Abstract
In a determinate meristem, such as a floral meristem, a genetically determined number of organs are produced before the meristem is terminated. In rice, iterative formation of organs during flower development with defects in meristem determinacy, classically called ‘proliferation’, is caused by several mutations and observed in dependence on environmental conditions. Here we report that overexpression of several JAZ proteins, key factors in jasmonate signaling, with mutations in the Jas domains causes an increase in the numbers of organs in florets, aberrant patterns of organ formation and repetitious organ production in spikelets. Our results imply that JAZ factors modulate mechanisms that regulate meristem functions during spikelet development.
Abbreviations
bHLH | = | basic helix–loop–helix |
JA | = | jasmonate |
JAZ | = | Jasmonate zim domain |
LSH1 | = | Leafy hull sterile1 |
MJA | = | methyl jasmonate |
mJAZ | = | mutated JAZ |
RT-PCR | = | reverse transcription-PCR |
RSS3 | = | Rice salt sensitive 3 |
qRT-PCR | = | quantitative real-time RT-PCR |
SAM | = | shoot apical meristem |
Meristems provide cells that differentiate and produce organs to sustain plant growth. In indeterminate meristems, such as vegetative and inflorescence meristems, meristematic activity is maintained by continuous cell proliferation balanced with cell differentiation. By contrast in determinate meristems, such as floral meristems, meristematic activity ceases after initiation of a certain number of organs. In general, floral meristem determinacy is regulated genetically. In Arabidopsis, for example, AGAMOUS has a role in repression of WUSCHEL that is required for maintenance of stem cells in the meristem during flower development (for a review see ref. 1). Strong agamous mutant alleles develop a set of floral organs (sepals–petals–petals) repeatedly, instead of carpel formation, reflecting prolonged maintenance of an active meristem at the center of the flower.Citation1 In rice, flower development occurs in a spikelet, a special inflorescence unit, which produces 2 rudimentary glumes and 2 sterile lemmas and a floret that comprises floral organs (pistil, stamen and lodicule) and outer organs (palea and lemma) (for reviews see refs. 2–3). Determinacy of the floral meristem in rice is controlled by OsMADS58,Citation4 whereas determinacy of the spikelet meristem and transition of the spikelet meristem to the floral meristem is regulated by OsMADS1/LEAFY HULL STERILE1 (LHS1).Citation3,5 Indeterminate development of flowers consisting of lodicules, stamens and caperl-like organ, but not palea and lemma, was caused by RNA siliencing of OsMADS58.Citation4 In lhs1 mutants, an additional flower generation in a floret and repetitious formation of leaf-like paleae and lemmas within a spikelet is often observed.Citation2,5
In rice, various types of abnormal spikelet development are induced by cold weather. These include an increase in the number of spikelet organs and formation of multiple-floret spikelets.Citation6 Moreover, findings of ‘proliferated propagule spikelet’ formation has been reported in a field survey of cold damages and is characterized by development of foliaceous glumes and leaves in a spikelet,Citation6 resembling the phenotype observed in lhs1 as described above. Takeoka and Shimizu (1973)Citation7 reported similar defects in a rice mutant where repetitious formation of vegetative organs (‘leafy-shoot-type proliferation’) or floral organs (‘spikelet-type proliferation’) occurs in a spikelet. This process is accompanied by enlargement of the meristem.Citation8 Moreover, in ‘leafy-shoot-type proliferation’, reversion to vegetative growth occurs.Citation7 Interestingly, whether vegetative organs or floral organs are additively formed in a spikelet depends on seasonal change.Citation9 Thus, in addition to genetic factors, environmental conditions appear to affect meristem determinacy and meristem identity, although the underlying mechanisms are not understood.
Jasmonate (JA) and its derivatives (JAs) act as phytohormones that regulate plant growth, leaf senescence and defense responses (for reviews see refs. 10–11). JA production is activated by several biotic and abiotic stresses, including wounding, pathogen infection and salt and drought stresses.Citation11,12 JA mediates responses to such stresses, and it is inactivated by negative feedback regulation to prevent exaggerated responses.Citation13-16 In plant cells, JA is converted into JA-Ile that is recognized by binding to the COI1 receptor and JAZ proteins.Citation17-19 This binding facilitates ubiquitination of JAZ factors by SCFCOI1 resulting in its degradation by 26S proteasomes.Citation20,21 In the absence of JA, JAZ factors repress the activity of bHLH transcription factors, whereas in the presence of JA, JAZ degradation leads to activation of genes downstream of the bHLH factors.Citation20,21 More recently, proteins other than bHLH factors have been reported to bind to JAZs, suggesting that JAZs modulate several intracellular signaling pathways.Citation22
In rice (Oryza sativa, cv. Nipponbare), there are 15 JAZ factors encoded in the genome, suggesting that their structural variation is responsible for versatile roles of JAs.Citation15 In this study, to investigate the functions of JAZs in rice, we designed genes encoding mutated JAZ (mJAZ) factors that carry 2 amino acid substitutions in Jas domains (). These mutations abolish binding of JAZ to COI1 but not to a basic helix-loop-helix (bHLH) transcription factor AtMYC2, an Arabidopsis homolog of myelocytomatosis oncoprotein MYC, and thus alleviates JA responses in Arabidopsis.Citation23 In rice, similar defects in JA signaling are expected in tissues where endogenous JAZ genes are expressed (). We prepared transgenic plants in which mJAZs were overexpressed by a rice Actin gene promoter using a Ti plasmid vector bearing the Gateway cassette.Citation24 Up to 10 times higher expression levels of JAZs in leaves were observed in transgenic lines with mJAZ (mJAZox) than in those with the vector control. These plants exhibited low fertility and interestingly, several aberrant patterns of organ formation in their spikelets (). In wild-type (WT) rice, spikelet development involves formation of 2 rudimentary glumes and 2 sterile lemmas besides transition from the spikelet meristem into the floral meristem, which subsequently produces one lemma, one palea, 2 lodicules, 6 stamens and one pistil along with terminal differentiation of the meristem.Citation2,3 By contrast, in the spikelets of transgenic plants expressing mJAZ3, mJAZ6, mJAZ7 and mJAZ11, but not the vector control, an increase in the number of palea/lemma-like organs or aberrant glume-like structures was frequently observed (; Le-l and A-Gl, ). These were often associated with ectopic development of tissues resembling spikelets (; E-Sp) or florets (; E-Fl) and a reduction in the number of internal floral organs (). Organ identity was often lost, resulting in aberrant structure of organs, such as elongated lodicule (E-Lo) and elongated sterile lemma (E-Sl), or appearance of mosaic organs. In some cases, the number of stamens increased in a spikelet (). We also observed an increase in the number of palea- or lemma-like organs in several lines that expressed mJAZ4 in addition to the lines described above. These results suggest that expression of mJAZ factors affects the mechanism that regulates transition of meristem fate (transition from spikelet meristem to flower meristem), meristem determinacy and organ identity during spikelet development in rice. Considering the generation of leafy glume-like organs (L-Gl) and pedicel-like organs (Pe-l), loss of identity of the lemma and palea and the reversion of the spikelet meristem to the branch meristem may also be caused by mJAZ expression. Collectively, different mJAZ constructs induced overlapping morphological defects (), although patterns of phenotype appeared, depending on the respective mJAZs.
Figure 1. Rice JAZ factors. (A) Jas domains of rice JAZ proteins. Amino acid sequences of the Jas domains were aligned using Clustal Omega (https://www.ebi.ac.uk/Tools/msa/clustalo/). The consensus sequence is indicated at the bottom. The 2 conserved positively charged amino acids shaded with yellow colors were substituted with alanine in the mutated JAZs. The other highly conserved amino acids are shaded in light blue. Names of JAZs are as reported in ref. 30 JAZ1, Os04g0653000 (OsTIFY3); JAZ2, Os07g0153000 (OsTIFY5); JAZ3, Os08g0428400 (OsTIFY6a); JAZ4, Os09g0401300 (OsTIFY6b), JAZ5, Os04g0395800 (OsTIFY9); JAZ6, Os03g0402800 (OsTIFY10a); JAZ7, Os07g0615200 (OsTIFY10b); JAZ8, Os09g0439200 (OsTIFY10c); JAZ9, Os03g0180800 (OsTIFY11a); JAZ10, Os03g0181100 (OsTIFY11b); JAZ11, Os03g0180900 (OsTIFY11c); JAZ12, Os10g0392400 (OsTIFY11d); JAZ13, Os10g0391400 (OsTIFY11e), JAZ14, Os10g0391801 (OsTIFY11f); JAZ15, Os03g0396500 (OsTIFY11g). (B) Expression of JAZ genes in rice tissues. The levels of respective JAZs were determined by RT-PCR. Arrows indicate bands specific to respective JAZs. RNA was extracted from root and shoot tissues of 1-week-old seedlings and basal shoot tissues (1 cm long) of 2-week-old seedlings grown on the MS-based medium.15 Reverse transcription was performed using the Quanti-Tect Rev transcription kit (Qiagen). First-strand cDNA was amplified by PCR using KOD-FX Neo (Toyobo) or EX-Taq (Takara). For amplification and subcloning of cDNA into pENTR-/D-Topo (Invitrogen), primers for JAZ1 (5′-caccATGGATCTGTTGGAGAAGAAG-3′ and 5′-TTACTGGGCCTTGCCCTCAG-3′), JAZ2 (5′-caccATGGCGGAGGAGCGGAGGAGAGACGAC-3′ and 5′-CTATCGCCGGGCGTACAGCGGCGCGG-3′), JAZ3 (5′-caccATGGAGAGGGATTTTCTTGGC-3′ and 5′-TCATATCTGTAACTTTGTGCTGGGGGGC-3′), JAZ4 (5′-caccATGGAGAGGGACTTCCTGGG-3′ and 5′-TCAGATTTGTAGCTTTGTACTGGGGGACTCC-3′), JAZ5 (5′-caccATGTCGACGAGGGCGCC-3′ and 5′-CTAGGACGCCGTGTGCTCCTCTTCCTTC-3′), JAZ6 (5′-caccATGGCTTCCGCGAAATC-3′ and 5′-TCATTGGCTCGATTCCTGCCG-3′), JAZ7 (5′-caccATGGCGGCTTCCGCGAGG-3′ and 5′-TCATTGGCCGCGTTCTATGGGCTTCACG-3′), JAZ8 (5′-caccATGGCCGGCCGTGC-3′ and 5′-TCATATCTCCTGCTTTATTGTCATCTCTTGGCC-3′), JAZ9 (5′-caccATGGCGTCGACGGATCCCAT-3′ and 5′-TCAGCGCGAGTGCATGTGTCCAA-3′), JAZ10 (5′-caccATGGCGATGGAGGGGAAGAGC-3′ and 5′-TCACAGCGCGATGGTGAGGCTGTC-3′), JAZ11 (5′-caccATGGCCGGTAGTAGCGAGCA-3′ and 5′-TCACAGGCTGAGAGTGGGGTTCACCT-3′), JAZ12 (5′-caccATGGCCGCCGCCGGCAGCAGC-3′ and 5′-TCAGAGCCCGAGCCATGTCGCCGGCTCA-3′) and for JAZ15 (5′-caccATGGACGCCGTCGGCGCAG-3′ and 5′-TCACTTTTGCTTCCTCTTTTGGAGGAATCG-3′), respectively, were used. After subcloning, mutations were introduced by site-directed mutagenesis, using primers for mJAZ3 (5′-GCCTCAAGCTGCAGCAGCATCTCTCG-3′ and 5′-CGAGAGATGCTGCTGCAGCTTGAGGC-3′), mJAZ4 (5′-CCCTCAAGCGGCGGCGGCATCCCTTG-3′ and 5′-CAAGGGATGCCGCCGCCGCTTGAGGG-3′), mJAZ6 (5′-GCCTCAGGCTGCGGCGGCGTCGCTTC-3′ and 5′-GAAGCGACGCCGCCGCAGCCTGAGGC-3′), mJAZ7 (5′-GCCTATTGCTGCGGCGGCATCACTCC-3′ and 5′-GGAGTGATGCCGCCGCAGCAATAGGC-3′) and mJAZ11 (5′-GCCCATCGCGGCGGCGGTGTCGCTGC-3′ and 5′-GCAGCGACACCGCCGCCGCGATGGGC-3′), respectively.
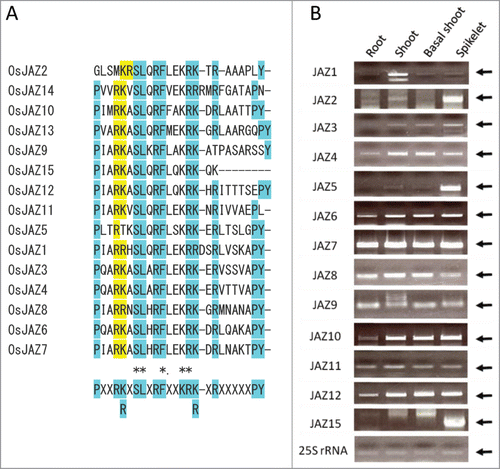
Table 1. Summary of aberrant morphogenesis observed in the transgenic lines
Figure 2. Morphology of spikelets in transgenic rice lines overexpressing mJAZ (A, B) A line carrying empty vector. (C, D) mJAZ3ox, line Y10. Note that an ectopic spikelet-like structure (E-Sp) has formed in a spikelet. To indicate internal tissues, outer and floral organs are separated in (B, D). (E) mJAZ6ox, Line1. Leafy glume-like organs (L-Gl) that exhibited curved structure along the apical-basal axis, resembling leaf blades, generated repetitively. (F) mJAZ7ox, Line5. (Inset) Internal tissues visualised by detaching glumes. No stamens or pistil have been formed. A-Gl, aberrant glume-like structure; E-Lo, elongated lodicule; E-Sp, ectopic spikelet-like organ; E-SL, elongated sterile lemma; L-Gl; leafy glume-like organ; Le, lemma; Le-l, lemma-like organ; Lo, lodicule; Pa, palea; Pe-l; pedicel-like organ; Pi, pistil; RG, rudimentary glume; SL, sterile lemma; St, stamen. For overexpression of mJAZ, respective cDNAs carrying the mutations were introduced into the binary vector pSMAHdN637L-GateA and used for Agrobacterium tumefaciens-mediated transformation of rice (Oryza sativa, cv Nipponbare) as described in ref. 24 Insertion of respective transgenes in the genome DNA was confirmed by PCR amplification. For preparation of an empty vector control, 1.7-kb DNA fragment containing ccdB and sequences for site-specific recombination (attR1 and attR2) were eliminated from the vector plasmid by digestion with XbaI and KpnI, followed by blunting and ligation.
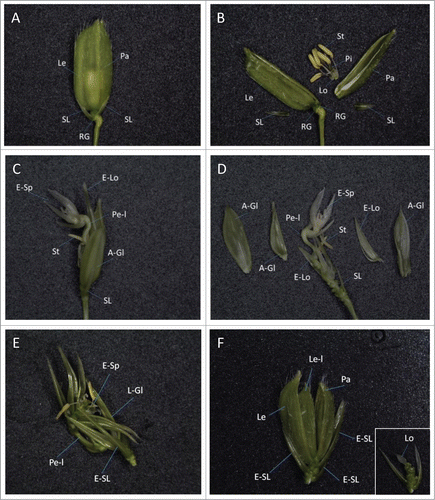
Figure 3. Repetitious formation of floral and outer organs in a spikelet of a transgenic rice. (A–D) A spikelet of a transgenic line (mJAZ11ox, Line A1). (A) A pedicel-like organ (Pe-I), carrying ectopic floral organs, formed at the center of the spikelet. (B) The pedicel-like organ was dissected from the center of the spikelet. Note that leafy glume-like organs (L-Gl) developed repetitiously. (C) A magnified view of the ectopic floral organ formation at the base of the pedicel-like organ. Mosaic of pistil- and lodicule-like organs (Mo) formed on an axillary branch. (D) A magnified view of the ectopic floral organs formed on the apex of the pedicel-like organ. Note the position of the pistils inside and outside of the repetitively formed elongated lodicules (E-Lo). (E–G) A spikelet of a transgenic line (mJAZ11ox, Line 4). (E) Repetitious formation of glumes in the spikelet. (F, G) Internal organs of the spikelet, visualised by removing 2 lemmas (F) and leafy glume-like organs (G; magnified). E-Fl, ectopic floret; E-Lo, elongated lodicule; E-SL, elongated sterile lemma; Le, lemma; L-Gl; leafy glume-like organ; Lo, lodicule; Pe-l; pedicel-like organ; Mo, mosaic organ; Pi, pistil; SL, sterile lemma; St, stamen.
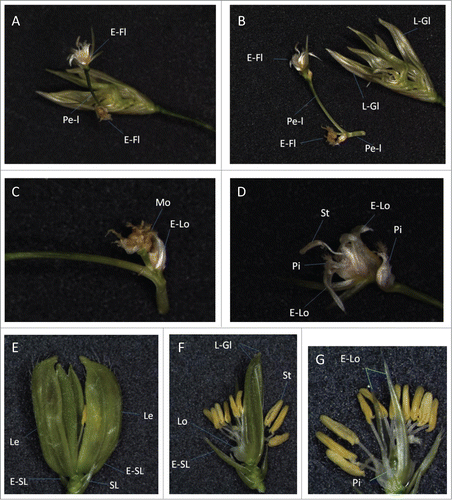
Repetitive formation of palea/lemma-like organs or glume-like structures is reminiscent of repetitive development of glumes (bracts) observed in the double mutant of indeterminate spikelete1 (ids1) and sister of indeterminate spikelet 1 (sid1) of maize.Citation25 In the supernumerary bract (snb) mutant of rice, repetitious formation of rudimentary glumes in a spikelet is observed.Citation26 In these mutants, the transition from the spikelet meristem to the flower meristem is delayed, leading to continuous cell division, allowing development of extra organs that enclose flower meristem(s). By contrast, in our transgenic lines, we could not observe such repetitious formation of rudimentary glumes. Repetitive formation of lemma/palea-like organs caused by expression of several mJAZs in our study likely reflects a delay in terminal differentiation of floral organs and a prolongation of cell proliferation of the flower meristem cells. This process may also account for an increase in internal flower organs as shown in . However, we observed deficiencies of palea or lemma development in some lines expressing mJAZ7 and mJAZ11 (, Type 6), suggesting that the program of organ determination can be disrupted in a different manner, possibly depending on the balance of mJAZ and endogenous JAZs in the specific developmental timing.
Several lines of evidence suggest that JA is involved in spikelet development in rice. In a JA-deficient mutant of rice, in which the function of allene oxide cyclase is disrupted, formation of extra lemma/palea is often observed.Citation27 Moreover, the extra glume1 (eg1) mutant of rice, in which a gene encoding a plastid-localized lipase required for JA production is mutated, shows changed floral organ number and iterative formation of glume-like structures.Citation28,29 The eg2–1D mutant of rice exhibits defects in spikelet development similar to those of eg1. Dominant eg2–1D has a point mutation, resulting in an amino acid substitution (Ala to Gly) in the Jas domain of JAZ1 (OsTIFY3), which binds to OsCOI1b and OsMYC2.Citation29 This mutation weakens the binding to OsCOI1b and thereby disrupts JA-dependent degradation of the JAZ factor, a process that is proposed to release the repression of OsMYC2 activity and downstream OsMADS1/LHS1 gene.Citation29 These results are consistent with our findings, although alteration of organ pattern formation appears to have been more drastic in our study. This alteration is exemplified by the repetitious formation of a unit including pistils (), suggesting that cell proliferation before differentiation of the flower meristem is prolonged not only just after development of rudimentary glumes and sterile lemmas, but also after repetitive initiation of outer organs (palea and lemma). A possible explanation for such differences in phenotypic severity is that mJAZs in our study were more stable than JAZ mutated in eg2–1D. Alternatively, activity of several factors binding to mJAZs, in addition to OsMYC2, might also be repressed.
Our results suggest that JAZ factors modulate regulatory systems involved in specification of meristem identity and meristem determinacy in the rice spikelet, and that expression of mJAZ factors affects such regulation, resulting in pleiotropic defects including repetitious formation of floral and outer organs. Some of these may mimic the defects in JA signaling that is coupled with developmental program of spikelet. However, it should also be noted that many of rice JAZ genes, including those examined with the mutations in this study, are induced by treatment with JA and/or by abiotic stresses, such as cold and drought and salt stress.Citation30 It is plausible that various types of abnormal spikelet development observed under stressful conditions is linked to function of these JAZ factors. Interestingly, an increase in the number of organs (palea/lemma, lodicule, stamen, and pistil) and morphological changes (such as elongation of lodicules) during spikelet development has been reported in rice, in which a gene encoding jasmonic acid carboxyl methyltransferase (JMT) is over-expressed under the control of maize ubiquitin1 promoter.Citation31 This aberrant morphogenesis in the transgenic lines are associated with enlarged spikelet meristem and increased accumulation of methyl jasmonate (MJA) in young panicles.Citation31 Overexpression of JMT may also result in alleviation in JA-signaling in rice, because it negatively affects JA-Ile formation in Nicotiana attenuate.Citation32 On the other hand, similar alteration in the number of organs and morphological changes in the spikelet is observed in WT after treatment with MJA or drought.Citation31 Considering these phenomena together with our results, induction of JAZ expression by abiotic stresses and in the process of feedback regulation of JA-signaling might be involved in defective spikelet development, when grown under stressful conditions. Further studies on function of JAZ factors and their binding proteins will help us to understand how meristem functions are modified by environmental factors.
Disclosure of Potential Conflicts of Interest
No potential conflicts of interest were disclosed.
Acknowledgments
We thank Drs. Hidemi Kitano, Yutaka Sato, Hiro-Yuki Hirano, Ahmed Ismail and Taisuke Nishimura for helpful discussion, Dr. Hiroaki Ichikawa for providing the binary vector pSMAHdN637L-GateA, Kyo Kurata for helpful technical comments and Mami Sugiura for technical assistance.
Funding
This work was supported in part by JSPS KAKENHI (24580493).
References
- Sablowski R. Flowering and determinacy in arabidopsis. J Exp Bot 2007; 58:899-907; PMID:17293602
- Hirano HY, Tanaka W, Toriba T. Grass flower development. Methods Mol Biol 2014; 1110:57-84; PMID:24395252; http://dx.doi.org/10.1007/978-1-4614-9408-9_3
- Tanaka W, Pautler M, Jackson D, Hirano HY. Grass meristems II: inflorescence architecture, flower development and meristem fate. Plant Cell Physiol 2013; 54:313-24; PMID:23378448; http://dx.doi.org/10.1093/pcp/pct016
- Yamaguchi T, Lee DY, Miyao A, Hirochika H, An G, Hirano HY. Functional diversification of the two C-class MADS box genes OSMADS3 and OSMADS58 in Oryza sativa. Plant Cell 2006; 18:15-28; PMID:16326928
- Jeon JS, Jang S, Lee S, Nam J, Kim C, Lee SH, Chung YY, Kim SR, Lee YH, Cho YG, et al. leafy hull sterile1 is a homeotic mutation in a rice MADS box gene affecting rice flower development. Plant Cell 2000; 12:871-84; PMID:10852934
- Takeoka Y, Shimizu M, Wada T. Panicles. In: Matsuo T, Hoshikawa K, eds. Science of the Rice Plant, Vol.1. Morphology, Tokyo: Food and Agriculture Policy Research Center, 1993, pp. 295-338
- Takeoka Y, Shimizu M. Vegetative proliferations of frolal spikelets in Oryza sativa L.: II. The proliferative structure in the spikelets of the mutant strain induced by X-ray irradiation. Proc Crop Sci Soc Japan 1973; 42:520-26
- Takeoka Y, Shimizu M. Vegetative proliferations of frolal spikelets in Oryza sativa L.: IV. Histo- and organo-genesis in the proliferated spikelets (I). Proc Crop Sci Soc Japan 1974; 43:445-52
- Takeoka Y, Shimizu M. Vegetative proliferations of frolal spikelets in Oryza sativa L.: V. Seasonal change of the type of proliferations which differentiate in the spikelets of a mutant induced by X-ray irradiation. Proc Crop Sci Soc Japan 1974; 43:523-30
- Creelman RA, Mullet JE. Biosynthesis and action of jasmonate in plants. Annu Rev Plant Physiol Plant Mol Biol 1997; 48:355-81; PMID:15012267
- Wasternack C. Jasmonates: an update on biosynthesis, signal transduction and action in plant stress response, growth and development. Ann Bot 2007; 100:681-97; PMID:17513307
- Takeuchi K, Gyohda A, Tominaga M, Kawakatsu M, Hatakeyama A, Ishii N, Shimaya K, Nishimura T, Riemann M, Nick P, et al. RSOsPR10 expression in response to environmental stresses is regulated antagonistically by jasmonate/ethylene and salicylic acid signaling pathways in rice roots. Plant Cell Physiol 2011; 52:1686-96; PMID:21828106; http://dx.doi.org/10.1093/pcp/pcr105
- Koo AJ, Cooke TF, Howe GA. Cytochrome P450 CYP94B3 mediates catabolism and inactivation of the plant hormone jasmonoyl-L-isoleucine. Proc Natl Acad Sci USA 2011; 108:9298-303; PMID:21576464; http://dx.doi.org/10.1073/pnas.1103542108
- Heitz T, Widemann E, Lugan R, Miesch L, Ullmann P, Désaubry L, Holder E, Grausem B, Kandel S, Miesch M, et al. Cytochromes P450 CYP94C1 and CYP94B3 catalyze two successive oxidation steps of plant hormone Jasmonoyl-isoleucine for catabolic turnover. J Biol Chem 2012; 287:6296-306; PMID:22215670; http://dx.doi.org/10.1074/jbc.M111.316364
- Toda Y, Tanaka M, Ogawa D, Kurata K, Kurotani K, Habu Y, Ando T, Sugimoto K, Mitsuda N, Katoh E, et al. RICE SALT SENSITIVE3 forms a ternary complex with JAZ and class-C bHLH factors and regulates jasmonate-induced gene expression and root cell elongation. Plant Cell 2013; 25:1709-25; PMID:23715469; http://dx.doi.org/10.1105/tpc.113.112052
- Toda Y, Yoshida M, Hattori T, Takeda S. RICE SALT SENSITIVE3 binding to bHLH and JAZ factors mediates control of cell wall plasticity in the root apex. Plant Signal Behav 2013; 8:e26256; PMID:23989667; http://dx.doi.org/10.4161/psb.26256
- Staswick PE, Tiryaki I. The oxylipin signal jasmonic acid is activated by an enzyme that conjugates it to isoleucine in Arabidopsis. Plant Cell 2004; 16:2117-27; PMID:15258265
- Katsir L, Schilmiller AL, Staswick PE, He SY, Howe GA. COI1 is a critical component of a receptor for jasmonate and the bacterial virulence factor coronatine. Proc Natl Acad Sci USA 2008; 105:7100-5; PMID:18458331; http://dx.doi.org/10.1073/pnas.0802332105
- Sheard LB, Tan X, Mao H, Withers J, Ben-Nissan G, Hinds TR, Kobayashi Y, Hsu FF, Sharon M, Browse J, et al. Jasmonate perception by inositol-phosphate-potentiated COI1-JAZ co-receptor. Nature 2010; 468:400-5; PMID:20927106; http://dx.doi.org/10.1038/nature09430
- Chini A, Fonseca S, Fernández G, Adie B, Chico JM, Lorenzo O, García-Casado G, López-Vidriero I, Lozano FM, Ponce MR, et al. The JAZ family of repressors is the missing link in jasmonate signalling. Nature 2007; 448:666-71; PMID:17637675
- Thines B, Katsir L, Melotto M, Niu Y, Mandaokar A, Liu G, Nomura K, He SY, Howe GA, Browse J. JAZ repressor proteins are targets of the SCF(COI1) complex during jasmonate signalling. Nature 2007; 448:661-5; PMID:17637677
- Kazan K, Manners JM. JAZ repressors and the orchestration of phytohormone crosstalk. Trends Plant Sci 2012; 17:22-31; PMID:22112386; http://dx.doi.org/10.1016/j.tplants.2011.10.006
- Melotto M, Mecey C, Niu Y, Chung HS, Katsir L, Yao J, Zeng W, Thines B, Staswick P, Browse J, et al. A critical role of two positively charged amino acids in the Jas motif of Arabidopsis JAZ proteins in mediating coronatine- and jasmonoyl isoleucine-dependent interactions with the COI1 F-box protein. Plant J 2008; 55:979-88; PMID:18547396; http://dx.doi.org/10.1111/j.1365-313X.2008.03566.x
- Hakata M, Nakamura H, Iida-Okada K, Miyao A, Kajikawa M, Imai-Toki N, Jinhuan P, Kou A, Akihiko H, Tomoko T-M, et al. Production and characterization of a large population of cDNA-overexpressing transgenic rice plants using Gateway-based full-length cDNA expression libraries. Breed Sci 2010; 60:575-85; http://dx.doi.org/10.1270/jsbbs.60.575
- Chuck G, Meeley R, Hake S. Floral meristem initiation and meristem cell fate are regulated by the maize AP2 genes ids1 and sid1. Development 2008; 135:3013-9; PMID:18701544; http://dx.doi.org/10.1242/dev.024273
- Lee DY, An G. Two AP2 family genes, supernumerary bract (SNB) and Osindeterminate spikelet 1 (OsIDS1), synergistically control inflorescence architecture and floral meristem establishment in rice. Plant J 2012; 69:445-61; PMID:22003982; http://dx.doi.org/10.1111/j.1365-313X.2011.04804.x
- Riemann M, Haga K, Shimizu T, Okada K, Ando S, Mochizuki S, Nishizawa Y, Yamanouchi U, Nick P, Yano M, et al. Identification of rice Allene Oxide Cyclase mutants and the function of jasmonate for defence against Magnaporthe oryzae. Plant J 2013; 74:226-38; PMID:23347338; http://dx.doi.org/10.1111/tpj.12115
- Li H, Xue D, Gao Z, Yan M, Xu W, Xing Z, Huang D, Qian Q, Xue Y. A putative lipase gene EXTRA GLUME1 regulates both empty-glume fate and spikelet development in rice. Plant J 2009; 57:593-605; PMID:18980657; http://dx.doi.org/10.1111/j.1365-313X.2008.03710.x
- Cai Q, Yuan Z, Chen M, Yin C, Luo Z, Zhao X, Liang W, Hu J, Zhang D. Jasmonic acid regulates spikelet development in rice. Nat Commun 2014; 5:3476; PMID:24647160; http://dx.doi.org/10.1038/ncomms4476
- Ye H, Du H, Tang N, Li X, Xiong L. Identification and expression profiling analysis of TIFY family genes involved in stress and phytohormone responses in rice. Plant Mol Biol 2009; 71:291-305; PMID:19618278; http://dx.doi.org/10.1007/s11103-009-9524-8
- Kim EH, Kim YS, Park SH, Koo YJ, Choi YD, Chung YY, Lee IJ, Kim JK. Methyl jasmonate reduces grain yield by mediating stress signals to alter spikelet development in rice. Plant Physiol. 2009; 149:1751-60; PMID:19211695; http://dx.doi.org/10.1104/pp.108.134684
- Stitz M, Gase K, Baldwin IT, Gaquerel E. Ectopic expression of AtJMT in Nicotiana attenuata: creating a metabolic sink has tissue-specific consequences for the jasmonate metabolic network and silences downstream gene expression. Plant Physiol 2011; 157:341-54; PMID:21753114; http://dx.doi.org/10.1104/pp.111.178582