Abstract
Hymenaea courbaril or jatoba is a tropical tree known for its medically important secondary metabolites production. Considering climate change, the goal of this study was to investigate differential expression of proteins and lipids produced by this tree under heat stress conditions. Total lipid was extracted from heat stressed plant leaves and various sesquiterpenes produced by the tree under heat stress were identified. Gas chromatographic and mass spectrometric analysis were used to study lipid and volatile compounds produced by the plant. Several volatiles, isoprene, 2-methyl butanenitrile, β ocimene and a numbers of sesquiterpenes differentially produced by the plant under heat stress were identified. We propose these compounds were produced by the tree to cope up with heat stress. A protein gel electrophoresis (2-D DIGE) was performed to study differential expression of proteins in heat stressed plants. Several proteins were found to be expressed many folds different in heat stressed plants compared to the control. These proteins included heat shock proteins, histone proteins, oxygen evolving complex, and photosynthetic proteins, which, we believe, played key roles in imparting thermotolerance in Hymenaea tree. To the best of our knowledge, this is the first report of extensive molecular physiological study of Hymenaea trees under heat stress. This work will open avenues of further research on effects of heat stress in Hymenaea and the findings can be applied to understand how global warming can affect physiology of other plants.
Abbreviations
2D-DIGE | = | 2D-difference in gel electrophoresis; GC-MS, gas chromatography – linked to mass spectrometer; GO, gene ontology; HS, heat stressed; HSPs, heat shock proteins; MALDI-TOF, matrix-assisted laser desorption/ionization-time of flight; MAPK, mitogen-activated protein kinase; OEC, oxygen evolving enhancer protein; PP2C, protein phosphatase 2C; PSI, photosystem I; PSII, photosystem II; RuBP, ribulose 1, 5-bisphosphate; ROS, reactive oxygen species; RT, retention time; SN, serial number; TMV, tobacco mosaic virus; VOC, volatile organic compounds |
Introduction
Hymenaea courbaril L., popularly known as “jatoba” in Brazil, is a tropical leguminous tree species found commonly in semi-deciduous forests in South America. Out of 14 species of genus Hymenaea (Fabaceae), 9 are known to be found in different regions including lowland tropical ecosystem of Brazilian Amazon.Citation1,2 Almost all Hymenaea courbaril L. plant parts including roots, leaves, fruits and particularly stem barks are used traditionally for medicine and folk-medicine. Decoctions and infusions of this plant parts are used to treat wide range of diseases such as anemia, kidney problems, sore throat, bronchitis and asthma.Citation3 This plant is also used as an important tree in timber industry.Citation4
Climate change causing heat stressed conditions is a serious threat for agriculture and crop productivity. Oxygen, hydrocarbons, and chemicals emitted by the trees are collectively known as VOCs (Volatile Organic Compounds).Citation5 The increasing environment temperature also affects the volatile compounds production by the trees. Plants can emit 400–800 Tg C/year (1 Tg = 1032 g) as hydrocarbons.Citation6 Various terpene molecules produced by heat stressed Hymenaea plants were identified in this study. Isoprene, a hemiterpene, emitted by plants has environmental effects in creation of ozone molecules and growth of aerosols.Citation7 It is well documented that heat stress in plants stimulate a cascade of biochemical reactions leading to ‘fright or flight’ mechanism, i.e., enhanced thermotolerance or succumb to heat stress (Reviewed by Wahid et al., 2007).Citation8 In this study, we attempt a comprehensive study on biochemical responses of Hymenaea plant at biomolecular level. Here, we report a number of proteins that are differentially expressed during heat stress in Hymenaea plants. Moreover, we have also performed an analysis on total lipids and volatiles produced by this tree under the heat stress condition. The research data produced will help to understand molecular physiology of thermotolerance mechanisms in this economically important, but considerably understudied tree species. The understudied status of this tree is evidenced by PubMed search with the keyword ‘Hymenaea’, which resulted in only 76 hits.
Terpenes have potentials to be used as biofuel precursorsCitation9,10 and in our study we have found out that Hymenaea produces many long chain sesquiterpene hydrocarbons. Nobel laureate Dr. Melvin Calvin while working on ‘diesel tree’ (Copaifera sp.)Citation11 reported that this tree produces sesquiterpenes, which could be used as diesel fuel in automobiles without any filtration. Meylemans et al. (2012)Citation12 produced high-density renewable fuel by dimerization of crude turpentines and other chemical compounds. Hymenaea is known as ‘kerosene tree’ for its ability to produce fuel-like compounds.Citation13 We think that this tree may have potentials to be used as a future biodiesel-producing tree because of diversity of terpenes produced by this tree, and thus new knowledge generated via this study is of great interest. However, further research is needed to justify the biofuel potentiality of this tree.
Results
Volatiles study
Three different peaks were seen in case of heat stressed plant samples at retention time (RT) of 1.62, 2.32 and 5.29 min respectively (). The first, second and third peaks have shown a 95%, 90% and 92% similarity match with isoprene, 2-Methylbutanenitrile and β-ocimene respectively to NIST/EPA/NIH Mass Spectral Library (Data version: NIST 11, Software version 2.0). While in case of control plant only one peak at RT of 1.62 min has occurred (). This experiment was performed with triplicate of biological samples and all triplicates in both control and heat stressed have shown the first peak but peaks in heat stressed samples were significantly higher than in controls one. The second peak was observed only in 2 out of 3 heat stressed plant samples and the third peak occurred in all heat stressed plant samples but neither second, nor third peak was observed in the control plant samples.
Figure 1. Showing a comparison of volatiles production from control (black) and heat stressed (red). Peaks 1, 2, 3 are isoprene, 2-methyl butanenitrile and β-Ocimene respectively as compared with >90% similarity match to NIST/EPA/NIH Mass Spectral Library, Data version: NIST 11, Software version 2.0. Above peaks were confirmed with 3 biological samples.
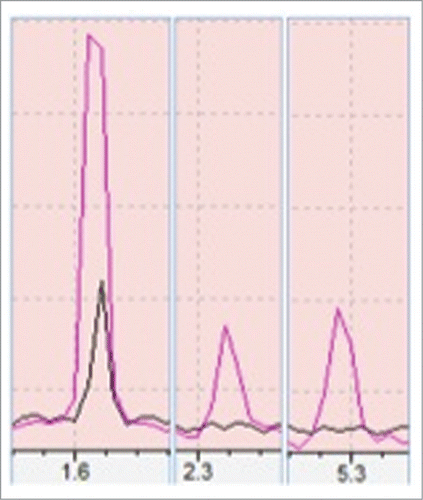
Lipid analysis
Multiple peaks for total lipid analysis from both control and heat stressed plant were observed. Most of these peaks were found to be sesquiterpenes with few diterpenes in both cases except for a phenol peak at RT of 4.60 min. This peak was only observed in total lipid extract of control plant (). Although, the compounds were same, their production was found to be differential in both cases. Sesqueterpens, α-cubebene, copaene, germacrene D, gamma-elemene, cadina-1(10), 4-diene and germacrene-D-4-ol which appear at RT of 8.45, 8.76 9.77, 9.90, 10.02, 10.58 min respectively () found to be produced in significantly higher amount in heat stressed than control plant. Other sesquiterpenes like β-elemene, caryophyllene, α-guaiene, humulene and α-Selinene which appear at RT of 8.85, 9.22, 9.28, 9.55 and 9.88 min respectively () were found to be same in both conditions. An α-gurjunene peak at RT 9.10 minute was found to be reduced in heat stressed conditions. Two peaks at RT of 12.28 and 12.59 min (), which found to be phytol acetate and phytol were produced in higher amount in heat stressed than control plant. A peak for octacosane was also observed at RT of 17.32 min (), production of which found to be same in both case.
Figure 2. Showing comparison of peaks produced by total lipid extracted from control (black) and heat stressed plant (red) through GC-MS. Only the peaks having >90% similarity with the GC-MS NIST/EPA/NIH Mass Spectral Library, Data version: NIST 11, Software version 2.0 were shown here. In the above figure each part is with different magnification to clearly show the differences. The peaks 1 to 16 are phenol, α- Cubebene, Copaene, β-Elemene, α-Gurjunene, Caryophyllene, α-Guaiene, Humulene, Germacrene D, α-Selinene, gamma-Elemene, Cadina-1(10),4-diene, Germacrene-D-4-ol, Phytol acetate, Phytol and Octacosane. The differences in peaks were inferred with triplicate of technical replications.
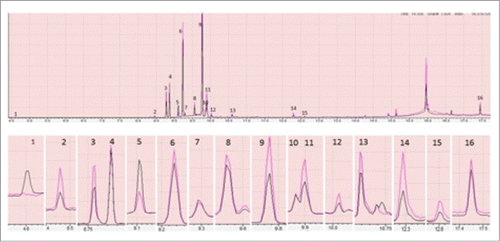
Protein analysis
Differential protein expressions between control and heat stressed leaf tissue were examined based on 2D-DIGE analysis. Based on this in-gel analysis, a total of 151 spots were identified as differential expressed (). All the spots were put for student's t-test, and based on significance (p-value, see supplemental information) 57 spots were selected for protein identification via MALDI-TOF (). These 57 identified proteins were further evaluated by GO annotation using Uniprot and NCBI databases, and were categorized according to the various metabolic processes that they are predicted to be involved in ().
Table 1. Volatile compounds produced by heat stressed (HS) Hymenaea plants
Table 2. Lipid analysis of heat stressed (HS) Hymenaea plant as identified by GC MS
Table 3. Differentially expressed proteins in Hymenaea plants after heat stress as identified by 2-D gel electrophoresis
Figure 3. 2D-DIGE analysis of Hymenaea leaf proteome. Protein samples of Hymenaea control plants and heat stressed plants were differentially labeled with Cy3 (green) and Cy5 (red) respectively. After mixing the 2 labeled proteins in equal ratios, they were first subjected to isoelectric focusing on a IPG strip, pH 3–10, and then on a 12.5% SDS-PAGE. The isoelectric point (pI) and molecular mass (kDa) are marked. Color coding: green spots indicates that the protein abundance is high in Cy3 (control), red spot indicates that the protein abundance is high in Cy5 (heat stressed) samples; yellow spots indicates that the protein abundance is similar in both the cases. Protein identifications of selected spots are shown in .
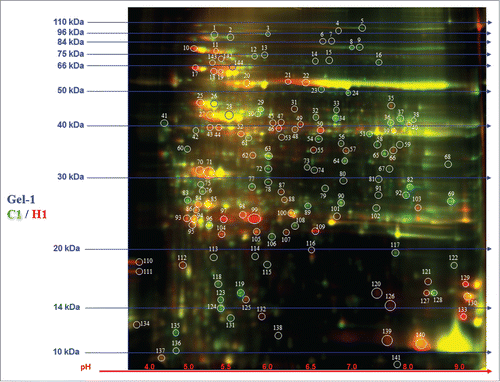
Discussion
Volatile compounds emission analysis from Hymenaea leaves
There are more than 1700 volatile compounds produced by more than 90 plant families.Citation14 Our assay with GC-MS for volatiles emitted from the leaves yielded 3 compounds namely, isoprene, 2-methyl butanenitrile, and β ocimene (). Isoprene emission is directly correlated with thermotolerance in plantsCitation7 and this plays an important role in protecting photosynthetic units in plants during heat stress.Citation7 2-methyl butanenitrile was reported to be present in the volatile compounds produced by lima beans infested with spider mites.Citation15 Also, Jardine et al. in 2010 reported production of 2-methyl butanenitrile from leaves of creosotebush (Larrea tridentata) under various environmental conditions.Citation16 Another compound, which was produced differentially in heat stressed leaf sample, was β ocimene, a monoterpene. Dudareva et al., 2003 reported β ocimene as floral scent produced by snapdragon flowers.Citation17 Beta ocimene is also produced by plants when attacked by insectsCitation18. We propose that production of 2-methyl butanenitrile and β ocimene was a mode of adaptation to abiotic stress. Production of β ocimene and 2-methyl butanenitrile can be justified as a method of thermotolerance.
Total lipid analysis: terpenes identified in heat stressed leaves
Total lipid extractions from heat stressed and control plant leaves yielded terpenes and sesquiterpenes ( and ). It has been reported that terpenes may protect plants against herbivory and insect attack.Citation19 Isoprenes, a hemiterepene also play important roles in protecting plants from heat stressCitation20,21 and water stress.Citation22 Tingey et al. (1991)Citation23 reported high rate of monoterpene emission from plant leaves at elevated temperatures. Loreto et al. (1998) reported Quercus ilex L. emitted high amount of monoterpenes under heat stress.Citation24 They also showed Quercus ilex L. acquired increased thermotolerance, when the plants were fumigated with monoterpenes. Pateraki and Kanellis (2010)Citation25 studied several terpene biosynthetic genes in Cistus creticus subsp creticus and concluded that these genes are upregulated in abiotic stress situation like heat, drought, mechanical wounding. Our finding of abundance of sesquiterpene production in heat stress situation concurs with previous observations by other scientists and will help us to understand mechanism of thermotolerance in Hymenaea tree.
Proteins identified from 2-D gel electrophoresis
Heat shock proteins
Heat shock proteins (HSPs) are produced in plants including all other life forms in response to heat stress. They play important roles in improving thermotolerance in plants (reviewCitation8). Transgenic plants overexpressing HSPs were successfully produced for improved thermotolerance (reviewCitation26). We have observed () a stromal HSP differentially expressed in heat stressed Hymenaea leaves.
Histone proteins
Two histone-like-proteins (H3 and H2B) were found upregulated more than 19 times in heat stressed compared to control plant (). Histones have been reported to be involved in heat sensing in yeast cells and in Arabidopsis thaliana.Citation27,28 Kumar and Wigge (2010) showed that in Arabidopsis thaliana cells, alternative histone H2A.Z was essential in accurate sensing of ambient temperatureCitation29. They have also shown H2A.Z played an important role in DNA unwrapping mechanism, which ultimately lead to heat sensingCitation29. In essence, warm temperature is directly associated with decrease in overall histone protein content. Weng et al. (2014) showed an interesting result, where, they showed histone and chaperone proteins can stimulate acetylation of histone H3K56 in Arabidopsis under heat stress.Citation30 Lang-Mladek et al., 2010 reported enhancement of heat-stress mediated histone acetylation events in plants.Citation31 So, there is a possibility that heat stressed Hymenaea cells upregulated histone acetylation process to protect DNA from damage. Stress-induced histone modification is a complex phenomenon as reviewed by Chinnusami and Zhu (2009).Citation32 Some histone modification including acetylation, phosphorylation and ubiquitination can enhance transcription,Citation33,34 whereas biotinylation and sumoylation may suppress histone gene expression.Citation34 So, there may be 3 explanations of enhanced production of histone proteins in Hymenaea cells: (1) heat stress induced histone chaperone proteins in cells, which in turn produced H3 and H2B at an increased rate, (2) histone acetylation is directly related to DNA repair in plantsCitation35 and (3) there may be acetylation, phosphorylation or ubiquitination of histones in Hymenaea cells and hence there were surge of histone proteins production.
Oxygen evolving complex and other photosynthetic proteins
Oxygen evolving enhancer protein (OEC) is an important protein in photosysytem II (PSII) of plants involved in photo-oxidation of water. Heat stress may rapidly inactivate PSII complexCitation36 along with OEC.Citation37 Mathur et al. (2011) reported a higher temperature lead to irreversible damage of OEC in wheat (Triticum aestivum).Citation38 Heat stress also induces light harvesting complex (LSII) to aggregate to protect plants from damaging effects of heat stress.Citation39 However, Heckathorn et al., 1998 reported that OEC production may decrease within first 24 hours of heat stress, followed by a sharp increase in continued heat stress.Citation40 This increased expression of OEC may be needed to repair damaged PSII proteins.Citation41 We observed OEC was upregulated 26 to 29 folds and downregulated 2 folds compared to control plant (). We speculate that OEC was dissociated following heat stress and then the cells upregulated OEC production to repair damaged PSII systems.
Heat stress directly impacts rate of photosynthesis in plants (reviewed by Wahid et al. 2007). Toth et al. (2005) showed heat stress can damage PSII units in barley leaves.Citation42 However de novo synthesis of PSII can be accounted for gradual improvement of photosynthetic activities following heat stress.Citation8 When spinach leaves were exposed to 40°C, electron flow through PSII was inhibited, but, activated cyclic electron transport in photosystem I (PSI).Citation43 Vasilikiotis and Melis (1994) showed PSII: PSI ratio increased from 1.4:1 to 15:1 when Dunaliella salina cells were transferred from low to high irradiance.Citation44 Accumulation of PSII following heat stress was proposed to play important roles in repairing and replacement of PSII.Citation44 It must be noted here that RuBP (ribulose 1,5-bisphosphate) and PSII reaction centers do not decreases with elevated levels of heat stress, although net photosynthesis is inhibitory at that temperature.Citation45 It has also been reported that RuBP concentration and electron transport may even increase following moderate heat stress in cotton (Gossypium hirsutum).Citation46 They concluded inhibition of photosynthesis in higher temperature may be caused by Rubisco inactivation under heat stress, and at the same time RuBP regeneration was observed to be increased in heat stressed plants. We observed activation of several photosynthesis related proteins (PSI reaction center subunit II, Rubisco) and at the same time we observed suppression of several similar proteins (Ferredoxin-NADP reducatse, Rubisco) ().
Glutamine synthase
El-Khatib et al (2004) showed that expression of a cytosolic glutamine synthetase (GS1) gene in poplar has improved tolerance to dehydaration.Citation47 Hoshida et al (2000) reported that overexpression of a chloroplast glutamine synthetase gene in rice has improved its tolerance to salinity.Citation48 Glutamine synthase protects plants from photo-oxidation under heat stress, resulting in increased photorespiration (as reported in tobaccoCitation49). In this study, we observed that glutamine synthase was upregulated 14-fold in Hymenaea leaves during heat stress. We propose this enzyme plays an important role in protecting plants from various types of abiotic stresses including heat stress.
ATP synthase
ATP synthesis is critical for cells to survive in a stressful situation. However, increased ATP/ADP ratio was observed in spinach leaves, mainly due to decrease in demand for ATP in heat stressed leaves.Citation50 We observed ATP synthase was upregulated in heat stressed plants 5.54, 4.90, 3.70 and 3.65 folds compared to control plant leaves (). Crafts-Bradner and Law (2000) proposed that higher temperature inhibited Rubisco activation (not by suppressing RuBP regeneration) and we propose Rubisco inactivation suppressed Calvin cycle thereby creating less demand for ATP. Hence, we hypothesize that the fluctuations in ATP synthase is probably due to variations in demands of ATP as described above.
Others proteins
A putative protein phosphatase 2C (PP2C) was found to be overexpressed (5.09 folds) in heat stressed Hymenaea plant (). Rodriguez in 1998 had shown that PP2C, which function as a down regulator of mitogen-activated protein kinase (MAPK) pathway, play roles in various signal transductions pathway in alfalfa.Citation51 We speculate PP2C may play an important role as a signal transduction protein during heat stress in Hymenaea.
An isoflavone reductase like enzyme was also found upregulated by more than 5 folds in heat stressed plants. Isoflavone reductase belongs to isoflavonoid biosynthesis pathway. A study has shown that an overexpression of isoflavone reductase-like gene in rice provides a resistance against reactive oxygen species (ROS).Citation52 This upregulation of isoflavone reductase-like gene is also in accordance with the fact that ROS activity generally elevated under different stresses including heat stress as reviewed before.Citation8,26 Our protein analysis also shows an enhanced expression (5.6 times) of Caffeoyl-CoA-O-methyltransferase. Caffeoyl-CoA-O-methyltransferases are enzymes involved in lignin biosynthesis and have shown to overexpress in response to tobacco mosaic virus (TMV) infection.Citation53-55 Our result of higher expression of caffeoyl-CoA-O-methyltransferase, again supports our hypothesis of cross interaction between genes against biotic stress (infection) and thermotolerance.
Materials and Methods
Hymenaea courbaril L. plants () were purchased from Future Forests Nursery, Hawaii, USA. To study the heat stress responses, 18-months-old test plants were grown in a plant growth chamber with diurnal cycle of 12 hours light, and 12 hours dark. The control plants were kept at 25°C, but the heat stressed plants were kept at 40°C for 48 hours with similar photoperiod. The plants and the plant leaves for experiment were selected carefully to minimize any biasness due to size, shape, color and level of maturity.
Volatiles study
To study the volatiles produced by Hymenaea courbaril L. plant, 2 leaflets per plant were excised from 3 separate plants. The leaflets were immediately kept inside airtight glass vials (15 ml volume). The vials were kept at 30° angle and the adaxial surface of the leaf faced the light mimicking natural condition. The control vials were incubated at 25°C while test vials were kept at 40°C for 48 hours in a growth chamber with photoperiod similar to treatments explained above. After 48 hours of incubation, headspace air from each of the controls and heat stressed vials were sampled by an injection, and analyzed by gas chromatography linked to mass spectrometer (GC-MS). A GC-MS (GCMS-QP2010S, SHIMADZU, USA) equipped with a HP-5 column (crosslink 5% PhMe siloxane 30 m X 0.25 μM film thickness) was used in this study for volatiles and total lipids analysis. The following setup was used for GC-MS: an oven temperature from 65°C to 300°C for 18.67 min with a ramping of 15°C /min, with helium carrier gas at a flow rate of 1.2 ml/min and a split ratio of 1:20 (for volatiles) and 1:75 (for lipid). All the peaks in this study were analyzed by its comparison with NIST/EPA/NIH Mass Spectral Library (Data version: NIST 11, Software version 2.0) and only the peaks with more than 90% similarity matches were considered and mass spectra of peaks were compared ().
Lipid analysis
Total lipids were extracted from heat stressed and control plants to study lipids and terpene production. Hymenaea courbaril L plant was heat stressed as described in the above section. About 0.5 g of leaves (from heat stressed and control plants) were excised, mixed with 0.5 g of sands (EMD Chemicals, USA) and ground in liquid N2. Sand was used for fine pulverization of leaf materials for improved lipid yield. The volume of leaf pastes were adjusted to 1 ml with sterile deionized water in glass tubes and total lipids were extracted following the Bligh and Dyer method.Citation56 Equal amount of lipid extracts were collected into Teflon capped glass vials, dried under N2 gas and then dissolved into 500 μl of hexane (Sigma-Aldrich, USA). The extracted total lipids were analyzed by injecting 1 μl of samples into GC-MS.
Protein analysis
Three independent leaf samples were collected from heat stressed and control plants. The leaf tissue grinded in liquid nitrogen from above described experiment was homogenized in 300 μl of lysis buffer (30 mM Tris-HCl, pH 8.8, 7 M urea, 2 M thiourea and 4% CHAPS) under cold conditions. The homogenized sample was subjected to sonication on ice, followed by 30 minutes incubation on a rotary shaker at room temperature. The mixture was centrifuged at 25,000g (4°C) to collect the supernatant. Protein concentration in the supernatant was measured using protein assay kit (BioRad catalog# 500–0006). The lysate samples were diluted with cell lysis buffer described above at 5 μg/μl concentration for downstream experiments. Two-D DIGE and Mass Spectrometric protein identification were run by Applied Biomics (Hayward, CA) using the method essentially as described in Robbins et al. (2013).Citation57 Based on the in-gel analysis and statistical analysis (Table S3), protein spots of interest were picked up by Ettan Spot Picker (GE Healthcare). Individual excised gel spots were washed a couple of times in sterilized HPLC grade water, and in-gel protein digestion was performed with trypsin protease (Promega) using manufacturer's protocol. The digested tryptic peptides were desalted by Zip-tip C18 (Millipore). Peptides were eluted from the Zip-tip with 0.5 μl of matrix solution (cyano-4-hydroxycinnamic acid, 5 mg/ml in 50% acetonitrile, 0.1% trifluoroacetic acid, 25 mM ammonium bicarbonate) and spotted onto the MALDI plate. MALDI-TOF (MS) were performed on a 5800 mass spectrometer (AB Sciex). MALDI-TOF mass spectra were acquired in reflectron positive ion mode, averaging 2000 laser shots per spectrum. TOF/TOF tandem MS fragmentation spectra were acquired for each sample, averaging 2000 laser shots per fragmentation spectrum on each of the 10 most abundant ions present in each sample (excluding trypsin autolytic peptides and other known background ions). Both the resulting peptide mass and the associated fragmentation spectra were submitted to GPS Explorer version 3.5 equipped with MASCOT search engine (Matrix science) to search the UniProtKB_Viridiplantae accessed on March 2014 with 2,127,634 sequences present in the database. Searches were performed without constraining protein molecular weight or isoelectric point, with variable carbamidomethylation of cysteine and oxidation of methionine residues, and with one missed cleavage allowed in the search parameters. Candidates with either protein score C.I.% or Ion C.I.% greater than 95 were considered significant. The 3D-view of all the selected spots (Figure S3), and the protein ID summary (Table S1), and the peptide summaries for all the selected proteins spots (Table S2) is shown as supplemental information.
Conclusion
Hymenaea is one of the most important, but understudied tropical plants. Its importance as plant of medicinal value is well known. This plant is also found to be a rich source of different hydrocarbons and also referred as ‘kerosene tree.’ Some studies have shown that this plant exists at a very low density in the nature.Citation58 Global warming has a negative impact on normal life cycle of all life forms and can further be a factor in declining population of Hymenaea plant because of its low density growth habitat. How a Hymenaea plant responses to heat stress is still unknown. Study on heat stress response of this plant will be helpful in developing a heat tolerant Hymenaea in future. In this study, we have analyzed heat stress response of this plant at volatiles, total lipids and protein levels. We found differential response at these macromolecules under heat stressed condition. We identified several overexpressed proteins and terpenes (like isoprene) under heat stress condition, which are the same ones that have been previously reported to play important roles in plant heat stress sensing and tolerance. This study also showed that a number of different sesquiterpenes are produced by this plant. Interestingly, we have also found some of the terpenoid compounds and proteins involved in insect response in plants are overexpressed under heat stress in Hymenaea. Based upon our result, it is possible that both biotic and abiotic response system might have been working in parallel in Hymenaea plant with a possibility of cross-talk. This work will open future research avenues in the field of understanding molecular physiology or heat stress in plants, especially in tropical tree species.
Disclosure of Potential Conflicts of Interest
No potential conflicts of interest were disclosed.
Supplementary_Table_3.pdf
Download PDF (11.7 KB)Supplementary_Table_2.pdf
Download PDF (3.6 MB)Supplementary_Table_1.pdf
Download PDF (43 KB)Supplementary_Figure_3.pdf
Download PDF (28.2 MB)Supplementary_Figure_2.pdf
Download PDF (3 MB)Supplementary_Figure_1.pdf
Download PDF (8.8 MB)Acknowledgments
CB and DG acknowledge Dr. Michael Summers, CSUN Department of Biology for assistance with lipid extraction and partial student stipend provided to DG through Pamela M Klein, M.D. Scholarship in Biology at CSUN.
Funding
JSR acknowledges financial support from SDSU Agricultural Experiment Station, USDA/SunGrant, and Department of Biology & Microbiology.
Supplemental Material
Supplemental data for this article can be accessed on the publisher's website.
References
- Lee Y, Langenheim JH. Systematics of the Genus Hymenaea L. (Leguminosae, Caesalpinioideae, Detarieae). In: University of California publications in Botany (Vol. 69). Berkeley, CA: University of California Press, 1975.
- Campos MAA, Uchida T. Influência do sombreamento no crescimento de mudas de três espécies amazônicas. Pesquisa Agropecuária Brasileira 2002; 37:281-8; http://dx.doi.org/10.1590/S0100-204X2002000300008
- Cartaxo SL, Souza MM, de Albuquerque UP. Medicinal plants with bioprospecting potential used in semi-arid northeastern Brazil. J Ethnopharmacol 2010; 131:326-42; PMID:20621178; http://dx.doi.org/10.1016/j.jep.2010.07.003
- Carneiro FS, Lacerda AE, Lemes MR, Gribel R, Kanashiro M, Sebbenn AM. Mendelian inheritance, linkage and genotypic disequilibrium in microsatellite loci isolated from Hymenaea courbaril (Leguminosae). Genet Mol Res 2012; 11:1942-8; PMID:22869549; http://dx.doi.org/10.4238/2012.July.19.13
- Fehsenfeld F, Calvert J, Fall R, Goldan P, Guenther AB, Hewitt CN, Lamb B, Liu S, Trainer M, Westberg H, et al. Emissions of volatile organic compounds from vegetation and the implications for atmospheric chemistry. Global Biogeochem Cycles 1992; 6:389-430; http://dx.doi.org/10.1029/92GB02125
- Guenther A, Hewitt CN, Erickson D, Fall R, Geron C, Graedel T, Harley P, Klinger L, Lerdau M, McKay WA, et al. A global model of natural volatile organic compound emissions. J Geophys Res: Atmospheres 1995; 100:8873-92; http://dx.doi.org/10.1029/94JD02950
- Sharkey TD, Wiberley AE, Donohue AR. Isoprene emission from plants: why and how. Ann Bot 2008; 101:5-18; PMID:17921528; http://dx.doi.org/10.1093/aob/mcm240
- Wahid A, Gelani S, Ashraf M, Foolad MR. Heat tolerance in plants: An overview. Environ Exp Bot 2007; 61:199-223; http://dx.doi.org/10.1016/j.envexpbot.2007.05.011
- Rude MA, Schirmer A. New microbial fuels: a biotech perspective. Curr Opin Microbiol 2009; 12:274-81; PMID:19447673; http://dx.doi.org/10.1016/j.mib.2009.04.004
- Harvey BG, Wright ME, Quintana RL. High-density renewable fuels based on the selective dimerization of pinenes. Energy Fuels 2009; 24:267-73; http://dx.doi.org/10.1021/ef900799c
- Calvin M. Hydrocarbons from plants: Analytical methods and observations. Naturwissenschaften 1980; 67:525-33; http://dx.doi.org/10.1007/BF00450661
- Meylemans HA, Quintana RL, Harvey BG. Efficient conversion of pure and mixed terpene feedstocks to high density fuels. Fuel 2012; 97:560-8; http://dx.doi.org/10.1016/j.fuel.2012.01.062
- Janick J, Paull, RE (Editors). The Encyclopedia of Fruit & Nuts. Cambridge, MA: CABI Publishing, 2008.
- Holopainen JK. Can forest trees compensate for stress-generated growth losses by induced production of volatile compounds? Tree Physiol 2011; 31:1356-77; PMID:22112623; http://dx.doi.org/10.1093/treephys/tpr111
- Dicke M, Gols R, Ludeking D, Posthumus M. Jasmonic Acid and Herbivory Differentially Induce Carnivore-Attracting Plant Volatiles in Lima Bean Plants. J Chem Ecol 1999; 25:1907-22; http://dx.doi.org/10.1023/A :1020942102181
- Jardine K, Abrell L, Kurc SA, Huxman T, Ortega J, Guenther A. Volatile organic compound emissions from Larrea tridentata (creosotebush). Atmospheric Chem Phys 2010; 10:12191-206; http://dx.doi.org/10.5194/acp-10-12191-2010
- Dudareva N, Martin D, Kish CM, Kolosova N, Gorenstein N, Fäldt J, Miller B, Bohlmann J. (E)-beta-ocimene and myrcene synthase genes of floral scent biosynthesis in snapdragon: function and expression of three terpene synthase genes of a new terpene synthase subfamily. Plant Cell 2003; 15:1227-41; PMID:12724546; http://dx.doi.org/10.1105/tpc.011015
- Navia-Giné WG, Gomez SK, Yuan J, Chen F, Korth KL. Insect-induced gene expression at the core of volatile terpene release in. Plant Signal Behav 2009; 4:636-8; http://dx.doi.org/10.4161/psb.4.7.8971
- Harborne JB. Recent advances in the ecological chemistry of plant terpenoids. In: Harborne JB, Tomas-Barberan FA, ed. Ecological Chemistry and Biochemistry of Plant Terpenoids Clarendon Press, Oxford, UK., 1991:399-426.
- Loreto F, Sharkey TD. On the relationship between isoprene emission and photosynthetic metabolites under different environmental conditions. Planta 1993; 189:420-4; PMID:24178500; http://dx.doi.org/10.1007/BF00194440
- Munné-Bosch S, Alegre L. Drought-induced changes in the redox state of alpha-tocopherol, ascorbate, and the diterpene carnosic acid in chloroplasts of Labiatae species differing in carnosic acid contents. Plant Physiol 2003; 131:1816-25; http://dx.doi.org/10.1104/pp.102.019265
- Sharkey T, Loreto F. Water stress, temperature, and light effects on the capacity for isoprene emission and photosynthesis of kudzu leaves. Oecologia 1993; 95:328-33; http://dx.doi.org/10.1007/BF00320984
- Tingey DT, Turner DP, Weber JA. Factors controlling the emission of monoterpenes and other volatile organic compounds. In: Sharkey TD, Holland EA, Mooney HA, ed. Trace Gas Emissions by Plants Academic Press, San Diego, CA, USA, 1991:93-119.
- Loreto F, Förster A, Dürr M, Csiky O, Seufert G. On the monoterpene emission under heat stress and on the increased thermotolerance of leaves of Quercus ilex L. fumigated with selected monoterpenes. Plant, Cell Environ 1998; 21:101-7; http://dx.doi.org/10.1046/j.1365-3040.1998.00268.x
- Pateraki I, Kanellis AK. Stress and developmental responses of terpenoid biosynthetic genes in Cistus creticus subsp. creticus. Plant Cell Rep 2010; 29:629-41; PMID:20364257; http://dx.doi.org/10.1007/s00299-010-0849-1
- Bokszczanin KL, Fragkostefanakis S, Consortium SPTITNS-I. Perspectives on deciphering mechanisms underlying plant heat stress response and thermotolerance. Front Plant Sci 2013; 4:315; PMID:23986766; http://dx.doi.org/10.3389/fpls.2013.00315
- Weng M, Yang YUE, Feng H, Pan Z, Shen W-H, Zhu YAN, Dong A. Histone chaperone ASF1 is involved in gene transcription activation in response to heat stress in Arabidopsis thaliana. Plant, Cell Environ 2014: http://dx.doi.org/10.1111/pce.12299
- Erkina TY, Erkine AM. Displacement of histones at promoters of Saccharomyces cerevisiae heat shock genes is differentially associated with histone H3 acetylation. Mol Cell Biol 2006; 26:7587-600; PMID:17015479; http://dx.doi.org/10.1128/MCB.00666-06
- Kumar SV, Wigge PA. H2A.Z-containing nucleosomes mediate the thermosensory response in Arabidopsis. Cell 2010; 140:136-47.
- Weng M, Yang Y, Feng H, Pan Z, Shen WH, Zhu Y, Dong A. Histone chaperone ASF1 is involved in gene transcription activation in response to heat stress in Arabidopsis thaliana. Plant Cell Environ 2014; PMID:24548003
- Lang-Mladek C, Popova O, Kiok K, Berlinger M, Rakic B, Aufsatz W, Jonak C, Hauser MT, Luschnig C. Transgenerational inheritance and resetting of stress-induced loss of epigenetic gene silencing in Arabidopsis. Mol Plant 2010; 3:594-602; PMID:20410255; http://dx.doi.org/10.1093/mp/ssq014
- Chinnusamy V, Zhu, J.K. Epigenetic regulation of stress responses in plants. Curr Opin Plant Biol 2009; 12:133-9; PMID:19179104; http://dx.doi.org/10.1016/j.pbi.2008.12.006
- Sridhar VV, Kapoor A, Zhang K, Zhu J, Zhou T, Hasegawa PM, Bressan RA, Zhu JK. Control of DNA methylation and heterochromatic silencing by histone H2B deubiquitination. Nature 2007; 447:735-8; PMID:17554311; http://dx.doi.org/10.1038/nature05864
- Zhang K, Sridhar VV, Zhu J, Kapoor A, Zhu JK. Distinctive core histone post-translational modification patterns in Arabidopsis thaliana. PLoS One 2007; 2:e1210; PMID:18030344; http://dx.doi.org/10.1371/journal.pone.0001210
- Drury GE, Dowle AA, Ashford DA, Waterworth WM, Thomas J, West CE. Dynamics of plant histone modifications in response to DNA damage. Biochem J 2012; 445:393-401; PMID:22574698; http://dx.doi.org/10.1042/BJ20111956
- Yamane Y, Kashino Y, Koike H, Satoh K. Effects of high temperatures on the photosynthetic systems in spinach: Oxygen-evolving activities, fluorescence characteristics and the denaturation process. Photosynthesis Res 1998; 57:51-9; http://dx.doi.org/10.1023/A :1006019102619
- De Ronde JA, Cress WA, Krüger GH, Strasser RJ, Van Staden J. Photosynthetic response of transgenic soybean plants, containing an Arabidopsis P5CR gene, during heat and drought stress. J Plant Physiol 2004; 161:1211-24; PMID:15602813; http://dx.doi.org/10.1016/j.jplph.2004.01.014
- Mathur S, Mehta P, Jajoo A. Effects of dual stress (high salt and high temperature) on the photochemical efficiency of wheat leaves (Triticum aestivum). Physiol Mol Biol Plants 2013; 19:179-88; PMID:24431485; http://dx.doi.org/10.1007/s12298-012-0151-5
- Tang Y, Wen X, Lu Q, Yang Z, Cheng Z, Lu C. Heat stress induces an aggregation of the light-harvesting complex of photosystem II in spinach plants. Plant Physiol 2007; 143:629-38; PMID:17142484; http://dx.doi.org/10.1104/pp.106.090712
- Heckathorn SA, Coleman JS, Hallberg RL. Recovery of net CO2 assimilation after heat stress is correlated with recovery of oxygen-evolving-complex proteins in Zea mays L. Photosynthetica 1998; 34:13-20; http://dx.doi.org/10.1023/A :1006899314677
- Gazanchian A, Hajheidari M, Sima NK, Salekdeh GH. Proteome response of Elymus elongatum to severe water stress and recovery. J Exp Bot 2007; 58:291-300; PMID:17210992; http://dx.doi.org/10.1093/jxb/erl226
- Tóth SZ, Schansker G, Kissimon J, Kovács L, Garab G, Strasser RJ. Biophysical studies of photosystem II-related recovery processes after a heat pulse in barley seedlings (Hordeum vulgare L.). J Plant Physiol 2005; 162:181-94.
- Bukhov N, Wiese C, Neimanis S, Heber U. Heat sensitivity of chloroplasts and leaves: Leakage of protons from thylakoids and reversible activation of cyclic electron transport. Photosynthesis Res 1999; 59:81-93; http://dx.doi.org/10.1023/A :1006149317411.
- Vasilikiotis C, Melis A. Photosystem II reaction center damage and repair cycle: chloroplast acclimation strategy to irradiance stress. Proc Natl Acad Sci U S A 1994; 91:7222-6; PMID:11607488; http://dx.doi.org/10.1073/pnas.91.15.7222
- Crafts-Brandner SJ, Salvucci ME. Rubisco activase constrains the photosynthetic potential of leaves at high temperature and CO2. Proc Natl Acad Sci U S A 2000; 97:13430-5; PMID:11069297; http://dx.doi.org/10.1073/pnas.230451497
- Crafts-Brandner SJ, Law RD. Effect of heat stress on the inhibition and recovery of the ribulose-1,5-bisphosphate carboxylase/oxygenase activation state. Planta 2000; 212:67-74; PMID:11219585; http://dx.doi.org/10.1007/s004250000364
- El-Khatib RT, Hamerlynck EP, Gallardo F, Kirby EG. Transgenic poplar characterized by ectopic expression of a pine cytosolic glutamine synthetase gene exhibits enhanced tolerance to water stress. Tree Physiology 2004; 24:729-36; PMID:15123444; http://dx.doi.org/10.1093/treephys/24.7.729
- Hoshida H, Tanaka Y, Hibino T, Hayashi Y, Tanaka A, Takabe T, Takabe T. Enhanced tolerance to salt stress in transgenic rice that overexpresses chloroplast glutamine synthetase. Plant Mol Biol 2000; 43:103-11; PMID:10949377; http://dx.doi.org/10.1023/A :1006408712416
- Kozaki A, Takeba G. Photorespiration protects C3 plants from photooxidation. Nature 1996; 384:557-60; http://dx.doi.org/10.1038/384557a0
- Weis E. Reversible heat-inactivation of the calvin cycle: A possible mechanism of the temperature regulation of photosynthesis. Planta 1981; 151:33-9; PMID:24301667; http://dx.doi.org/10.1007/BF00384234
- Rodriguez P. Protein phosphatase 2C (PP2C) function in higher plants. Plant Mol Biol 1998; 38:919-27; PMID:9869399; http://dx.doi.org/10.1023/A :1006054607850
- Kim SG, Kim ST, Wang Y, Kim SK, Lee CH, Kim KK, Kim JK, Lee SY, Kang KY. Overexpression of rice isoflavone reductase-like gene (OsIRL) confers tolerance to reactive oxygen species. Physiol Plant 2010; 138:1-9; PMID:19825006; http://dx.doi.org/10.1111/j.1399-3054.2009.01290.x
- Jaeck E, Dumas B, Geoffroy P, Favet N, Inzé D, Van Montagu M, Fritig B, Legrand M. Regulation of enzymes involved in lignin biosynthesis: induction of O-methyltransferase mRNAs during the hypersensitive reaction of tobacco to tobacco mosaic virus. Mol Plant Microbe Interact 1992; 5:294-300; PMID:1515665; http://dx.doi.org/10.1094/MPMI-5-294
- Pellegrini L, Geoffroy P, Fritig B, Legrand M. Molecular cloning and expression of a new class of ortho-diphenol-O-methyltransferases induced in tobacco (Nicotiana tabacum L.) leaves by infection or elicitor treatment. Plant Physiol 1993; 103:509-17.
- Maury S, Geoffroy P, Legrand M. Tobacco O-methyltransferases involved in phenylpropanoid metabolism. The different caffeoyl-coenzyme A/5-hydroxyferuloyl-coenzyme A 3/5-O-methyltransferase and caffeic acid/5-hydroxyferulic acid 3/5-O-methyltransferase classes have distinct substrate specificities and expression patterns. Plant Physiol 1999; 121:215-24; PMID:10482677; http://dx.doi.org/10.1104/pp.121.1.215
- Bligh EG, Dyer WJ. A rapid method of total lipid extraction and purification. Canadian J Biochem Physiol 1959; 37:911-7; PMID:13671378; http://dx.doi.org/10.1139/o59-099
- Robbins ML, Roy A, Wang P-H, Gaffoor I, Sekhon RS, de O, Buanafina MM, Rohila JS, Chopra S. Comparative proteomics analysis by DIGE and iTRAQ provides insight into the regulation of phenylpropanoids in maize. J Prot 2013; 93:254-75; PMID:23811284; http://dx.doi.org/10.1016/j.jprot.2013.06.018
- Lacerda AEB, Kanashiro M, Sebbenn AM. Effects of selective logging on genetic diversity and spatial genetic structure of a Hymenaea courbaril population in the Brazilian Amazon Forest. Forest Ecol Manag 2008; 255:1034-43.