Abstract
The hypothesis that plants can benefit from a memory of past stress exposure has recently attracted a lot of attention. Here, we discuss two different examples of heat stress memory to elucidate the potential benefits that epigenetic responses may provide at both the level of acclimation of the individual plant and adaptation at a species-wide level. Specifically, we discuss how microRNAs regulate the heat stress memory and thereby increase survival upon a recurring heat stress. Secondly, we review how a prolonged heat stress in a small interfering RNA-deficient background induces retrotransposition that is transmitted to the next generation, thus creating genetic variation for natural selection to act on. Collectively, these studies reveal a crucial role of short RNAs in heat stress memory across different time scales.
Abbreviations
HSP | = | heat shock protein |
HSF | = | heat shock transcription factor |
HS memory | = | heat stress, memory |
miRNA | = | microRNA |
siRNA | = | small interfering RNA |
Abiotic stress is a major factor limiting crop productivity, and this problem is likely to be exacerbated in the future. The analysis of the early responses to abiotic stress in Arabidopsis thaliana has led to important insights into how stress tolerance is achieved molecularly.Citation1 However, it remains unclear how plants deal with enduring or recurring stress conditions. Numerous observations support the notion that plants have a cellular memory of abiotic stress exposure, yet the underlying molecular mechanisms are largely unknown. Here, we suggest an operational definition of memory, which is not only applicable to memory in the narrow sense, i. e. memory associated with nervous systems and behavior, but also to other memory processes such as machine learning and memory-like processes in plants and other organisms lacking a central nervous system. According to this definition, the term memory is applicable when three requirements are fulfilled: (1) Activation of the memory by information provided by external or intrinsic cues; (2) active storage of the memory after the external/ intrinsic cue has subsided, (3) retrieval of the memory, which is evident from a modified response to a secondary external cue or modified development. A classic example for memory from the plant kingdom is the phenomenon of vernalization, where a prolonged period of cold temperature (such as winter) accelerates the transition to flowering weeks or months after the cold has subsided (such as spring).Citation2,3 In Arabidopsis, vernalization involves the epigenetic silencing of a floral repressor through chromatin modification. An example for biotic stress memory is priming, where a pathogen elicits a response that leads to an altered reaction upon a secondary infection, which occurs at a later point in time.Citation4 In systemic acquired resistance a local infection triggers a systemic response that protects the plant beyond the infected leaf.Citation5 Once induced, systemic acquired resistance lasts for weeks or months. There is evidence that the memory of systemic acquired resistance involves specific chromatin modifications independent of changes in gene expression.Citation6-8 Priming may also be associated with costs due to the allocation of resources, the targeted reduction of growth, or the metabolic load of defense compounds. In the case of priming with the chemical compound β-aminobutyric acid, priming was reported to entail only slightly lower fitness in the absence of secondary stress events.Citation9
The discovery of epigenetic regulatory mechanisms over the past decades has provided an attractive mechanistic hypothesis about how plant memory could be established and maintained over time, and how gene expression upon a recurring stress could be modified. These mechanisms include DNA methylation and the posttranslational modification of histone tails on the nucleosomes. Some of these modifications are self-maintained; others are directed by complementary small interfering RNAs (siRNAs) that act both in the establishment and in the maintenance of these modifications. Together, these epigenetic mechanisms act in the suppression of (retro)transposon activity. Excellent reviews cover the mechanism of epigenetic silencing in detail.Citation10-12 MicroRNAs (miRNAs) are small RNAs that are derived from dedicated miRNA genes and that repress their target genes mostly at the posttranscriptional level through cleavage of complementary transcripts or translational inhibition.Citation13,14 In addition, a recent study implicates miRNAs in transposon silencing.Citation15
Several groups have reported transgenerational effects of stress exposure at the morphological and epigenetic level (most recently in Ref.Citation16,17). However, the significance and extent of transgenerational stress memory is still far from resolved.Citation18 This area has been discussed recently by an excellent review and will not be covered here.Citation18
Stress exposure can also lead to reactivation of transposons und repetitive elements, which was already described by Barbara McClintock, who referred to it as “genomic shock”.Citation19-21 Through transposition, this reactivation introduces mutations at the single-gene level, but can also cause large chromosomal rearrangements, thus creating genetic variation for selection to act on. Although this process is undirected, it has the potential to generate plants that are better adapted to stressful environments. In the following, we focus on the memory triggered by heat stress exposure and discuss two recent examples that illustrate the concept of memory at different scales.
Heat stress induces immediate responses that are well studied and conserved across kingdoms. They involve the massive induction of heat shock proteins (HSP) through members of the heat shock transcription factor (HSF) family.Citation22 Besides the immediate responses that lead to the acquisition of thermotolerance and that last only a few hours after the end of the stress, heat stress triggers a memory process that is referred to as maintenance of acquired thermotolerance, heat stress memory or long-term acquired thermotolerance.Citation23,24 This is an active process that is genetically separable from the acquisition as several mutants with normal acquisition of thermotolerance, but a defect in the maintenance of acquired thermotolerance, have been described.Citation25-27 They include mutants in HEAT SHOCK TRANSCRIPTION FACTOR A2 (HSFA2), which encodes a heat inducible HSF transcription factor, HEAT SHOCK-ASSOCIATED 32 (HSA32), which encodes a protein of unknown function and ROF1, which encodes a peptidyl-prolyl cis-trans isomerase of the FKBP (FK506-binding protein) family.
Very recently, a role for miRNAs in heat stress memory was reported.Citation23 The authors showed that the miRNA pathway and in particular its components ARGONAUTE1, DICER-LIKE1 and SUO, are required for an intact heat stress memory. miR156 was highly induced by heat stress and activation persisted for several days. Functional analysis showed that miR156 is required for heat stress memory, and that increased miR156 levels can prolong the memory. miR156 has previously been implicated in the regulation of leaf initiation rates and the developmental transitions from the juvenile to the adult vegetative phase and from the vegetative to the reproductive phase.Citation28 A common action of miR156 in those pathways is the repression of members of the SQUAMOSA PROMOTOR BINDING PROTEIN-LIKE (SPL) transcription factor family. In heat stress memory, downregulation of SPL2 and additional targets by miR156 also is an essential step. This double function of the miR156-SPL module in heat stress memory and the regulation of development may serve to integrate development with stressful environmental conditions (). As miR156 has been hypothesized to report on the internal age of the plant,Citation29,30 this integration may involve the adjustment of developmental time with the time spent under stressful conditions. The repression of target genes by miRNAs may be especially suited to integrate stress responses over time, as, in the case of translational inhibition, the repression is reversible and a degradation of the miRNA will quickly relieve the block on translation. Other small RNAs (miR398, TAS1) were recently implicated in the acquisition of thermotolerance,Citation31,32 however, it remains to be tested whether they also play a role in heat stress memory.
Figure 1. Heat stress memory is regulated by the HSFA2 cascade and the miR156-SPL module. Heat stress induces expression of HSFA2 which in turn activates HSA32 and additional target genes that maintain acquired thermotolerance (HS memory). This also requires ROF1 activity. Maintenance of acquired thermotolerance is also promoted by increased repression of SPL2 and other targets through miR156. Biogenesis of miR156 requires DCL1 and repression of SPL2 and other target mRNAs requires AGO1. The miR156-SPL module also regulates leaf initiation rates and the transition to flowering. Thus, its dual role in heat stress memory and the regulation of development may serve to integrate development with environmental stress.
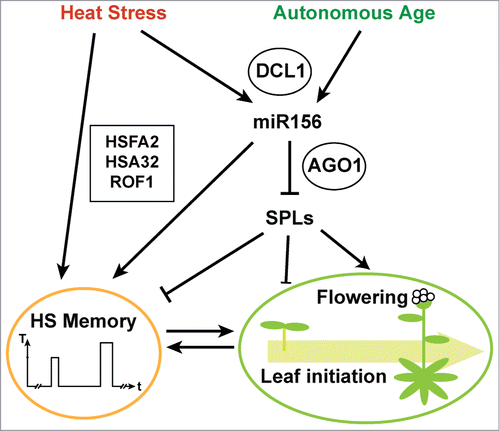
Does heat stress memory also involve changes at the chromatin level? This is an attractive hypothesis, as specific marks on the chromatin may allow specific loci to remain in a hyperactivatable state that would either enable their sustained induction or their accelerated or enhanced re-induction upon a repeated heat stress. It remains to be investigated whether such a mechanism directly enhances heat stress memory at individual loci of associated genes.
Transposable elements make up a large part of eukaryotic genomes.Citation20 Due to their mutagenic potential, host organisms have evolved pathways that tightly control their activity and these involve silencing at the transcriptional and posttranscriptional level. It is well-known that stress exposure can interfere with transposon silencing and lead to transient loss of transcriptional silencing that is, however, re-established quickly after the stress has subsided in most cases.Citation33-35 The re-establishment of silencing after stress requires both DDM1 and MOM1, both of which have been implicated in transposon silencing.Citation36 Among the transposable elements reactivated by a prolonged heat stress is the COPIA retroelement ONSEN/COPIA78. Citation33,37 ONSEN inactivation is independent of DNA methylation, but requires components of the siRNA-directed silencing pathway.Citation37
After prolonged heat stress, new ONSEN copies arise through retrotransposition in mutants deficient in siRNA biogenesis and are transmitted into the next generation.Citation37 Interestingly, the retrotransposition occurs during flower development and before gametogenesis and may be linked to actively dividing cells.Citation37,38 Furthermore, ONSEN retrotransposition can confer heat-inducibility onto neighboring genes.Citation37 Thus, retrotransposition of ONSEN induces not only insertional mutations, but also has the potential to create new regulatory connections; both features create genetic variation that natural selection for improved heat resistance can act on. Very recently, the mechanism of the heat-dependent induction of ONSEN has been discovered.Citation39 Through heat-stress response elements in its long terminal repeat, ONSEN utilizes heat stress response components of the host for its activation. Specifically, ONSEN activation requires HSFA1 and HSFA2 transcription factors. Short heat stress was not effective in activating ONSEN transcription. Thus, it is plausible that HSFA2 (which is downstream of HSFA1 and required for heat stress memory) binds directly to the ONSEN long-terminal repeat. However, induction was not fully abolished in a hsfa2 knockout mutant and thus additional HSF proteins may be involved.Citation39 In summary, ONSEN hijacks the plant heat stress response, but in return the plant may take advantage of new transposition events to adapt to stressful conditions in the long term. This illustrates nicely the complex interactions between transposons and their host organisms, and the long-term impact of stress memory on adaptation.
Here, we have discussed two contrasting examples for heat stress memory, acting on different timescales and mechanisms but both having the potential to improve the ability of plants to cope with heat stress. It will be interesting to see whether these mechanisms are also applicable to other abiotic stresses.
Disclosure of Potential Conflicts of Interest
No potential conflicts of interest were disclosed.
Funding
Work in the Bäurle lab is funded by a Sofja-Kovalevskaja-Award (Alexander-von-Humboldt-Foundation), the DFG SFB 973 (Project A02), and the IMPRS Primary Metabolism and Plant Growth (IMPRS-PMPG, Max Planck Institute of Molecular Plant Physiology/ University of Potsdam).
References
- Jenks MA, Hasegawa PM. Plant Abiotic Stress. 2nd edn, (Wiley-Blackwell, 2013).
- Ream TS, Woods DP, Amasino RM. The molecular basis of vernalization in different plant groups. Cold Spring Harb Symp Quant Biol 2012; 77:105-15; PMID:23619014; http://dx.doi.org/10.1101/sqb.2013.77.014449
- Song J, Irwin J, Dean C. Remembering the prolonged cold of winter. Curr Biol 2013; 23:R807-811; PMID:24028964; http://dx.doi.org/10.1016/j.cub.2013.07.027
- Conrath U. Molecular aspects of defence priming. Trends Plant Sci 2011; 16:524-31; PMID:21782492; http://dx.doi.org/10.1016/j.tplants.2011.06.004
- Fu ZQ, Dong X. Systemic acquired resistance: turning local infection into global defense. Annu Rev Plant Biol 2013; 64:839-63; PMID:23373699; http://dx.doi.org/10.1146/annurev-arplant-042811-105606
- Dowen RH. Pelizzola M, Schmitz RJ, Lister R, Dowen JM, Nery JR, Dixon JE, Ecker JR. Widespread dynamic DNA methylation in response to biotic stress. Proc Natl Acad Sci U S A 2012; 109:E2183-2191; PMID:22733782; http://dx.doi.org/10.1073/pnas.1209329109
- Jaskiewicz M, Conrath U, Peterhänsel C. Chromatin modification acts as a memory for systemic acquired resistance in the plant stress response. EMBO Rep 2011; 12:50-55; PMID:21132017; http://dx.doi.org/10.1038/embor.2010.186
- Luna E, Bruce TJ, Roberts MR, Flors V, Ton J. Next-generation systemic acquired resistance. Plant Physiol 2012; 158:844-53; PMID:22147520; http://dx.doi.org/10.1104/pp.111.187468
- van Hulten M, Pelser M, van Loon LC, Pieterse CM, Ton J. Costs and benefits of priming for defense in Arabidopsis. Proc Natl Acad Sci U S A 2006; 103:5602-07; PMID:16565218; http://dx.doi.org/10.1073/pnas.0510213103
- Bucher E, Reinders J, Mirouze M. Epigenetic control of transposon transcription and mobility in Arabidopsis. Curr Opin Plant Biol 2012; 15:503-10; PMID:22940592; http://dx.doi.org/10.1016/j.pbi.2012.08.006
- Castel SE, Martienssen RA. RNA interference in the nucleus: roles for small RNAs in transcription, epigenetics and beyond. Nat Rev Genet 2013; 14:100-12; PMID:23329111; http://dx.doi.org/10.1038/nrg3355
- Rigal M, Mathieu OA. “mille-feuille” of silencing: epigenetic control of transposable elements. Biochim Biophys Acta 2011; 1809:452-8; PMID:21514406; http://dx.doi.org/10.1016/j.bbagrm.2011.04.001
- Axtell MJ. Classification and Comparison of Small RNAs from Plants. Annu Rev Plant Biol 2013; 64:137-59; PMID:23330790; http://dx.doi.org/10.1146/annurev-arplant-050312-120043
- Rogers K, Chen X. Biogenesis, Turnover, and Mode of Action of Plant MicroRNAs. Plant Cell 2013; 25:2383-99; PMID:23881412; http://dx.doi.org/10.1105/tpc.113.113159
- Creasey KM. Zhai J, Borges F, Van Ex F, Regulski M, Meyers BC, Martienssen RA. miRNAs trigger widespread epigenetically activated siRNAs from transposons in Arabidopsis. Nature 2014; 508:411-5; PMID:24670663; http://dx.doi.org/10.1038/nature13069
- Suter L, Widmer A. Environmental heat and salt stress induce transgenerational phenotypic changes in Arabidopsis thaliana. PLoS One 2013; 8:e60364; http://dx.doi.org/10.1371/journal.pone.0060364
- Migicovsky Z, Yao Y, Kovalchuk I. Transgenerational phenotypic and epigenetic changes in response to heat stress in Arabidopsis thaliana. Plant Signal Behav 2014; 9:e27971; PMID:24513700; http://dx.doi.org/10.4161/psb.27971
- Pecinka A, Mittelsten Scheid O. Stress-induced chromatin changes: a critical view on their heritability. Plant Cell Physiol 2012; 53:801-8; PMID:22457398; http://dx.doi.org/10.1093/pcp/pcs044
- McClintock B. The significance of responses of the genome to challenge. Science 1984; 226:792-801; PMID:15739260 http://dx.doi.org/10.1126/science.15739260
- Bennetzen JL, Wang H. The contributions of transposable elements to the structure, function, and evolution of plant genomes. Annu Rev Plant Biol 2014; 65:505-30; PMID:24579996; http://dx.doi.org/10.1146/annurev-arplant-050213-035811
- Levin HL, Moran JV. Dynamic interactions between transposable elements and their hosts. Nat Rev Genet 2011; 12:615-27; PMID:21850042; http://dx.doi.org/10.1038/nrg3030
- Mittler R, Finka A, Goloubinoff P. How do plants feel the heat? Trends Biochem Sci 2012; 37:118-25; PMID:22236506; http://dx.doi.org/10.1016/j.tibs.2011.11.007
- Stief A, Altmann S, Hoffmann K, Pant BD, Scheible WR, Bäurle I. Arabidopsis miR156 Regulates Tolerance to Recurring Environmental Stress through SPL Transcription Factors. Plant Cell 2014; 26:1792-807; PMID:24769482; http://dx.doi.org/10.1105/tpc.114.123851
- Yeh CH, Kaplinsky NJ, Hu C, Charng YY. Some like it hot, some like it warm: phenotyping to explore thermotolerance diversity. Plant Sci 2012; 195:10-23; PMID:22920995; http://dx.doi.org/10.1016/j.plantsci.2012.06.004
- Charng YY, Liu HC, Liu NY, Chi WT, Wang CN, Chang SH, Wang TT. A heat-inducible transcription factor, HsfA2, is required for extension of acquired thermotolerance in Arabidopsis. Plant Physiol 2007; 143:251-62; PMID:17085506; http://dx.doi.org/10.1104/pp.106.091322
- Charng YY, Liu HC, Liu NY, Hsu F C, Ko SS. Arabidopsis Hsa32, a novel heat shock protein, is essential for acquired thermotolerance during long recovery after acclimation. Plant Physiol 2006; 140:1297-305; PMID:16500991; http://dx.doi.org/10.1104/pp.105.074898
- Meiri D, Breiman A. Arabidopsis ROF1 (FKBP62) modulates thermotolerance by interacting with HSP90.1 and affecting the accumulation of HsfA2-regulated sHSPs. Plant J 2009; 59:387-99; PMID:19366428; http://dx.doi.org/10.1111/j.1365-313X.2009.03878.x
- Huijser P, Schmid M. The control of developmental phase transitions in plants. Development 2011; 138:4117-29; PMID:21896627; http://dx.doi.org/10.1242/dev.063511
- Wang JW, Czech B, Weigel D. miR156-regulated SPL transcription factors define an endogenous flowering pathway in Arabidopsis thaliana. Cell 2009; 138:738-49; PMID:19703399; http://dx.doi.org/10.1016/j.cell.2009.06.014
- Wu G, Park MY, Conway SR, Wang JW, Weigel D, Poethig RS. The sequential action of miR156 and miR172 regulates developmental timing in Arabidopsis. Cell 2009; 138, 750-9; PMID:19703400; http://dx.doi.org/10.1016/j.cell.2009.06.031
- Guan Q, Lu X, Zeng H, Zhang Y, Zhu J. Heat stress induction of miR398 triggers a regulatory loop that is critical for thermotolerance in Arabidopsis. Plant J 2013; 74:840-51; PMID:23480361; http://dx.doi.org/10.1111/tpj.12169
- Li S, Liu J, Liu Z, Li X, Wu F, He Y. Heat-induced tas1 target1 mediates thermotolerance via heat stress transcription factor a1a-directed pathways in arabidopsis. Plant Cell 2014; 26:1764-80; PMID:24728648; http://dx.doi.org/10.1105/tpc.114.124883
- Pecinka A, Dinh HQ, Baubec T, Rosa M, Lettner N, Mittelsten Scheid O. Epigenetic regulation of repetitive elements is attenuated by prolonged heat stress in Arabidopsis. Plant Cell 2010; 22:3118-29; PMID:20876829; http://dx.doi.org/10.1105/tpc.110.078493
- Lang-Mladek C, Popova O, Kiok K, Berlinger M, Rakic B, Aufsatz W, Jonak C, Hauser MT, Luschnig C. Transgenerational inheritance and resetting of stress-induced loss of epigenetic gene silencing in Arabidopsis. Mol Plant 2010; 3:594-602; PMID:20410255; http://dx.doi.org/10.1093/mp/ssq014
- Tittel-Elmer M, Bucher E, Broger L, Mathieu O, Paszkowski J, Vaillant I. Stress-induced activation of heterochromatic transcription. PLoS Genet 2010; 6:e1001175; PMID:21060865; http://dx.doi.org/10.1371/journal.pgen.1001175
- Iwasaki M, Paszkowski J. Identification of genes preventing transgenerational transmission of stress-induced epigenetic states. Proc Natl Acad Sci U S A 2014; 111:8547-52; PMID:24912148; http://dx.doi.org/10.1073/pnas.1402275111
- Ito H, Gaubert H, Bucher E, Mirouze M, Vaillant I, Paszkowski J. An siRNA pathway prevents transgenerational retrotransposition in plants subjected to stress. Nature 2011; 472:115-9; PMID:21399627; http://dx.doi.org/10.1038/nature09861
- Matsunaga W, Kobayashi A, Kato A, Ito H. The effects of heat induction and the siRNA biogenesis pathway on the transgenerational transposition of ONSEN, a copia-like retrotransposon in Arabidopsis thaliana. Plant Cell Physiol 2012; 53:824-33; PMID:22173101; http://dx.doi.org/10.1093/pcp/pcr179
- Cavrak VV, Lettner N, Jamge S, Kosarewicz A, Bayer LM, Mittelsten Scheid O. How a retrotransposon exploits the plant's heat stress response for its activation. PLoS Genet 2014; 10:e1004115; PMID:24497839; http://dx.doi.org/10.1371/journal.pgen.1004115