via the concerted activity of 12-lipoxygenase and secreted phospholipase A2-IIA. Proc Natl Acad Sci USA. 2015; 112: E3564–73.
- Srinivasan S, Vannberg FO, Dixon JB. Lymphatic transport of exosomes as a rapid route of information dissemination to the lymph node. Sci Rep. 2016; 6: 24436.
- Rousseau M, Belleannee C, Duchez AC, Cloutier N, Levesque T, Jacques F, etal. Detection and quantification of microparticles from different cellular lineages using flow cytometry. Evaluation of the impact of secreted phospholipase A2 on microparticle assessment. PLoS One. 2015; 10: e0116812.
- Arraud N, Linares R, Tan S, Gounou C, Pasquet JM, Mornet S, etal. Extracellular vesicles from blood plasma: determination of their morphology, size, phenotype and concentration. J Thromb Haemost. 2014; 12: 614–27.
- Chandler WL. Measurement of microvesicle levels in human blood using flow cytometry. Cytometry B Clin Cytom. 2016; 90: 326–36.
- Libby P, Ridker PM, Maseri A. Inflammation and atherosclerosis. Circulation. 2002; 105: 1135–43.
- Greenberg AS, Obin MS. Obesity and the role of adipose tissue in inflammation and metabolism. Am J Clin Nutr. 2006; 83: 461S–5S.
- Sloop CH, Dory L, Krause BR, Castle C, Roheim PS. Lipoproteins and apolipoproteins in peripheral lymph of normal and cholesterol-fed dogs. Atherosclerosis. 1983; 49: 9–21.
- Sloop CH, Dory L, Hamilton R, Krause BR, Roheim PS. Characterization of dog peripheral lymph lipoproteins: the presence of a disc-shaped “nascent” high density lipoprotein. J Lipid Res. 1983; 24: 1429–40.
- Arraud N, Gounou C, Turpin D, Brisson AR. Fluorescence triggering: a general strategy for enumerating and phenotyping extracellular vesicles by flow cytometry. Cytometry A. 2015; 89: 184–95.
- Jungel A, Distler O, Schulze-Horsel U, Huber LC, Ha HR, Simmen B, etal. Microparticles stimulate the synthesis of prostaglandin E2 via induction of cyclooxygenase 2 and microsomal prostaglandin E synthase 1. Arthritis Rheum. 2007; 56: 3564–74.
- Chistiakov DA, Orekhov AN, Bobryshev YV. Extracellular vesicles and atherosclerotic disease. Cell Mol Life Sci. 2015; 72: 2697–708.
- Szotowski B, Antoniak S, Goldin-Lang P, Tran QV, Pels K, Rosenthal P, etal. Antioxidative treatment inhibits the release of thrombogenic tissue factor from irradiation- and cytokine-induced endothelial cells. Cardiovasc Res. 2007; 73: 806–12.
- Milasan A, Dallaire F, Mayer G, Martel C. Effects of LDL Receptor modulation on lymphatic function. Sci Rep. 2016; 6: 27862.
- Lotvall J, Hill AF, Hochberg F, Buzas EI, Di Vizio D, Gardiner C, etal. Minimal experimental requirements for definition of extracellular vesicles and their functions: a position statement from the International Society for Extracellular Vesicles. J Extracell Vesicles. 2014; 3: 26913. doi: http://dx.doi.org/10.3402/jev.v3.26913.
- Mulcahy LA, Pink RC, Carter DR. Routes and mechanisms of extracellular vesicle uptake. J Extracell Vesicles. 2014; 3: 24641, doi: http://dx.doi.org/10.3402/jev.v3.24641.