Abstract
The mitochondrial quality control system regulating mitochondria biogenesis, dynamics, and degradation has been extensively studied because of its roles in normal cell homeostasis and dysfunction due to aging or disease. Mitochondria degradation is generally thought to occur by autophagy and has therefore been viewed as a cell-autonomous process. In a recent study, we demonstrated that a large fraction of retinal ganglion cell mitochondria undergo lysosomal degradation within the astrocytes of the optic nerve head. It will be important to determine whether other neurons with long axons also use transcellular mitophagy, or transmitophagy, as a primary mitochondrial quality control mechanism either under normal physiological conditions or in disease. The elucidation of the underlying molecular mechanisms is necessary to determine whether defects in transmitophagy are involved in pathogenesis and whether it should become a therapeutic target.
Relatively little is known about how neurons with long axons degrade mitochondria that, in many cases, can be centimeters away from the soma. Mitochondria might undergo degradation in the axoplasm with the assistance of local axonal lysosomes, by macro- or microautophagy of whole mitochondria or mitochondria-derived vesicles (). Autophagosome-enclosed mitochondria or dysfunctional mitochondria produced by asymmetric fission events might instead undergo retrograde transport back to the soma for degradation by somatic lysosomes (). However, the experimental data supporting some of these cell-autonomous modes of axonal mitochondria degradation have come largely from either cultured cells or pathological specimens. Our recent findings indicate that, under normal physiological conditions in vivo, axonal mitochondria of one type of long-projection neuron are instead enclosed in axoplasmic membranes that are shed and degraded by neighboring cells ().
Figure 1. Degradation of axonal mitochondria may occur by several pathways. (A) Degradation of axonal mitochondria by lysosome (Ly) fusion with autophagosomes (AP) or shed mitochondria-derived vesicles locally within axons. Bidirectional arrow indicates a fission event, and mitochondrial matrix color indicates acidification state, where yellow represents neutral pH and red represents acidification, as reported by the MitoEGFPmCherry transgene. (B) Retrograde axonal transport of either autophagosome-sequestered mitochondria or dysfunctional mitochondria derived from asymmetric fission events can enable degradation of axonal mitochondria within the often-distant cell soma. (C) Axonal transmitophagy.
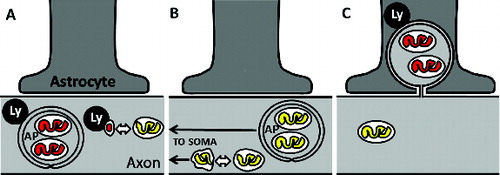
Retinal ganglion cells (RGCs) project axons from the eye to image- and nonimage-processing areas of the brain. In humans and most mammals, RGC axons are unmyelinated as they converge onto the optic disc within the retina and travel through a meshwork of orthogonally oriented astrocytic processes in the lamina of the optic nerve head (ONH). RGC axons become myelinated in the retrolaminar region of the ONH and remain so in the rest of the optic nerve. The majority of RGC mitochondria reside in their axons, as the length of their axons are at least 3 orders of magnitude greater than their soma diameter. High densities of mitochondria are needed in unmyelinated regions of axons, nodes of Ranvier, and synaptic terminals to satisfy the high energetic demands of nerve fiber conduction and neurotransmitter release.
In order to identify the contents of RGC axonal protrusions and evulsions that we had previously reported at the ONH of normal mice, we recently re-examined the ONH with structural and molecular tools. Electron microscopy (EM) studies using transmission EM, EM-tomography, and block-face scanning EM demonstrated that large protrusions, some of which are pinching off from morphologically healthy RGC axons, contain large numbers of mitochondria. Additional EM experiments found similar foci of clustered degrading mitochondria on neurites in superficial layers of the cerebral cortex in young wild-type mice, though at a much lower density than at the ONH. A new dual-fluorophore transgene, MitoEGFPmCherry, which behaves similarly to dual-fluorophore LC3 reporters used extensively in autophagy research, was used to report the location of acidified RGC mitochondria. MitoEGFPmCherry was introduced into RGCs by ocular injection of an AAV vector, and was used to image the acidified RGC mitochondria in the retina and ONH. This viral reporter showed that acidified RGC axonal mitochondria are found in abundance in the ONH within astrocytes and associated with lysosomes. A combination of mitochondrial DNA fluorescence in situ hybridization and a novel use of terminal deoxynucleotidyl dUTP nick end-labeling (TUNEL) demonstrated extensive mitochondria degradation within the ONH astrocytes. Furthermore, colabeling of MitoEGFPmCherry and TUNEL demonstrated that the acidified RGC mitochondria within the ONH are being degraded. Finally, MitoEGFPmCherry-labeled RGC mitochondria were quantified by 2 methods in the ONH relative to the RGC layer of the retina. Surprisingly, MitoEGFPmCherry reported a greater fraction of RGC mitochondria acidified in the ONH than in the RGC layer.
Additional experiments are needed to determine whether this mode of axonal mitochondria degradation is common in other types of neurons under normal physiological conditions, or in pathological conditions. There is extensive evidence of defective mitochondrial quality control and abnormal autophagic and phagocytic activities in neurodegenerative disorders, including Alzheimer and Parkinson diseases, and amyotrophic lateral sclerosis. Interestingly, structurally similar axonal elaborations filled with mitochondria have been reported in aged monkey brains and proposed to be an alternative mode of degradation for axonal materials that might lead to plaque formation in Alzheimer disease. The different pathways that regulate axonal mitochondrial turnover should continue to be characterized in these and other disorders, in vivo where possible. The particular pathway or pathways primarily responsible for mitochondrial turnover likely vary in different types of neurons, even in different long-projection neurons, as they differ greatly in their axon to soma ratio, myelination pattern, mitochondrial load, and susceptibility to mechanical or biochemical stressors. Thus, determining the primary and backup modes of mitochondria degeneration in different cellular compartments of different neurons involved in neurodegenerative disorders likely will be complicated, but nonetheless should be prioritized.
The discovery of transmitophagy of ONH axons evokes other recently described transcellular degradation processes. The convergence of molecular machineries involved in autophagy and phagocytosis has been described in a process termed LC3-associated phagocytosis (LAP). LAP is involved in antigen processing after degradation of pathogens and phagocytic clearance of apoptotic cells by macrophages, and in the phagocytosis of photoreceptor outer segments by retinal pigment epithelial cells. It is possible that ONH transmitophagy involves astrocytic LAP, as it is conceptually similar to the degradation of both distal tips of photoreceptor outer segments and pathogens. Further elucidation of phagocytic and autophagic mechanisms that might be involved in transmitophagy is needed as we seek interventions for the ONH axonal insult that blinds in glaucoma, and perhaps also for the axonal insults that often occur early in various other neurodegenerative disorders.
Disclosure of Potential Conflicts of Interest
No potential conflicts of interest were disclosed.