Abstract
The physical separation of the embryonic regions that give rise to the tissues and organs of multicellular organisms is a fundamental aspect of morphogenesis. Pioneer experiments by Holtfreter had shown that embryonic cells can sort based on “tissue affinities,” which have long been considered to rely on differences in cell-cell adhesion. However, vertebrate embryonic tissues also express a variety of cell surface cues, in particular ephrins and Eph receptors, and there is now firm evidence that these molecules are systematically used to induce local repulsion at contacts between different cell types, efficiently preventing mixing of adjacent cell populations.
Introduction
The transformation of the embryo from an unstructured ball of cells into a well-organized, multilayered structure proceeds by successive patterning events that dictate specific fates to progressively smaller regions. This increasingly refined pattern of subdivisions is stabilized by boundaries which delimit the various cell populations and restrict cell movements by division or migration.Citation1-4 Such boundaries are essential for the development and functioning of the animal organism and are therefore ubiquitous in metazoans.
Two basic types of boundaries can be distinguished already in the simple cnidarians, Hydra: the ectoderm layer and the endodermal sheet are separated by an extracellular matrix-filled space, and morphologically less conspicuous transversal boundaries partition the plane of each cell layer and divide the body into head and foot regions.Citation5 We will refer to the latter type as sorting boundaries, and the former as cleft-like boundaries which start as dynamic gaps between cell populations and often develop into ECM-filled spaces that ensure permanent tissue separation.Citation1
At sorting boundaries, cells of adjacent populations are in seamless contact, yet movement across the interface is prevented. Often, this kind of boundary becomes obvious only in experiments which show, for example, the restriction of cell dispersal. A putative mechanism depends on the remarkable property of embryonic cells to recognize and associate preferentially with cells of the same type in a homotypic interaction. The concept goes back to seminal work by Holtfreter. He showed that when cells dissociated from amphibian embryonic tissues were mixed, they formed a single aggregate, but then sorted into separate populations and eventually differentiated into structures that closely resembled the organs formed during normal development. This indicated that all cells could adhere to each other, yet associated with different affinities.Citation6-8
In the absence of molecular data, the first mechanistic explanation of this behavior was inspired by the observation that cell populations sorted into configurations resembling those of immiscible liquids. By analogy, SteinbergCitation9,10 proposed in his “differential adhesion hypothesis” (DAH) that differences in adhesive strengths could account for the sorting of cell populations, and that adhesive strenght was in turn proportional to adhesion molecule density on the cell surface Citation11 (). Cells would tend to maximize adhesive contacts, which generates tissue surface tension and causes the rounding up of cell aggregates. It leads to the preferential association of similarly adhesive cells and hence boundary formation, and places more adhesive cell populations inside less adhesive ones ().
Figure 1. Models for boundary formation. (A) Classical models for cell sorting and tissue separation based on differences in cell-cell adhesion and cortical contractility. Sorting may be achieved (A) by cell-type specific expression of different cell adhesion molecules (CAMs) with stronger affinity for homotypic binding than for heterotypic interaction, or (A’) by differences in adhesive strength between cell types (differential adhesion hypothesis, or DAH), resulting for instance from different levels of adhesion molecules. (A”) Difference in cell cortex contractility can also lead to cell sorting. Recently, adhesion and contractility have been integrated into a single description of adhesive cell interactions, but for simplicity of depiction they are shown in separate panels here. B. Cell sorting/tissue separation may also be produced by the complementary expression of repellent cell surface cues, which would trigger local cortex contraction and cell repulsion at heterotypic contacts, a process termed contact inhibition.
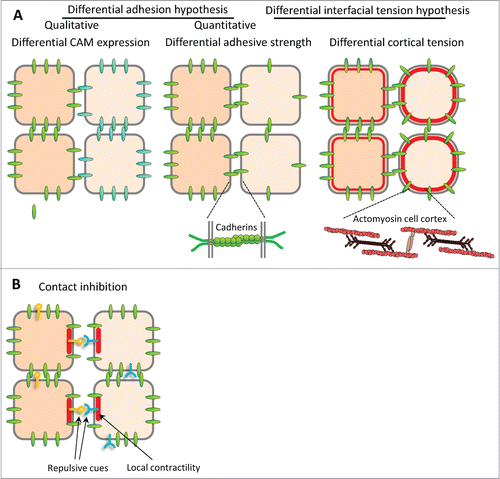
The discovery of a variety of cell-cell adhesion molecules (CAMs), most of which were expressed in tissue specific patterns, and the notion that most CAMs preferred homophilic binding, provided a different explanation for boundary formation: Differential CAM expression would promote cohesion within each tissue, but not between tissues endowed with different CAMsCitation12,13 (). This model, which provided a molecular basis for Holtfreter's “affinities,” was intuitively obvious and became rapidly popular. However, experimental evidence in its favor remained scarce, and the selectivity of homophilic CAM binding came under questioning, at least for classical cadherins (e.g. E-cadherin, or cadherin 1, and N-cadherin, or cadherin 2.Citation14,15 Quantitative measurements of cadherin-cadherin interactions showed surprisingly minor differences between homo- and heterophilic binding, and respective cells failed to sort.Citation16 Even more disturbing was the finding that the binding energy of cadherins is generally too low to quantitatively account for the strength of cell-cell adhesion.Citation17,18 This shifted attention to the role of a cell's cortical cytoskeleton in adhesion. Previously invoked by HarrisCitation19 to explain cell sorting, cortical tension was integrated into the DAH in the Differential Interfacial Tension Hypothesis (DITH) to explain cell sortingCitation20-22 to clarify the relationship between tissue surface tension and cell adhesion ().
This extended concept of differential adhesion was successfully applied to cell sorting in the Zebrafish embryo.Citation23 However, when adhesion is experimentally manipulated to generate highly adhesive cell populations with high surface tension, and low adhesion, low surface tension populations, sorting occurs in vitro but boundaries are fuzzyCitation11,24,25 and in the intact embryo sorting can even be completely absent.Citation25 Apparently, the DAH in its various forms is not generally sufficient for the establishment of distinct sorting boundaries, and other factors may be required in addition or instead.
In fact, compartment boundaries in insect embryos provide an example of a mechanism that prevents the mixing of cells which are in intimate contact. Here, tension in the cortical actomyosin cytoskeleton is increased locally at the interface between cells on one side of the boundary and directly adjacent cells on the other side. This elevated tension tends to straighten the boundary and thus prevents cells from moving across.Citation2,26 The presence of a row of specialized boundary cells is consistent with both mechanisms of sorting boundary formation.
Sorting boundaries can keep cells of different fate from intermixing until tissues become actually separated by more distinct boundaries, characterized by a histologically defined cleft between cell populations. Cell contact across the cleft may be maintained, but then it has to be dynamic, with cells fluctuating between adhesion and detachment. In contrast to sorting boundaries where cells are firmly attached, cleft-like boundaries permit the movement of tissue primordia past each other, for example during massive cell migrations such as in vertebrate gastrulation.Citation27 Eventually, the gaps between cell populations may become filled with an extracellular matrix, e.g., in the form of a basal lamina, to separate tissues permanently.Citation1 It appears that these cleft-like boundaries form initially by a localized process directly at the boundary, the mutual repulsion of cells on either side.
Studies on somite and rhombomere segmentation in various vertebrates implicated ephrins and Eph receptors in the formation of these tissue separation boundaries.Citation28 The membrane-bound ephrin ligand-Eph receptor pairs are prototypic examples of repulsive cues. They were initially mainly characterized for their function in guiding neurons during the complex organization of the nervous system,Citation29 but they are widely expressed beyond the nervous system in embryos and adult organisms.Citation30,31 Their requirement for somite and hindbrain segmentation suggested a completely different mechanism for separation, based on active repulsion at “heterotypic” contacts.Citation28,32-34 We recently demonstrated that ephrins and Eph receptors are also essential for separation of the mesoderm from the ectoderm, and the notochord from the paraxial mesoderm.Citation35,36 At later stages, they are also involved in the individualization of the eye field within the anterior neuroderm.Citation37 It appears that these repulsive cues control virtually all tissue separation events so far investigated in vertebrate embryos, and it is tempting to speculate that these finding reflect a general mechanism of tissue separation based on “negative affinities” that trigger contact inhibition at heterotypic contacts.
In the following sections we have compiled expression and functional data on ephrins and Ephs pertinent to embryonic boundaries in vertebrates. We then discuss aspects of ephrin-Eph signaling that add complexity to these systems, in particular the dual role in repulsion and adhesion, as well as the presence of multiple ephrins and Ephs in embryonic tissues, and how these features may be integrated in what seems to constitute a tissue “identity-code” dictating the response to contact with different neighboring cells. We will also review evidence supporting the existence of additional mechanisms that probably function in parallel to ephrin-Eph signaling to create tissue boundaries.
Expression of Ephrins and Ephs in Early Embryos
Ephrins and Eph receptors were initially characterized in the nervous system, but it was soon realized that their expression is much more widespread, and includes the early embryos of all vertebrates examined so far. The number of different forms expressed in surprisingly high. For instance, in Xenopus gastrula embryos we have detected expression of 7 out of the 8 ephrins and 11 out of the 14 Eph receptors (). Although data for other stages and other species remain incomplete and essentially qualitative, they nevertheless give us an idea of the types of expression patterns present in vertebrate embryos. We present in simplified maps relevant for the 6 boundaries that have been functionally studied: dorsal and ventral ectoderm-mesoderm boundaries,Citation35,38 notochord-presomitic mesoderm,Citation36 hindbrain,Citation33,39,40 somites,Citation32,41,42 and eye field.Citation37 We have selected in each case the pattern from the species where most functional studies were performed. Available data for the hindbrain and somitic mesoderm indicate that these patterns are largely conserved across vertebrates, with some variations though. Unfortunately, the different modes of gastrulation between non-amniotes and amniotes and the insufficient annotation of available expression data make a comparison of the early germ layer expression pattern difficult, and we thus limited the present description to Xenopus. The midbrain-hindbrain boundary, another interesting boundary for which functional? analyses have been published,Citation43 has still not been explored for a role of ephrin/Eph signaling, and available annotation of in situ hybridization images is generally not sufficiently precise to present the relevant pattern of expressions.
Figure 2. Ephrin and Eph receptor expression in the early Xenopus embryo. Transcripts of 7 ephrins and 11 Eph receptors are detected in the early Xenopus stages. The color code symbolizes relative mRNA levels, determined by real-time RT-PCR using tissues dissected from late blastula (stage 9.5), early gastrula (stage 10.5) mid gastrula (stage 11.5) and early neurula (stage 14). Gray color indicates that levels were too low and variable to be quantified.
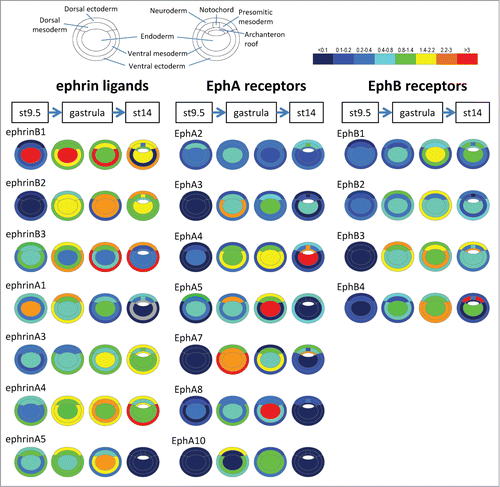
Figure 3. Ephrin and Eph receptor distribution at vertebrate embryonic boundaries. Each diagram represents the pattern observed in the species where most studies were performed: (A) Dorsal ectoderm-mesoderm boundary in the early Xenopus gastrula. (B) Ventral ectoderm-mesoderm boundary in the middle Xenopus gastrula. (C) Notochord-presomitic boundary in the late Xenopus gastrula. (D) Somitogenesis in zebrafish. (E) Hindbrain segmentation in zebrafish. (E’) Comparative diagram from available data in the 4 species, zebrafish (z), Xenopus (x), chicken (c) and mouse (m). Species abbreviated in black denotes expression, in red absence of (or low) expression in the corresponding segment. Absence of symbol indicates that the gene was not investigated in this species. Note that the ephrinB3 gene is altogether absent from chicken. (F) Eye field segregation in zebrafish. In (A–C), the font and box size represent relative enrichments. Comparative data on expression levels are not available for the other systems, but in a few instances lower expression was clearly observed in the hindbrain (ephrinB2 in r1,r3 and r6, EphA4 in r2, EphB4 in r4). Patterns were built based on expression data from:Citation35,36,38,47 for (A–C), Citation32,42,48,52,59,122-125 for (D), Citation33,48,53-56,124,126-128 for (E), and Citation37 for (F), complemented with the Mouse Gene Expression Databases for zebrafish (http://zfin.org) and mouse (http://www.informatics.jax.org/gxd).
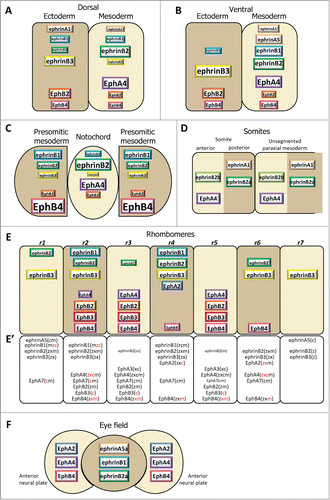
Note that we still do not know precisely the timing of ephrin-Eph activity as related to the initial implementation versus maintenance of tissue separation. In Xenopus, the action of ephrins and Ephs in ectoderm-mesoderm separation appears to be also required throughout most of gastrulation. Similarly, the notochord boundary remains permeable to experimentally induced cell sorting for several hours,Citation36,44 suggesting a long-lasting role for Eph-ephrin signaling in boundary maintenance. In the case of the somites, however, segmentation is coupled to a profound reorganization of tissue organization at the segment boundaries,Citation45,46 and it remains unclear whether cells would still be able to mix at later stages even if Eph-ephrin function would be inhibited. Consistent with a transient role of ephrins and Ephs in the actual process of somite segmentation, some of the expression patterns seem to be specific to the nascent somites, and fade rapidly as somites mature. The timing of hindbrain segment formation and maintenance is also not known. One should note that in situ hybridization images tend to yield variable contrasts, sometimes exaggerating differences between tissues. Thus, an apparently complementary pattern of Ephs and ephrin ligands could mask a lower yet significant expression of components in the adjacent tissue. Keeping these caveats in mind, we have tried to extract from the available data (published articles and zebrafish/mouse expression databases) those patterns that most closely coincide with the stage where each boundary appears.
Ephrin-Eph expression in the gastrula
We have systematically screened all ephrins and Ephs by RT-qPCR on dissected tissues of Xenopus embryos at 4 stages, from late blastula to early neurula. presents the entire collection of patterns, while focuses on the dorsal and ventral ectoderm-mesoderm boundaries and the notochord boundary, and shows only the set of molecules which were functionally tested.Citation35,36,38,47 These studies revealed an astonishing diversity of refined patterns, consistent with a notion that each of the 18 genes detected at these stages is precisely regulated by a complex developmental program. Most show highly dynamic expression, sometimes increasing and/or decreasing dramatically between subsequent stages. Besides germ layer-specific changes, global dorsal or ventral-specific activations or repressions are also apparent, such as the boost of EphA2 on the dorsal side of the early neurula, which transcends germ layers (). None of the components appeared exclusively restricted to a single tissue, although several of them are significantly enriched in one or the other. EphrinB3 is particularly remarkable by its ectoderm enrichment throughout the gastrula and neurula stages (). Beside ectodermal ephrinB3, the dorsal ectoderm-mesoderm boundary, which is the earliest to appear, and the later ventral boundary also share a complementary distribution of EphA4 in the mesoderm and of EphB receptors in the ectoderm, but they differ in ephrin expression ().The patterns corresponding to the separation of axial notochord and paraxial presomitic mesoderm at the end of gastrulation are also interesting (): The notochord largely maintains the typical pattern of the earlier dorsal mesoderm, including a strong enrichment of EphA4 and low levels of ephrinB3 and EphB4. In contrast, the presomitic mesoderm acquires partially complementary features reminiscent of the earlier “ectoderm-specific” pattern.
Available information for other species is currently too fragmentary to reveal generalities of ephrin/Eph expression during gastrulation. However, patterns are in all cases very dynamic. For instance, several ephrins and Ephs (e.g., EphB3 and EphA4) are specifically up-regulated in the primitive streak of the chicken embryo and rapidly down-regulated in the mesoderm after ingression, while ephrinB2, on the contrary, accumulates precisely as cells ingress and remains high in the mesoderm.Citation48
Segmenting paraxial mesoderm
Somitogenesis occurs by progressive segmentation of the paraxial mesoderm in an anterior to posterior sequence. A sophisticated gene regulation circuit determines this process, and segmental expression of several genes prefigures the position of the anterior and posterior extremities of the future somite before physical separation.Citation46,49 Among these the receptor, EphA4, and 2 ephrins, A1 and B2, have been detected in all 4 vertebrate models, in the anterior and posterior halves of somites, respectively (). The detailed patterns are not fully established: in some in situ hybridization images, ephrins and EphA4 appear roughly complementary, while in others they seem to form 2 opposite gradients. These differences may explain the modalities of ephrin-Eph signaling on segmentation (see below). Some of the components are expressed specifically during actual segmentation, for instance EphA4 (see e.g.,Citation48). Others are maintained, to be joined by additional receptors and ligands mostly expressed in specific sub-patterns in more mature somites, where they regulate other processes, such as migrationof neural crest cells and formation of intersomitic veins.Citation50,51 A striking example of a dynamic pattern concerns chicken EphA3, which first accumulates posteriorly in the segmenting somite, then rapidly shifts to an anterior position.Citation48 Note also that in zebrafish, a second ephrinB2, termed B2b, is specifically expressed in the anterior part of the forming somites, in addition to B2a, which is posterior.Citation52 Whether the combined expressions of the 2 forms results in a graded activity remains to be determined.
Hindbrain
Ephrin-Eph expression in the hindbrain has been studied in all 4 vertebrate models, and most features appear highly conserved (). Of the 7 segments, or rhombomeres, the central 3 segments r3-5 show a clear complementary pattern, with r4 expressing high levels of all 3 ephrinB ligands, and r3 and r5 high levels of Eph receptors. This complementarity is absent from the outer segments: the outmost segments r1 and r7 are rich in ephrins, but r2 and r6 express a mixture of the ephrins and Eph receptors which are expressed in adjacent segments. Note that even in r3-5 the complementarity is not absolute, e.g. lower levels of ephrinB2 are also detected in r3. This complex distribution reflects the upstream regulation of hindbrain segmentation by Hox genes. For instance, EphA4 expression in r3 and r5 depends on Krox20, EphA2 in r4 onHoxA1 and HoxB1,Citation53 while the continuous expression of EphA7 in most rhombomeres, but with a sharp r6/7 boundary, is due to repression by HoxB4/D4 in the more anterior segments.Citation54 Thus the overall pattern may be explained by the conjunction of activations and repressions controlled by the multiple HOX genes expressed in overlapping domains.
Evidence for A Role of Ephrins/Eph in Tissue Separation
There is strong evidence for an essential role of ephrin and Eph molecules at all vertebrate boundaries examined so far, with the possible exception of the mouse embryo. We review here the key experimental data gathered in each system (summarized in ). The adopted sequence is historical, starting with the hindbrain and the somites, where a function for ephrins and Ephs was first discovered.
Table 1. Summary of functional results implicating ephrin-Eph signaling in formation of vertebrate boundaries
Hindbrain segmentation
The suggestive alternate expression of EphA4 in odd numbered rhombomeres led Xu et alCitation55 to test its role in hindbrain segmentation. They expressed, both in Xenopus and in zebrafish embryos, a truncated version lacking the cytoplasmic tail (EphA4ΔC), with the rationale that it would sequester endogenous ligands and interfere with normal EphA4 activation. They observed strong disruption of the segmental pattern, providing the first experimental evidence for a role of Eph receptors in boundary formation. Subsequent experiments used mosaic zebrafish embryos produced by cell transplantation to examine the effect of juxtaposing groups of cells with different ephrin/Eph activities. Efficient sorting of cells ectopically expressing ephrins or Ephs was observed. Expression of EphΔC constructs gave similar results, but here the effect was not interpreted as interference with Eph forward signaling as in the original study,Citation55 but rather as activation of ephrin reverse signaling.Citation33 We comment below on the ambiguity in interpreting the effect of these truncated forms. The zebrafish Valentino mutant (homolog to the mouse kreizler gene) provided a proxy to Eph loss-of-function, since this mutation caused loss of EphA4 and EphB4a (and ectopic ephrinB2a expression) specifically in rhombomeres r5 and r6, concomitant with their fusion with adjacent rhombomeres.Citation39,56 Eventually antisense morpholino oligonucleotides, which had just been validated in Xenopus,Citation57 were used to deplete ephrins B2a, ephrin B3 and EphA4.Citation39,40,58 These studies focused largely on r3-5, where thanks to the strong complementarity, the interpretation of the phenotypes was more straightforward.
These series of experiments can be summarized as follows (): Global EphA4 depletion caused partial segmentation defects. By itself, ephrinB2a depletion gave no detectable phenotype, but segmentation was completely disrupted in double ephrinB2/EphA4 depletions.Citation39 In mosaic experiments, forced expression of EphrinB2a, EphA4 or EphB4, caused cells to sort out from those segments where these molecules are normally not expressed and to integrate into the adjacent segments where they are expressed.Citation33,54,56 These results were mirror-imaged in loss-of-function experiments: depleted cells sorted from those rhombomeres that expressed this particular component (r3 and r5 for EphA4MO, r4 for ephrinB2a), but integrated the adjacent rhombomeres where it was naturally absent.
Altogether these data clearly established a role for ephrins and Ephs in cell sorting during hindbrain segmentation. However, these results, despite their apparent coherence, revealed an unexpected puzzle: a simple model based on repulsion between ephrin-expressing and Eph-expressing cells could not explain the observed sorting of the EphA4-depleted cells from the EphA4-expressing rhombomeres. On the contrary, it predicted that EphA4-depleted cells should mix with both populations, creating a continuum between the even and odd segments. To account for this sorting phenotype, the authors postulated a role of EphA4 in intra-segmental cell-cell adhesion,Citation39,40 see below).
Somite segmentation
The role of ephrins and Eph receptors in somitogenes has been mostly studied in zebrafish. Durbin et alCitation32 first showed that widespread ectopic expression of any of the 3 complementarily expressed factors, ephrinA1, ephrinB2 or EphA4, produced a variety of defects ranging from mild deformation of the segments to their almost complete absence. The same effect was observed when expressing soluble secreted forms of these factors, which were considered to act as inhibitors of the endogenous partners. Durbin et alCitation59 went on to show that ephrinB2 and EphA4 expression were under the control of the transcription factor fss, which itself was required for somite segmentation. This mutant was used here similarly as Valentino was in the analysis of hindbrain segmentation: Mosaic embryos were created by grafting groups of fss-/- cells into wild- type embryos or vice versa. EphA4 re-expression in fss-/- cells was sufficient to induce an ectopic boundary, which precisely segregated EphA4-expressing cells, indicating that the process was cell autonomous. A boundary could also be formed between fss-/- cells expressing EphAΔC and wild type cells, or between fss-/- cells expressing full length EphA and wild type cells expressing ephrinB2ΔC.Citation41 Since these 2 truncated variants should not be able to transmit a signal into the expressing cell, but could serve as ligands to activate a cognate partner in the adjacent cells, these data indicated that either EphA4 forward signaling or ephrinB2 reverse signaling were sufficient to induce separation. Similar truncated constructs were more recently tested in the chicken embryo.Citation42 In this case, only EphA4ΔC, but not ephrinB2ΔC, could induce a boundary, and it was concluded that segmentation relied on ephrinB2 reverse signaling (but see below for an alternative explanation).
Ectoderm-mesoderm separation
Ephrin/Eph expression in the Xenopus gastrula is particularly complex and does not show any clear complementarity. Unraveling this system turned out being a daunting task, which required systematic evaluation of each of the ephrins and Ephs by single and multiple depletions, and various combinations of rescues.Citation35,47 Interference with ephrin-Eph signaling caused germ layer fusion in whole embryos. To study their direct involvement in tissue separation, we had to isolate this process from other aspects of gastrulation, in particular mesoderm involution. We thus used mostly an in vitro reconstitution of the ectoderm-mesoderm boundary generated by the apposition of tissue explants.Citation60,61 In this assay, depletion of any of the tested ephrins and Ephs (ephrinA1,B1-3, EphA4,B2,B4Citation35,47) in any of the 2 tissues partially inhibited separation, to a degree that correlated well with the relative abundance of each of these molecules in the targeted tissue. Single, but also multiple depletions in one of the 2 tissues maximally led to roughly 50% inhibition. Stronger inhibition was obtained by depleting Ephs in both tissues, suggesting that signals on both sides of the boundary contributed to separation. Given the multitude of ephrin ligands and Eph receptors expressed, redundancy was surprisingly low, and no case of completely redundant roles was observed.
The use of soluble pre-clustered ephrin or Eph extracellular domains fused to Fc fragments allowed us to directly activate Eph receptors or ephrin ligands, respectively, at the surface of the explants, thus controlling precisely the time and the target of the stimulation. Using this approach, we showed that 2 ectoderm explants could be induced to remain separated by ephrin/Eph stimulation. Strongest separation was obtained with ephrin or Eph isotypes normally enriched in the mesoderm (ephrinB2 and EphA4, ). Conversely, a fragment corresponding to ephrinB3, which is normally enriched in the ectoderm, could induce separation of 2 mesoderm tissues. The same type of gain- and loss-of-function experiments yielded similar results for the ventral ectoderm-mesoderm boundary, demonstrating that despite some differences in the expression pattern, the logic of the system was essentially the same in both cases.Citation47
These data indicated that asymmetrically expressed ephrins and Ephs were interacting across the boundary, dominated by Eph forward signaling in both directions. As mentioned in the context of hindbrain segmentation, ephrins and Eph receptors may also function within tissues, and could indirectly influence separation, for instance by modulating tissue adhesion. To demonstrate that the molecules were directly required to signal across the boundary, we depleted an Eph receptor in one of the tissues, and rescued separation by supplying the missing Eph as a soluble fragment to the surface of the other tissue. This indicates that Eph signaling is required immediately during boundary establishment, and directly at the boundary.
Notochord boundary
The segregation of the notochord in the late Xenopus gastrula also correlates with partial ephrin/Eph enrichments (ephrinB3 and EphB4 in the notochord, ephrinB2 and EphA4 in the presomitic mesoderm, ). The role of these components was addressed by examining sorting of single cells in mosaic embryos. Separation was strongly impaired by depletions of either ephrinB2, ephrinB3, EphA4 or EphB4.Citation36,47 Although each of these molecules was expressed in both tissues, tissue mixing was significant only when the tissue expressing the highest levels was targeted.Citation36
Eye field
Ephrin/Eph depletion in zebrafish also led to eye defects, apparently due to failure of cells of the prospective optic vesicle to sort from the surrounding brain field.Citation37 The process seems to rely on redundant signals (), since double ephrinB1 and B2a depletion produced relatively mild phenotypes, which were made more severe by simultaneous depletion of EphA4a.Citation37
Complications, Caveats, Ambiguities and Discrepancies
In summary, a key role of ephrin-Eph signaling has been firmly established for each of these boundaries. Yet the mechanisms that effectively mediate separation are still not entirely elucidated. The problem is complicated by several factors, including the widespread co-expression of multiple ephrins and Ephs, and the likely existence of parallel, complementary or compensatory mechanisms. A discussion of the unsolved issues necessitates a short review of our current knowledge of some of the peculiarities of ephrins and Eph receptors, in particular their partial selectivity and their ability to promote adhesion under some circumstances ().
Figure 4 (See previous page). Ephrin-Eph signaling in embryonic tissues. (A) Multiple activities and cross-talks. Ephrin-Eph receptor interactions typically activate an intracellular signal that induces reorganization of the actin cytoskeleton, including increased actomyosin contraction, leading to retraction. This repulsive activity is thought to be triggered only at high levels of signal. It requires disruption of the ephrin-Eph bonds which connect the 2 cells. This can occur either by endocytosis, or by shedding of the ephrin/Eph extracellular domains by ADAMs proteinases (not shown). It is believed that, under certain condition, in particular under low ephrin-Eph signaling, ephrin-Eph interactions contribute on the contrary to cell-cell adhesion. Note that the exact mechanisms that control adhesive versus repulsive modes are not well understood. Like many other ligand-receptor systems, ephrin-Eph signaling influences other aspects of cell function, such as gene regulation or cell-matrix adhesion. Ephrins and Eph receptors have been found to directly associate with other cell surface components, such as tight junctions. The effect of these interactions is still poorly understood. When co-expressed in the same cell, ephrins and Eph receptors may also establish cross-talks, which are generally considered to lead to signal dampening. (B–E) Examples of ephrin-Eph configurations (top 2 cell rows) and of predicted consequences of loss-of-function on signaling and adhesion within and between tissues (lower rows). (B’–E’) Resulting effect on tissue cohesion/separation. Sorting/separation is symbolized by a gap between the cells. (B) Embryonic tissues express multiple ephrins and Eph receptors. In the simplest cases, they are expressed in fully complementary patterns, such that signaling is exclusively triggered at the tissue interface, where repulsion antagonizes cadherin cell adhesion, resulting in low effective adhesion. Lowering ephrins or Ephs is predicted to decrease repulsion/increase adhesion at the tissue interface and cause their fusion, without major effect on intratissular contacts. Note that the systematic expression of several ephrins and/or several Ephs observed in all systems creates partial - and sometimes even full - redundancy. As a consequence, more than one of these components may need to be removed to inhibit separation. (C) In more complex cases, expression is only partially complementary. Here ephrins and Eph receptors can also interact in one or both tissues. These intracellular interactions can generate repulsion, which may confer to the tissue different physical properties, e.g., lower rigidity and higher migration. (D) Under certain circumstances, Ephrin-Eph intratissular signaling may generate a pro-adhesive activity, effectively increasing tissue cohesion. In this case, ephrin/Eph depletion is predicted to decrease tissue cohesion, and could even in principle result in artificial sorting from the tissue of origin, while concomitant inhibition of repulsion across the boundary could lead the depleted cells to join the opposite tissue. (E) In a situation where intratissular signaling is weakly repulsive (C), partial loss-of-function may switch from repulsive to adhesive mode. Depleted cells may sort into a compact independent population.
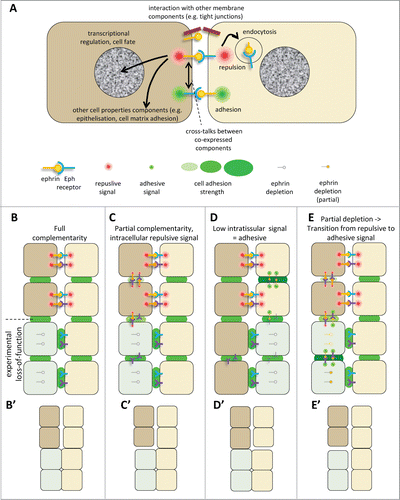
Ephrin-Eph affinities and selectivities
Determining the affinity of ephrin-Eph interactions is a difficult task that has not yet been fully solved. Dealing with membrane-bound proteins whose diffusion is restricted to a 2D layer, and which form clusters is conceptually and technically extremely complex. The affinity values published so far were obtained using soluble recombinant proteins, either as monomers or as preclustered complexes.Citation62-64 Despite the problematic physiological validity of these values, and a relatively large variability between different studies, it can be concluded with good confidence that interactions are far less promiscuous than generally assumed (). For instance, of the 3 ephrinBs, ephrinB2 is the only one which can bind all EphBs and EphA4. EphrinB1 only binds a subset of EphBs and not EphA4, ephrinB3 only EphA4 and EphB3.Citation62,65 In many but not all cases, pairs showing a high affinity in vitro were also identified as interaction partners based on biological functions.Citation62,66 However, the biological relevance of several high affinity ephrin-Eph pairs has yet to be determined. EphrinB3 and EphB3 is one example.Citation62,66,67
Table 2. Preferred ephrin-Eph pairs. A. Pairs formed by ephrinB ligands. B. Pairs formed by ephrinA ligands. In vitro: Summary of measurements of interactions between purified recombinant extracellular domains.Citation62,65,67,111-121 The symbolized strengths of the interactions are symbolized by ++ (strong, Kd < 2 nM), + (medium, Kd ∼2–10 nM), weak (+/−, >10nm) and – (not detected). Note that affinities varied significantly between reports. +(−) and ++(−) indicate contradictory data. ND, not determined. *: EphrinAs were reputed not to bind EphBs. Strong interaction was reported byCitation65 for ephrinA4 with EphB1-3 However, interaction for other ephrinAs was only determined with EphB2. Embryo: Functional assays for ephrinB1-3 and cognate receptors using stimulation of Xenopus tissues with soluble preclustered ephrin/Eph fragments.Citation47
In addition to selective pairing, there is also evidence that different Eph receptors can trigger downstream signaling responses that are not only quantitatively but also qualitatively different.Citation68 In the case of ectoderm-mesoderm separation, however, the cytoplasmic tails of EphA4 and EphB4 can be swapped without influencing activity.Citation47 Again, such studies are still rare and incomplete, and a general perspective is currently missing.
Dual ephrin-Eph functions: Repulsion vs. adhesion
While ephrin-Eph signaling is typically considered to act as a repellent, there is a body of evidence supporting an opposite role in cell-cell adhesion, at least under certain circumstances.Citation69 The current explanation for this dual activity can be summarized as follows: the interaction of large clusters of ephrins and Ephs, binding with high affinity (estimated in the low nanomolar range or below) creates a direct physical link between the membranes of the 2 cells, which may represent a significant adhesive force. For de-adhesion, ephrin-Eph bonds must in some way be disrupted or disengaged. This can occur via 2 different mechanisms: endocytosis of the entire ephrin-Eph clusters, into one of the 2 attached cells,Citation70,71 or proteolytic cleavage of ephrins or Ephs by ADAMs.Citation72 It is not clear whether some ephrin-Eph interactions are by nature adhesive, but it seems that the strength of the signal plays an important role in determining whether the reaction will be adhesive or repulsive, with adhesion generally requiring lower signal intensity than repulsion. Consistent with this principle, those mechanisms that down-regulate signaling usually favor adhesion.Citation69,73,74 Several factors could in principle control the strength of the signal, in particular ligand and receptor cell surface densities. As discussed below, this phenomenon may provide an explanation for the observed adhesive activity of ephrins and Ephs in embryonic tissues.
Evidence for an adhesive ephrin/Eph function in embryonic tissues, and its contribution to separation
In a simple system where ephrins would only react with cognate receptors across the boundary, depletion of either component would be expected to create a situation where cells could integrate into either tissue. The behavior of EphA4-depleted cells in mosaic hindbrain was clearly inconsistent with this expectation.Citation39 Depleted and wild type cells did mix well in even-numbered, ephrinB-expressing rhombomeres, but they robustly sorted out from the odd, EphA4-expressing rhombomeres. The authors concluded that EphA4 must play a role independent of ephrinB2, and postulated that it strengthened adhesion between EphA-expressing cells.Citation39 Similar experiments were repeated with ephrinB2- and double ephrinB2-EphA4 depletions.Citation40 Again, ephrinB2-depleted cells sorted from neighboring cells expressing ephrinB2 (r4), and double depleted cells sorted from both even and odd r3-r5 segments. Note that cell adhesion was not directly examined in these studies, but the proposed model appeared reasonable considering evidence in other systems for an adhesive function of ephrins/Ephs.Citation4
We did monitor the impact of ephrin/Eph loss- and gain-of-function on adhesion for Xenopus gastrula cells using the classical reaggregation assay, and observed that either depletion or overexpression decreased reaggregation of ectoderm cells.Citation35 Our data are consistent with ephrin-Eph signaling within the ectoderm being set to low levels that would correspond to a pro-adhesive regime. Ephrin/Eph depletion would eliminate this adhesive component, while their overexpression would boost the signal above the threshold required to move into the repulsive mode. Assuming that ephrin-Eph signals do indeed switch between adhesive and repelling depending on expression levels, one may expect that experimental depletion may also cause a transition to the adhesive mode. This is a possibility that should be kept in mind when interpreting loss-of-function experiments, including those intriguing sorting phenotypes observed upon ephrin/Eph depletion in zebrafish rhombomeres. Examples of situations that may be determined by different ephrin-Eph systems are presented in . One may also postulate additional partners for ephrins/Ephs, which may directly or indirectly control cell-cell adhesion in these tissues.Citation39 There are certainly precedents, including interactions with tight junction proteins, integrins or FGF receptors.Citation75 A physiological role of Eph/ephrin signaling in mediating adhesion and tissue fusion has been demonstrated for example by Dravis et al.Citation76
Incomplete description of expression patterns
These considerations show the wide spectrum of effects that may be produced by ephrins and Ephs, and emphasize the necessity of a precise knowledge of the distribution and levels of expression of each component to predict their activities and interpret gain- and loss-of-function phenotypes. All embryonic tissues seem to express more than one ephrin and/or Eph receptor, and in many cases they co-express both ligands and receptors. Expression patterns have been quite extensive investigated (), yet besides our systematic screen in the early Xenopus stages (), none of the other inventories is exhaustive. Naturally, most attention has been paid to the components that showed remarkable patterns, in particular those with strong complementary expression such as ephrinBs in r4 and EphA4/B4 in r3 and r5 of the hindbrain. While there is no doubt that these molecules have a key role in segmentation at least in non-mammalian vertebrates, one cannot exclude that other, less conspicuous contributions (including widespread EphA7 in most rhombomeres, ’) may need to be taken into account to explain the observed phenotypes. Lower levels of other ephrins/Ephs may for instance be responsible for residual ephrin-Eph signaling in ephrinB2 or EphA4-depleted cells, which could put these cells into an adhesive regime, thus contributing to their artificial segregation ().
Activity of interfering reagents
Ephrin and Eph deletion constructs lacking the cytoplasmic tail have been used for 2 very different purposes: on one hand they should compete with endogenous Eph receptors for ephrin binding, and thus act as dominant negatives. The same constructs, however, are also expected to stimulate ephrin reverse signaling. Interpreting their effect (or lack of effect) should take into consideration these 2 activities. In addition, the specific interactions of these reagents vary with the spectrum of potential partners: for instance, EphA4ΔC could activate both ephrinB2 and B3, and at the same time compete with all potential receptors, i.e. all EphBs for ephrinB2, EphA4 and B3 for ephrinB3. Interpretation may thus be straightforward sometimes (e.g., suggesting dominant negative activity when the construct mimics depletion, as in all our manipulations at the ectoderm-mesoderm boundary Citation35), but other results may be more ambiguous. For instance, the failure of ephrinB2ΔC to induce ectopic separation of chicken somites was interpreted as a requirement for reverse signaling.Citation42 This view is only valid if a single ephrin-Eph signal were involved. Yet, considering the likely scenario where separation requires the combined activity of several ephrins and Eph receptors, activation of reverse signaling may be masked by the simultaneous inhibition of all receptors of ephrinB2 by the overexpressed construct. Thus different levels of expression and the balance between multiple ligands and receptors may favor either the activating or the inhibiting forward or reverse signals, which probably accounts for reported discrepancies.Citation41,42 The broader range of interference of ΔC constructs may also explain why these reagents may be at times more effective than the equivalent depletions (e.g., EphA4,Citation32).
Redundancy
The effect of single gene depletions varies widely between systems: in the early Xenopus ectoderm-mesoderm boundary, each component seemed to be required for complete separation, to a degree roughly proportional to its relative expression level.Citation35,47 Note however that none of the depletions was sufficient to completely block separation. In the hindbrain, ephrinB2 depletion alone gave no phenotypes, but it strongly cooperated with EphA4, indicating that it does play an important role.Citation39 Neither ephrinB2 nor EphA4 depletions appeared sufficient to inhibit somite segmentation.Citation32 Similarly, separation of the eye field could only be inhibited by simultaneous depletion of all 3 ephrinB1-3.Citation37 Altogether, these results support the notion that separation depends in all cases on multiple ephrins and Eph receptors, which all contribute to some degree to a global repulsion signal. Apparent differences in requirement for single or multiple components may be due to the sensitivity to disturbances of each system: in the case of the ectoderm-mesoderm boundary, repulsion seems to occur close to the threshold required to overcome cell-cell adhesion across the boundary, and a relatively small decrease in global repulsive input may be sufficient to cause tissue fusion. For other boundaries, repulsion may be stronger, and separation only be inhibited by more drastic inhibition. It would be interesting to directly compare the 2 opposite forces mediated by cell adhesion and ephrin-induced repulsion in the different systems. Compensation by alternative pathways (see below) could also explain that some boundaries may better resist to ephrin/Eph depletion.
Lack of separation phenotype in the ephrin/Eph-null mice
This leads to the puzzle of mouse development, where tissue separation appears largely unaffected by knock-outs of ephrins and Ephs: In particular, all the above-mentioned boundaries form perfectly even in mice lacking all 3 ephrinBs.Citation77 Similarly, knock out of EphA4, which stands as a major receptor in many ephrin-mediated processes, did not lead to any overt defect in morphogenesis.Citation78 Several explanations can be put forward: a) Boundary formation in mammals may be independent of ephrin/Eph signaling, and rely on a completely different mechanism, which is possible yet perhaps unlikely; b) mammals may use the same mechanisms as other vertebrates, but compensatory mechanisms may be more active; c) The role of ephrins and Ephs in mammalian tissue separation may be even more redundant, with additional ephrins (ephrinAs) and additional receptors being involved. d) Separation may generally use 2 or more independent mechanisms, which would be active in all vertebrates, but with different degrees of redundancy: they may be only partially redundant in lower vertebrates but fully in mammals.
Additional or alternative separation mechanisms
Additional mechanisms can contribute to vertebrate tissue separation besides ephrin-Eph signaling. Several non-ephrin/Eph factors have been identified which control mesodermal separation behavior in the Xenopus gastrula. A main player is protocadherin 8, also termed paraxial protocadherin (PAPC). This transmembrane protein begins to be expressed in the dorsal mesoderm of the early gastrula.Citation79 Shortly afterwards, it becomes depleted from the most dorsal portion of the mesoderm that forms the notochord, but remains expressed in the adjacent paraxial mesoderm.Citation80-82 As this tissue eventually segments into somites, PAPC expression persists in the anterior half of somites, where it is under the control of Meso1/Mesp2, the same transcription factor that controls EphA4. However, it disappears from the posterior half, generating a pattern of alternating stripes (Citation83,84 for Xenopus + fish;Citation85 for mice). Thus, PAPC delimits all the main tissue separation boundaries identified in the early embryo.
Interference with PAPC function inhibited ectoderm-mesoderm separation.Citation79 At this boundary, PAPC acts through its downstream effector ankyrin repeat domain protein 5,Citation86 and together with a Fz7-dependent non-canonical Wnt pathwayCitation87 it promotes separation behavior.Citation79,88 Preliminary evidence suggests that the Fz7/PAPC module acts in parallel to ephrin-Eph signaling (Luu et al. in preparation). The transmembrane protein Ep-CAM is also involved in the formation of the ectoderm-mesoderm boundaryCitation89; its relationship to the Fz7/PAPC pathway and to ephrin-Eph signaling remains to be elucidated.
Interference with PAPC function also affects somitogenesis in the Xenopus embryo.Citation83 Morphogenesis of the notochord in the late gastrula was likewise perturbed by truncated PAPC,Citation81 and we found that PAPC knockdown affected notochord-somite boundary formation in Xenopus and zebrafish (unpublished results). These findings suggest a widespread role for PAPC in tissue separation. It must be noted however, that again, protocadherin 8 null mice are normal.Citation82 The mechanism through which PAPC regulates tissue separation remains unclear. PAPC does not appear to function as an adhesion molecule itselfCitation90,91 but rather as a modulator of the actin cytoskeleton and of cadherin-mediated cell adhesion.Citation79,90-95 It could promote separation by reinforcing cohesion at homotypic contacts, by locally decreasing adhesion at the boundary, or by both mechanisms.
Combinatorial Ephrin-Eph Code and Tissue Affinities
The classical description of ephrin-Eph-mediated repulsion considers a cell expressing an ephrin ligand contacting a cell of a different type expressing a cognate Eph receptor. When transposed to cell populations, such a system has only 2 possible outputs: repulsion at heterotypic contacts, for example at the boundary between 2 populations, and no signal at homotypic contacts within each population. shows, however, that embryonic tissues as a rule express multiple ephrins and Ephs. This expands the range of outputs and makes predictions much more difficult. Still, as long as ephrins are all in one cell type and Eph receptors in the other, the problem can be reduced to the combined contribution of several components, all acting in the same direction. Separation of the eye anlage seems to follow this simple scheme ().Citation37 Hindbrain separation constitutes a similar case for 2 of its boundaries, r3/4 and r4/5 (), although the situation is more complex for the other segments (see below).
On the other extreme of the spectrum, the Xenopus embryonic germ layers display a rich array of ephrins and Eph receptors (). Most strikingly, each tissue expresses both ligands and receptors, which could potentially activate signals indifferently at homo- and heterotypic contacts. Yet overt repulsion is actually observed only at the tissue interface. The key that explains this highly localized reaction despite the multiplicity of components resides in 2 simple properties of the system: 1) the abovementioned restricted selectivity of ephrin-Eph interactions, which allows only a subset of pairs to engage in functionally significant interactions, and which we have functionally verified in embryonic tissues (),Citation47 and 2) the partially complementary expression of some of these pairs ().Citation35,47
Figure 5. Ephrin-Eph code at embryonic boundaries. The same code, based on selective ephrin-Eph pairs, is used in different configurations. In each case, repulsion results strongest at the tissue interface. (A–C) Simplified examples, taking into consideration a subset of ephrins and Eph receptors. A. Full complementary ephrin/Eph expression accounts for separation of the eye field and of the central hindbrain segments. (B) In the early gastrula, significant intracellular signaling occurs in the mesoderm, but strongest repulsion is restricted to the ectoderm-mesoderm contacts. EphrinB3 and EphB4, both enriched in the ectoderm, do not interact. (C) Hypothetical diagram of ephrin-Eph signaling in the somites. Segmentation may involve the combined activation of EphA4 by ephrinB2 and ephrinA1. EphA4 may be expressed in a gradient, with highest levels coinciding with the boundary. The distribution of ephrinB2 remains unclear, but may span the whole segment, thus activating some intratissular signal in the anterior half. (D) Complete description of ephrinB-Eph signaling at the ectoderm-mesoderm boundary. Individual contributions of each functional pair are semi-quantitatively symbolized by red lines of different thicknesses.
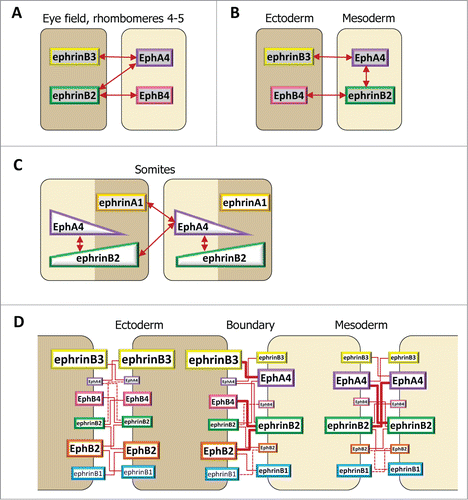
The overall picture can be then summarized as follows: Within each tissue, only a few strong interactions take place, for example mostly ephrinB2-EphA4 in the mesoderm; for the majority of components, the cognate partners are only expressed at low levels (). Thus some ephrins and Ephs can be co-expressed at high levels without much impact, as they largely “ignore” each other (e.g., ephrinB1 and EphA4, both ephrinB1 and B3 and EphB4). Across the boundary, however, the number of strong interactions is significantly higher (). Computing all possible interactions predicted repulsion signals to be strongest at the boundary, intermediate within the mesoderm and weakest within the ectoderm.Citation47 This prediction was fully consistent with a series of experimental data. For instance, 2 ectoderm or 2 mesoderm explants could be artificially separated by supplying one of the explants with an interactor normally enriched in the opposite tissue (e.g., mesoderm-enriched ephrinB2 for ectoderm Citation35,47). Furthermore, as already mentioned, reaggregation assays showed that ephrin/Eph depletion in the mesoderm increased cohesion, indicating the existence of intratissular ephrin-Eph repulsion signals, while it decreased cohesion in the ectoderm, consistent with very low signal intensity that would put the ephrin-Eph system in an adhesive mode. The absence of effective repulsion within the mesoderm could be explained by sub-threshold signal levels insufficient to overcome cell-cell adhesion. This was experimentally verified by decreasing cadherin levels. Under these conditions, strong ephrin-Eph-dependent repulsion within the mesoderm was observed.Citation47
Is the selectivity of ephrin-Eph trans-interactions sufficient to account for separation? Different ephrins and Ephs may activate qualitatively distinct signals, and co-expressed ligands and receptors could establish complex antagonistic cross-talks.Citation31,75,96 We demonstrated, however, that the cytoplasmic tails of EphA4 and EphB4 could be swapped without affecting the ability of these receptors to mediate their function in the mesoderm and the ectoderm, respectively.Citation47 Furthermore, separation was still efficient even when the ephrinB3-EphA4 pair was artificially reconstituted in the opposite direction (ephrinB3 in the mesoderm, EphA4 in the ectoderm). We thus concluded that partial complementary expression of selective ephrin-Eph pairs was sufficient to account for ectoderm-mesoderm separation.
This notion was extended to the ventral ectoderm-mesoderm boundary, where some of the expression patterns are conserved, others distinct from the dorsal boundary (),Citation47 and to the notochord boundary ().Citation36,47 A complete functional description of all these systems would require the additional analysis of ephrinAs and other EphA receptors. EphrinA1 was implicated at the dorsal boundary,Citation38 but it seems that the A families play a less important role, at least during gastrulation (Citation35,47, and unpublished results).
In summary, cells of the early embryo appear to probe contacts with their neighbors based on the array of ephrin-Eph cues expressed at their surface. When this reaction reaches the threshold required to undo adhesive bonds, cells actively repel each other. In principle, the various cell populations may express different, overlapping sets of cues, which could dictate the entire organization of the embryo. Such a mechanism would account for the “tissue affinities” postulated decades ago by Holtfreter.
Why Such Complexity?
The multiplication of ephrins and Ephs and the divergence of their binding selectivity have important implications for vertebrate embryonic development: A system where separation could only function based on the strictly complementary expression of ligands and receptors would be essentially binary. Each boundary would then need to be irreversibly stabilized, for instance by secretion of extracellular matrix, before the next process could take place. How much of early development could be reduced to this binary logic is an interesting question that should be addressed. A more complex set of surface cues may offer much more flexibility, by creating multiple cell surface identities. A new round of separation could then start without waiting for stabilization of the older boundary. It will be interesting, for instance, to determine whether in ephrin-Eph signaling is still required to maintain ectoderm-mesoderm separation at the end of gastrulation, when the dorsal mesoderm splits into notochord and paraxial mesoderm. In principle, simultaneous separation of 3 or more cell populations could even occur.
Alternatively, or in addition, other functions (involution, cell-matrix interactions, ) may necessitate receptor and ligand to be co-expressed within tissues. A network of ephrin-Eph pairs would have then evolved that could accommodate both within-tissue functions and repulsion at boundaries.
Revisiting Other Boundaries Based on The Ephrin-Eph Code
Assuming that the striking selectivity of ephrin-Eph interactions does not depend on the specific cellular context of the Xenopus gastrula, the same principles should be applicable to the other boundaries where multiple ephrins and Ephs are expressed. We comment here on the hindbrain and the somites, as they provide an interesting glimpse into possible variations on the same theme that may all be compatible with tissue separation.
In the hindbrain, a truly complementary pattern is only observed at boundaries r3-4 and r4-5, and published studies have focused on these 2 simple cases. However, these may not represent typical examples: While r1 and r7 resemble r4 in being ephrin-rich, r2 and r6 have mixed expression, sharing ephrins with r1 and r7, and EphB receptors with r3 and r5 (). Note that r3 also expresses some ephrinB2, which could in principle react with the multiple Ephs present within this segment. The puzzling expression of EphBs in both even and odd rhombomeres had led Durbin et al to postulate that r2 and r6 would express a ligand that would be specific for r3/5-enriched EphA4.Citation59 This ligand is almost certainly ephrinB3,Citation97 but this awaits experimental validation. The situation at the first and last boundaries remains unclear, and their resolution may require searching for additional components and/or revisiting the system with more quantitative methods.
The organization of ephrin-Eph signaling in the somite is also puzzling, despite the simple picture commonly presented, which emphasizes the alternate expression of ephrinB2a in the posterior half and EphA4 in the anterior half of each somite. This description poses at least 2 problems: a) It does not explain why separation does not occur as well in the middle of the somite, where ephrinB2a and EphA4 should also be in contact (). Two explanations have been proposedCitation98: 1) The 2 components seem to be distributed in graded manners (), and separation may then occur only at the interface where levels of both ligand and receptor peak. According to this hypothesis, signaling within the segment would always remain below the threshold for effective repulsion, and/or a graded level of repulsive signals would not be sufficient to drive cell sorting within the segment. 2) Additional mechanisms could strengthen cohesion in the middle of the segment, perhaps involving N-cadherin and N-CAM.Citation98 b) In Zebrafish, which is partially tetraploid, there are 2 ephrinB2 “pseudo-alleles.” Only ephrinB2a was studied, which fitted with the classical view of ligand-receptor complementarity. Ephrin B2b, however, is specifically expressed in the anterior half of the somites where it should interact with EphA4 (). The fact that the single ephrinB2 gene of birds and mammals is expressed in a posterior to anterior gradient in the forming somites, similar to zebrafish ephrinB2a, suggests that the contribution of the second zebrafish gene to somite segmentation may be minor and that total ephrinB2 is indeed asymmetrically distributed. However, this remains to be confirmed. In fact, ephrinB2 expression must not determine the site of somite segmentation: EphA4 graded distribution may in principle be sufficient to account for separation even in the presence of a more homogenous ephrinB2 expression. For instance, EphA4 levels could be limiting, and the threshold required for repulsion may only be reached at the peak of expression. Alternatively, ephrinB2 and EphA4 may antagonize each other when co-expressed in the same cell.Citation75 Moreover, posteriorly-enriched ephrinA1 is an obvious additional ligand for EphA4 that should be considered ().
The specific upregulation of ephrinB2b in the somite precisely in the domain of its putative receptor EphA4 is surprising, but probably not fortuitous. Indeed, the ephrinB2-EphA4 pair was consistently found to be systematically co-expressed in the Xenopus gastrula, first in the dorsal mesoderm, then in the ventral mesoderm, and finally in the notochord (). EphrinB2 and EphA4 are also co-expressed in rhombomeres 2 and 6 (). This contrasts with the ephrinB3-EphA4 pair, which is always complementarily expressed. We postulate that this tight correlation reflects a specific intratissular function, perhaps independent of the repulsive functions (). In the Xenopus gastrula, ephrinB2-EphA4 control gene expression in the mesoderm to regulate involution.Citation99
Back to The Models: Global Differences in Tissue Properties Versus Local Discontinuities
In the classical DAH and in its DITH version, the interface generated by the juxtaposition of 2 cell types is typically predicted to display adhesive and contractile strengths intermediate between those of the 2 tissues. In other words, given cell type A with low adhesion and type B with high adhesion, the strength of A-B adhesion should be intermediate between that of A-A and B-B. Thus, the efficiency of sorting will depend on how large the difference is between A-A and B-B adhesions. In a system based on contact-dependent local repulsion, however, conditions at the boundary are controlled by a signal that is specifically triggered at heterotypic contacts (). Although intrinsic tissue properties can certainly affect the degree of adhesion at the boundary, such global differences between the tissues are not required. As long as a sufficiently strong repulsion signal is generated at the interface, separation can occur even between 2 tissues of identical adhesive properties.
Global adhesive and contractile properties of embryonic tissues have been studied in some detail, but whether the often substantial quantitative differences found are sufficient to drive separation remains uncertain. Data on interfacial tension at the boundary or adhesion across the boundary are still scarce (e.g., Citation100,101). Yet a number of observations argue that vertebrate embryonic boundaries are sites of strong discontinuity in tissue properties: they are characterized by intense activation of Eph receptors (zebrafish somite boundary,Citation102 Xenopus ectoderm-mesoderm boundary,Citation47 local RhoGTPase activation (ectoderm-mesoderm,Citation35 rhombomeresCitation58) and prominent accumulation of actomyosin structures (ectoderm-mesoderm and notochord boundaries,Citation36,47 rhombomeresCitation58). The effect on cell adhesion seems to vary between boundaries, probably depending on the intensity of repulsive reactions. At the ectoderm-mesoderm boundary, ephrin-Eph signaling induces cyclic alternations between cell repulsion/detachment and re-attachment.Citation35 At the notochord boundary, adhesion appears to be permanently low or absent, probably due to an extremely high cortical tension that prevents establishment of adhesive bonds.Citation36
In conclusion, the conditions at cleft-like boundaries clearly do not correspond to an intermediate state of adhesive strength as predicted by DAH/DITH. On the contrary they present unique cell behaviors that entail an acute decrease in cell adhesion. Obviously, embryonic development requires fast and pervasive mechanisms for cell segregation that allow for rapid tissue rearrangements. Local cell surface cues such as ephrin and Ephs are ideal to produce this effect.
Ephrins/Ephs in nvertebrates
While ephrins and Eph receptors are best known in mammals for their role in neurogenesis and angiogenesis, this cannot be their original function, since they exist in all metazoans, including those without nervous and blood circulatory systems such as sponges.Citation103-105 Yet such simple organisms are subdivided by boundaries which separate, in cnidarians for instance, the ectoderm from the endoderm and different morphological regions of the organism.Citation5 Experiments on ephrins and Ephs in invertebrates are still lacking, but it is tempting to speculate that ephrin-Eph signaling underlies the very origin of the cell sorting processes that characterize the metazoan organization. Eph receptors can be traced back to pre-metazoan taxa and are found already in sponges,Citation103 and cnidarians express multiple ephrins and Ephs including, quite remarkably, the 3 classical ephrins B1-3, which suggests that the ephrin-Eph code may be already functional in these animals. Insects seem to have lost secondarily all but one ephrin and one Eph receptor. A single pair could still be sufficient for dichotomic separations, e.g. ectoderm-mesoderm separation, but not for more complex processes such as the compartmentalization of the imaginal disc along simultaneous dorso-ventral and an anterior-posterior boundaries. These systems may have exploited other types of repulsive cues, or completely different mechanisms. Echinoid is an example of a surface protein that may function in an analogous way to PAPC,Citation106-108 although it has not been implicated so far at classical compartment boundaries.
Conclusion
Our understanding of tissue separation has evolved from a relatively simple view based on differences in adhesion to a quite different and incomparably more complex picture. Embryonic cells display an astonishing wealth of surface cues that contribute to identify neighboring cells and to activate instructive signals that promote either adhesion or repulsion. Ephrins and Eph receptors certainly play a key role in this process. We are starting to grasp some of the logic of these systems, but considering the multiplicity of these cues and the versatility of their cellular activities, it seems that there is still a whole world of cell-cell contact dependent regulations to be explored.
Disclosure of Potential Conflicts of Interest
No potential conflicts of interest were disclosed.
References
- Tepass U, Godt D, Winklbauer R. Cell sorting in animal development: signalling and adhesive mechanisms in the formation of tissue boundaries. Curr Opin Genet Dev 2002; 12:572-82; PMID:12200163; http://dx.doi.org/10.1016/S0959-437X(02)00342-8
- Dahmann C, Oates AC, Brand M. Boundary formation and maintenance in tissue development. Nat Rev Genet 2011; 12:43-55; PMID:21164524; http://dx.doi.org/10.1038/nrg2902
- Krens SF, Heisenberg CP. Cell sorting in development. Curr Top Dev Biol 2011; 95:189-213; PMID:21501752; http://dx.doi.org/10.1016/B978-0-12-385065-2.00006-2
- Batlle E, Wilkinson DG. Molecular mechanisms of cell segregation and boundary formation in development and tumorigenesis. Cold Spring Harb Perspect Biol 2012; 4:a008227; PMID:22214769; http://dx.doi.org/10.1101/cshperspect.a008227
- Bottger A, Hassel M. Hydra, a model system to trace the emergence of boundaries in developing eumetazoans. Int J Dev Biol 2012; 56:583-91; PMID:22689360; http://dx.doi.org/10.1387/ijdb.113454ab
- Holtfreter J. Gewebsaffinität, ein Mittel der embryonalen Formbildung. Arch Exp Zellforsch Gewebeszücht 1939; 23: 169-209.
- Holtfreter J. A study of the mechanics of gastrulation. J Exp Zool 1944; 95:171-212; http://dx.doi.org/10.1002/jez.1400950203
- Townes PL, Holtfreter J. Directed movements and selective adhesion of embryonic amphibian cells. J Exp Zool 1955; 128:53-120; http://dx.doi.org/10.1002/jez.1401280105
- Steinberg MS. Reconstruction of tissues by dissociated cells. Some morphogenetic tissue movements and the sorting out of embryonic cells may have a common explanation. Science 1963; 141:401-8; PMID:13983728; http://dx.doi.org/10.1126/science.141.3579.401
- Steinberg MS. Does differential adhesion govern self-assembly processes in histogenesis? Equilibrium configurations and the emergence of a hierarchy among populations of embryonic cells. J Exp Zool 1970; 173:395-434; PMID:5429514; http://dx.doi.org/10.1002/jez.1401730406
- Foty RA, Steinberg MS. The differential adhesion hypothesis: a direct evaluation. Dev Biol 2005; 278:255-63; PMID:15649477; http://dx.doi.org/10.1016/j.ydbio.2004.11.012
- Edelman GM. Cell adhesion molecules in the regulation of animal form and tissue pattern. Annu Rev Cell Biol 1986; 2:81-116; PMID:3548776; http://dx.doi.org/10.1146/annurev.cb.02.110186.000501
- Takeichi M. Morphogenetic roles of classic cadherins. [Review]. Curr Op Cell Biol 1995; 7:619-27; PMID:8573335; http://dx.doi.org/10.1016/0955-0674(95)80102-2
- Foty RA, Steinberg MS. Cadherin-mediated cell-cell adhesion and tissue segregation in relation to malignancy. Int J Dev Biol 2004; 48:397-409; PMID:15349815; http://dx.doi.org/10.1387/ijdb.041810rf
- Niessen CM, Gumbiner BM. Cadherin-mediated cell sorting not determined by binding or adhesion specificity. J Cell Biol 2002; 156:389-99; http://dx.doi.org/10.1083/jcb.200108040
- Shi Q, Chien YH, Leckband D. Biophysical properties of cadherin bonds do not predict cell sorting. J Biol Chem 2008; 283:28454-63; PMID:18552401; http://dx.doi.org/10.1074/jbc.M802563200
- Youssef J, Nurse AK, Freund LB, Morgan JR. Quantification of the forces driving self-assembly of three-dimensional microtissues. Proc Natl Acad Sci U S A 2011; 108:6993-8; PMID:21482784; http://dx.doi.org/10.1073/pnas.1102559108
- Maitre JL, Berthoumieux H, Krens SF, Salbreux G, Julicher F, Paluch E, Heisenberg CP. Adhesion Functions in Cell Sorting by Mechanically Coupling the Cortices of Adhering Cells. Science 2012; 338:253-6; PMID:22923438; http://dx.doi.org/10.1126/science.1225399
- Harris AK. Is Cell sorting caused by differences in the work of intercellular adhesion? A critique of the Steinberg hypothesis. J Theor Biol 1976; 61:267-85; PMID:985668; http://dx.doi.org/10.1016/0022-5193(76)90019-9
- Brodland GW. The Differential Interfacial Tension Hypothesis (DITH): a comprehensive theory for the self-rearrangement of embryonic cells and tissues. J Biomech Eng 2002; 124:188-97; PMID:12002128; http://dx.doi.org/10.1115/1.1449491
- Brodland GW, Yang J, Sweny J. Cellular interfacial and surface tensions determined from aggregate compression tests using a finite element model. HFSP J 2009; 3:273-81; PMID:20057960; http://dx.doi.org/10.2976/1.3175812
- Manning BD, Barrett JG, Wallace JA, Granok H, Snyder M. Differential regulation of the Kar3p kinesin-related protein by two associated proteins, Cik1p and Vik1p. J Cell Biol 1999; 144:1219-33; PMID:10087265; http://dx.doi.org/10.1083/jcb.144.6.1219
- Krieg M, Arboleda-Estudillo Y, Puech PH, Kafer J, Graner F, Muller DJ, Heisenberg CP. Tensile forces govern germ-layer organization in zebrafish. Nat Cell Biol 2008; 10:429-36; PMID:18364700; http://dx.doi.org/10.1038/ncb1705
- Steinberg MS, Takeichi M. Experimental specification of cell sorting, tissue spreading, and specific spatial patterning by quantitative differences in cadherin expression. Proc Natl Acad Sci USA 1994; 91:206-9; PMID:8278366; http://dx.doi.org/10.1073/pnas.91.1.206
- Ninomiya H, David R, Damm EW, Fagotto F, Niessen CM, Winklbauer R. Cadherin-dependent differential cell adhesion in Xenopus causes cell sorting in vitro but not in the embryo. J Cell Sci 2012; 125:1877-83; PMID:22328523; http://dx.doi.org/10.1242/jcs.095315
- Landsberg KP, Farhadifar R, Ranft J, Umetsu D, Widmann TJ, Bittig T, Said A, Julicher F, Dahmann C. Increased cell bond tension governs cell sorting at the Drosophila anteroposterior compartment boundary. Curr Biol 2009; 19:1950-5; PMID:19879142; http://dx.doi.org/10.1016/j.cub.2009.10.021
- Winklbauer R. Cell adhesion in amphibian gastrulation. Int Rev Cell Mol Biol 2009; 278:215-75; PMID:19815180; http://dx.doi.org/10.1016/S1937-6448(09)78005-0
- Xu Q, Wilkinson DG. Boundary formation in the development of the vertebrate hindbrain. Wiley Interdisc Rev Dev Biol 2013; 2:735-45; http://dx.doi.org/10.1002/wdev.106
- Flanagan JG, Vanderhaeghen P. The ephrins and Eph receptors in neural development. Annu Rev Neurosci 1998; 21:309-45; PMID:9530499; http://dx.doi.org/10.1146/annurev.neuro.21.1.309
- Klein R. Eph/ephrin signaling in morphogenesis, neural development and plasticity. Curr Op Cell Biol 2004; 16:580-9; PMID:15363810; http://dx.doi.org/10.1016/j.ceb.2004.07.002
- Pasquale EB. Eph receptors and ephrins in cancer: bidirectional signalling and beyond. Nat Rev Cancer 2010; 10:165-80; PMID:20179713; http://dx.doi.org/10.1038/nrc2806
- Durbin L, Brennan C, Shiomi K, Cooke J, Barrios A, Shanmugalingam S, Guthrie B, Lindberg R, Holder N. Eph signaling is required for segmentation and differentiation of the somites. Genes Dev 1998; 12:3096-109; PMID:9765210; http://dx.doi.org/10.1101/gad.12.19.3096
- Xu Q, Mellitzer G, Robinson V, Wilkinson DG. In vivo cell sorting in complementary segmental domains mediated by Eph receptors and ephrins. Nature 1999; 399:267-71; PMID:10353250; http://dx.doi.org/10.1038/20452
- Mellitzer G, Xu Q, Wilkinson D. Eph receptors and ephrins restrict cell intermingling and communication. Nature 1999; 400:77-81; PMID:10403252; http://dx.doi.org/10.1038/21907
- Rohani N, Canty L, Luu O, Fagotto F, Winklbauer R. EphrinB/EphB signaling controls embryonic germ layer separation by contact-induced cell detachment. PLoS Biol 2011; 9:e1000597; PMID:21390298; http://dx.doi.org/10.1371/journal.pbio.1000597
- Fagotto F, Rohani N, Touret AS, Li R. A molecular base for cell sorting at embryonic boundaries: contact inhibition of cadherin adhesion by ephrin/ Eph-dependent contractility. Dev Cell 2013; 27:72-87; PMID:24094740; http://dx.doi.org/10.1016/j.devcel.2013.09.004
- Cavodeassi F, Ivanovitch K, Wilson SW. Eph/Ephrin signalling maintains eye field segregation from adjacent neural plate territories during forebrain morphogenesis. Development 2013; 140:4193-202; PMID:24026122; http://dx.doi.org/10.1242/dev.097048
- Park EC, Cho GS, Kim GH, Choi SC, Han JK. The involvement of Eph-Ephrin signaling in tissue separation and convergence during Xenopus gastrulation movements. Dev Biol 2011; 350:441-50; PMID:21147090; http://dx.doi.org/10.1016/j.ydbio.2010.12.012
- Cooke JE, Kemp HA, Moens CB. EphA4 is required for cell adhesion and rhombomere boundary formation in the zebrafish. Curr Biol 2005; 15:536-42; PMID:15797022; http://dx.doi.org/10.1016/j.cub.2005.02.019
- Kemp HA, Cooke JE, Moens CB. EphA4 and EfnB2a maintain rhombomere coherence by independently regulating intercalation of progenitor cells in the zebrafish neural keel. Dev Biol 2009; 327:313-26; PMID:19135438; http://dx.doi.org/10.1016/j.ydbio.2008.12.010
- Barrios A, Poole RJ, Durbin L, Brennan C, Holder N, Wilson SW. Eph/Ephrin signaling regulates the mesenchymal-to-epithelial transition of the paraxial mesoderm during somite morphogenesis. Curr Biol 2003; 13:1571-82; PMID:13678588; http://dx.doi.org/10.1016/j.cub.2003.08.030
- Watanabe T, Sato Y, Saito D, Tadokoro R, Takahashi Y. EphrinB2 coordinates the formation of a morphological boundary and cell epithelialization during somite segmentation. Proc Natl Acad Sci USA 2009; 106:7467-72; PMID:19380726; http://dx.doi.org/10.1073/pnas.0902859106
- Jungbluth S, Larsen C, Wizenmann A, Lumsden A. Cell mixing between the embryonic midbrain and hindbrain. Curr Biol 2001; 11:204-7; PMID:11231158; http://dx.doi.org/10.1016/S0960-9822(01)00049-5
- Reintsch WE, Habring-Mueller A, Wang RW, Schohl A, Fagotto F. β-Catenin controls cell sorting at the notochord-somite boundary independently of cadherin-mediated adhesion. J Cell Biol 2005; 170:675-86; PMID:16103232; http://dx.doi.org/10.1083/jcb.200503009
- Takahashi Y, Sato Y. Somitogenesis as a model to study the formation of morphological boundaries and cell epithelialization. Dev Growth Differ 2008; 50 Suppl 1:S149-55; PMID:18482400; http://dx.doi.org/10.1111/j.1440-169X.2008.01018.x
- Maroto M, Bone RA, Dale JK. Somitogenesis. Development 2012; 139:2453-6; PMID:22736241; http://dx.doi.org/10.1242/dev.069310
- Rohani N, Parmeggiani A, Winklbauer R, Fagotto F. Variable combinations of specific ephrin ligand/Eph receptor pairs control embryonic tissue separation. PloS Biol 2014; 12: e1001955; http://dx.doi.org/10.1371/journal.pbio.1001955
- Baker RK, Antin PB. Ephs and ephrins during early stages of chick embryogenesis. Dev Dyn 2003; 228:128-42; PMID:12950087; http://dx.doi.org/10.1002/dvdy.10354
- Aulehla A, Pourquie O. Oscillating signaling pathways during embryonic development. Curr Opin Cell Biol 2008; 20:632-7; PMID:18845254; http://dx.doi.org/10.1016/j.ceb.2008.09.002
- Davy A, Soriano P. Ephrin-B2 forward signaling regulates somite patterning and neural crest cell development. Dev Biol 2007; 304:182-93; PMID:17223098; http://dx.doi.org/10.1016/j.ydbio.2006.12.028
- Helbling PM, Saulnier DM, Brandli AW. The receptor tyrosine kinase EphB4 and ephrin-B ligands restrict angiogenic growth of embryonic veins in Xenopus laevis. Development 2000; 127:269-78; PMID:10603345
- Chan J, Mably JD, Serluca FC, Chen JN, Goldstein NB, Thomas MC, Cleary JA, Brennan C, Fishman MC, Roberts TM. Morphogenesis of prechordal plate and notochord requires intact Eph/ephrin B signaling. Dev Biol 2001; 234:470-82; http://dx.doi.org/10.1006/dbio.2001.0281
- Chen J, Ruley HE. An enhancer element in the EphA2 (Eck) gene sufficient for rhombomere-specific expression is activated by HOXA1 and HOXB1 homeobox proteins. J Biol Chem 1998; 273:24670-5; PMID:9733765; http://dx.doi.org/10.1074/jbc.273.38.24670
- Prin F, Serpente P, Itasaki N, Gould AP. Hox proteins drive cell segregation and non-autonomous apical remodelling during hindbrain segmentation. Development 2014; 141:1492-502; PMID:24574009; http://dx.doi.org/10.1242/dev.098954
- Xu Q, Alldus G, Holder N, Wilkinson DG. Expression of truncated Sek-1 receptor tyrosine kinase disrupts the segmental restriction of gene expression in the Xenopus and zebrafish hindbrain. Development 1995; 121:4005-16; PMID:8575301
- Cooke J, Moens C, Roth L, Durbin L, Shiomi K, Brennan C, Kimmel C, Wilson S, Holder N. Eph signalling functions downstream of Val to regulate cell sorting and boundary formation in the caudal hindbrain. Development 2001; 128:571-80; PMID:11171340
- Heasman J. Morpholino oligos: making sense of antisense? Dev Biol 2002; 243:209-14; PMID:11884031; http://dx.doi.org/10.1006/dbio.2001.0565
- Calzolari S, Terriente J, Pujades C. Cell segregation in the vertebrate hindbrain relies on actomyosin cables located at the interhombomeric boundaries. EMBO J 2014; 33:686-701; PMID:24569501; http://dx.doi.org/10.1002/embj.201386003
- Durbin L, Sordino P, Barrios A, Gering M, Thisse C, Thisse B, Brennan C, Green A, Wilson S, Holder N. Anteroposterior patterning is required within segments for somite boundary formation in developing zebrafish. Development 2000; 127:1703-13; PMID:10725246
- Wacker S, Grimm K, Joos T, Winklbauer R. Development and Control of Tissue Separation at Gastrulation in Xenopus. Dev Biol 2000; 224:428-39; PMID:10926778; http://dx.doi.org/10.1006/dbio.2000.9794
- Winklbauer R, Luu O. Frizzled-7-dependent tissue separation in the Xenopus gastrula. Methods Mol Biol 2008; 469:485-92; PMID:19109728; http://dx.doi.org/10.1007/978-1-60327-469-2_31
- Blits-Huizinga CT, Nelersa CM, Malhotra A, Liebl DJ. Ephrins and their receptors: binding versus biology. IUBMB Life 2004; 56:257-65; PMID:15370889; http://dx.doi.org/10.1080/15216540412331270076
- Day B, To C, Himanen JP, Smith FM, Nikolov DB, Boyd AW, Lackmann M. Three distinct molecular surfaces in ephrin-A5 are essential for a functional interaction with EphA3. J Biol Chem 2005; 280:26526-32; PMID:15901737; http://dx.doi.org/10.1074/jbc.M504972200
- Pabbisetty KB, Yue X, Li C, Himanen JP, Zhou R, Nikolov DB, Hu L. Kinetic analysis of the binding of monomeric and dimeric ephrins to Eph receptors: correlation to function in a growth cone collapse assay. CA>Protein Sci 2007; 16:355-61; http://dx.doi.org/10.1110/ps.062608807
- Noberini R, Rubio de la Torre E, Pasquale EB. Profiling Eph receptor expression in cells and tissues: a targeted mass spectrometry approach. Cell Adh Migr 2012; 6:102-12; PMID:22568954; http://dx.doi.org/10.4161/cam.19620
- Flanagan JG, Vanderhaeghen P. The ephrins and Eph receptors in neural development. Annu Rev Neurosci 2003; 21:309-45; http://dx.doi.org/10.1146/annurev.neuro.21.1.309
- Surawska H, Ma PC, Salgia R. The role of ephrins and Eph receptors in cancer. Cytokine Growth Factor Rev 2004; 15:419-33; PMID:15561600; http://dx.doi.org/10.1016/j.cytogfr.2004.09.002
- Astin JW, Batson J, Kadir S, Charlet J, Persad RA, Gillatt D, Oxley JD, Nobes CD. Competition amongst Eph receptors regulates contact inhibition of locomotion and invasiveness in prostate cancer cells. Nat Cell Biol 2010; 12:1194-204; PMID:21076414; http://dx.doi.org/10.1038/ncb2122
- Schaupp A, Sabet O, Dudanova I, Ponserre M, Bastiaens P, Klein R. The composition of EphB2 clusters determines the strength in the cellular repulsion response. J Cell Biol 2014; 204:409-22; PMID:24469634; http://dx.doi.org/10.1083/jcb.201305037
- Zimmer M, Palmer A, Kohler J, Klein R. EphB-ephrinB bi-directional endocytosis terminates adhesion allowing contact mediated repulsion. Nat Cell Biol 2003; 5:869-78; PMID:12973358; http://dx.doi.org/10.1038/ncb1045
- Marston DJ, Dickinson S, Nobes CD. Rac-dependent trans-endocytosis of ephrinBs regulates Eph-ephrin contact repulsion. Nat Cell Biol 2003; 5:879-88; PMID:12973357; http://dx.doi.org/10.1038/ncb1044
- Janes PW, Saha N, Barton WA, Kolev MV, Wimmer-Kleikamp SH, Nievergall E, Blobel CP, Himanen JP, Lackmann M, Nikolov DB. Adam meets Eph: an ADAM substrate recognition module acts as a molecular switch for ephrin cleavage in trans. Cell 2005; 123:291-304; PMID:16239146; http://dx.doi.org/10.1016/j.cell.2005.08.014
- Wimmer-Kleikamp SH, Nievergall E, Gegenbauer K, Adikari S, Mansour M, Yeadon T, Boyd AW, Patani NR, Lackmann M. Elevated protein tyrosine phosphatase activity provokes Eph/ephrin-facilitated adhesion of pre-B leukemia cells. Blood 2008; 112:721-32; PMID:18385452; http://dx.doi.org/10.1182/blood-2007-11-121681
- Halloran MC, Wolman MA. Repulsion or adhesion: receptors make the call. Curr Opin Cell Biol 2006; 18:533-40; PMID:16930978; http://dx.doi.org/10.1016/j.ceb.2006.08.010
- Arvanitis D, Davy A. Eph/ephrin signaling: networks. Genes Dev 2008; 22:416-29; PMID:18281458; http://dx.doi.org/10.1101/gad.1630408
- Dravis C, Yokoyama N, Chumley MJ, Cowan CA, Silvany RE, Shay J, Baker LA, Henkemeyer M. Bidirectional signaling mediated by ephrin-B2 and EphB2 controls urorectal development. Dev Biol 2004; 271:272-90; PMID:15223334; http://dx.doi.org/10.1016/j.ydbio.2004.03.027
- Senturk A, Pfennig S, Weiss A, Burk K, Acker-Palmer A. Ephrin Bs are essential components of the Reelin pathway to regulate neuronal migration. Nature 2011; 472:356-60; PMID:21460838; http://dx.doi.org/10.1038/nature09874
- Dottori M, Hartley L, Galea M, Paxinos G, Polizzotto M, Kilpatrick T, Bartlett PF, Murphy M, Kontgen F, Boyd AW. EphA4 (Sek1) receptor tyrosine kinase is required for the development of the corticospinal tract. Proc Natl Acad Sci U S A 1998; 95:13248-53; PMID:9789074; http://dx.doi.org/10.1073/pnas.95.22.13248
- Medina A, Swain RK, Kuerner KM, Steinbeisser H. Xenopus paraxial protocadherin has signaling functions and is involved in tissue separation. EMBO J 2004; 23:3249-58; PMID:15272309; http://dx.doi.org/10.1038/sj.emboj.7600329
- Kim SH, Yamamoto A, Bouwmeester T, Agius E, Robertis EM. The role of paraxial protocadherin in selective adhesion and cell movements of the mesoderm during Xenopus gastrulation. Development 1998; 125:4681-90; PMID:9806917
- Yamamoto A, Amacher SL, Kim SH, Geissert D, Kimmel CB, De Robertis EM. Zebrafish paraxial protocadherin is a downstream target of spadetail involved in morphogenesis of gastrula mesoderm. Development 1998; 125:3389-97; PMID:9693142
- Yamamoto A, Kemp C, Bachiller D, Geissert D, De Robertis EM. Mouse paraxial protocadherin is expressed in trunk mesoderm and is not essential for mouse development. Genesis 2000; 27:49-57.
- Kim SH, Jen WC, De Robertis EM, Kintner C. The protocadherin PAPC establishes segmental boundaries during somitogenesis in Xenopus embryos. Curr Biol 2000.
- Sawada A, Fritz A, Jiang YJ, Yamamoto A, Yamasu K, Kuroiwa A, Saga Y, Takeda H. Zebrafish Mesp family genes, mesp-a and mesp-b are segmentally expressed in the presomitic mesoderm, and Mesp-b confers the anterior identity to the developing somites. Development 2000; 127:1691-702; PMID:10725245
- Rhee J, Takahashi Y, Saga Y, Wilson-Rawls J, Rawls A. The protocadherin papc is involved in the organization of the epithelium along the segmental border during mouse somitogenesis. Dev Biol 2003; 254:248-61; PMID:12591245; http://dx.doi.org/10.1016/S0012-1606(02)00085-4
- Chung HA, Yamamoto TS, Ueno N. ANR5, an FGF Target Gene Product, Regulates Gastrulation in Xenopus. Curr Biol 2007; 17:932-9; PMID:17475493; http://dx.doi.org/10.1016/j.cub.2007.04.034
- Winklbauer R, Medina A, Swain RK, Steinbeisser H. Frizzled-7 signalling controls tissue separation during Xenopus gastrulation. Nature 2001; 413:856-60; PMID:11677610; http://dx.doi.org/10.1038/35101621
- Hukriede NA, Tsang TE, Habas R, Khoo PL, Steiner K, Weeks DL, Tam PP, Dawid IB. Conserved requirement of Lim1 function for cell movements during gastrulation. Dev Cell 2003; 4:83-94; PMID:12530965; http://dx.doi.org/10.1016/S1534-5807(02)00398-2
- Maghzal N, Vogt E, Reintsch W, Fraser JS, Fagotto F. The tumor associated EpCAM regulates morphogenetic movements through intracellular signaling. J Cell Biol 2010; 119:645-59; http://dx.doi.org/10.1083/jcb.201004074
- Chen X, Gumbiner BM. Paraxial protocadherin mediates cell sorting and tissue morphogenesis by regulating C-cadherin adhesion activity. J Cell Biol 2006; 174:301-13; PMID:16847104; http://dx.doi.org/10.1083/jcb.200602062
- Chen X, Koh E, Yoder M, Gumbiner BM. A protocadherin-cadherin-FLRT3 complex controls cell adhesion and morphogenesis. PLoS ONE 2009; 4:e8411; PMID:20027292; http://dx.doi.org/10.1371/journal.pone.0008411
- Kraft B, Berger CD, Wallkamm V, Steinbeisser H, Wedlich D. Wnt-11 and Fz7 reduce cell adhesion in convergent extension by sequestration of PAPC and C-cadherin. J Cell Biol 2012; 198:695-709; PMID:22908314; http://dx.doi.org/10.1083/jcb.201110076
- Unterseher F, Hefele JA, Giehl K, De Robertis EM, Wedlich D, Schambony A. Paraxial protocadherin coordinates cell polarity during convergent extension via Rho A and JNK. EMBO J 2004; 23:3259-69; PMID:15297873; http://dx.doi.org/10.1038/sj.emboj.7600332
- Wang Y, Janicki P, Koster I, Berger CD, Wenzl C, Grosshans J, Steinbeisser H. Xenopus Paraxial Protocadherin regulates morphogenesis by antagonizing Sprouty. Genes Dev 2008; 22:878-83; PMID:18381892; http://dx.doi.org/10.1101/gad.452908
- Kietzmann A, Wang Y, Weber D, Steinbeisser H. Xenopus paraxial protocadherin inhibits Wnt/β-catenin signalling via casein kinase 2beta. EMBO Rep. 2012; 13:129-34; PMID:22193776; http://dx.doi.org/10.1038/embor.2011.240
- Kao TJ, Kania A. Ephrin-mediated cis-attenuation of Eph receptor signaling is essential for spinal motor axon guidance. Neuron 2011; 71:76-91; PMID:21745639; http://dx.doi.org/10.1016/j.neuron.011.05.031
- Xu Q, Wilkinson DG. Eph-related receptors and their ligands: mediators of contact dependent cell interactions. J Mol Med 1997; 75:576-86; PMID:9297625; http://dx.doi.org/10.1007/s001090050142
- Glazier JA, Zhang Y, Swat M, Zaitlen B, Schnell S. Coordinated action of N-CAM, N-cadherin, EphA4, and ephrinB2 translates genetic prepatterns into structure during somitogenesis in chick. Curr Top Dev Biol 2008; 81:205-47; PMID:18023729; http://dx.doi.org/10.1016/S0070-2153(07)81007-6
- Fagotto F The cellular basis of tissue separation. Development; 2014; 141:3303–3318. http://dx.doi.org/10.1242/dev.090332
- Luu O, David R, Ninomiya H, Winklbauer R. Large-scale mechanical properties of Xenopus embryonic epithelium. Proc Natl Acad Sci U S A 2011; 108:4000-5; PMID:21368110; http://dx.doi.org/10.1073/pnas.1010331108
- Aliee M, Roper JC, Landsberg KP, Pentzold C, Widmann TJ, Julicher F, Dahmann C. Physical mechanisms shaping the Drosophila dorsoventral compartment boundary. Curr Biol 2012; 22:967-76; PMID:22560616; http://dx.doi.org/10.1016/j.cub.2012.03.070
- Julich D, Mould AP, Koper E, Holley SA. Control of extracellular matrix assembly along tissue boundaries via Integrin and Eph/Ephrin signaling. Development 2009; 136:2913-21; PMID:19641014; http://dx.doi.org/10.1242/dev.038935
- Richter DJ, King N. The genomic and cellular foundations of animal origins. Annu Rev Genet 2013; 47:509-37; PMID:24050174; http://dx.doi.org/10.1146/annurev-genet-111212-133456
- Mellott DO, Burke RD. The molecular phylogeny of eph receptors and ephrin ligands. BMC Cell Biol 2008; 9:27; PMID:18495034; http://dx.doi.org/10.1186/1471-2121-9-27
- Reddy PC, Bidaye SS, Ghaskadbi S. Genome-wide screening reveals the emergence and divergence of RTK homologues in basal Metazoan Hydra magnipapillata. J Biosci 2011; 36:289-96; PMID:21654083; http://dx.doi.org/10.1007/s12038-011-9065-6
- Chang LH, Chen P, Lien MT, Ho YH, Lin CM, Pan YT, Wei SY, Hsu JC. Differential adhesion and actomyosin cable collaborate to drive Echinoid-mediated cell sorting. Development 2011; 138:3803-12; PMID:21795280; http://dx.doi.org/10.1242/dev.062257
- Laplante C, Nilson LA. Asymmetric distribution of Echinoid defines the epidermal leading edge during Drosophila dorsal closure. J Cell Biol 2011; 192:335-48; PMID:21263031; http://dx.doi.org/10.1083/jcb.201009022
- Lin HP, Chen HM, Wei SY, Chen LY, Chang LH, Sun YJ, Huang SY, Hsu JC. Cell adhesion molecule Echinoid associates with unconventional myosin VI/Jaguar motor to regulate cell morphology during dorsal closure in Drosophila. Dev Biol 2007; 311:423-33; PMID:17936269; http://dx.doi.org/10.1016/j.ydbio.2007.08.043
- Theil T, Frain M, Gilardi-Hebenstreit P, Flenniken A, Charnay P, Wilkinson DG. Segmental expression of the EphA4 (Sek-1) receptor tyrosine kinase in the hindbrain is under direct transcriptional control of Krox-20. Development 1998; 125:443-52; PMID:9425139
- Kemler R. From cadherins to catenins: cytoplasmic protein interactions and regulation of cell adhesion. Trends Genet 1993; 9:317-21; PMID:8236461; http://dx.doi.org/10.1016/0168-9525(93)90250-L
- Himanen JP, Rajashankar KR, Lackmann M, Cowan CA, Henkemeyer M, Nikolov DB. Crystal structure of an Eph receptor-ephrin complex. Nature 2001; 414:933-8; PMID:11780069; http://dx.doi.org/10.1038/414933a
- Nikolov DB, Li C, Barton WA, Himanen JP. Crystal structure of the ephrin-B1 ectodomain: implications for receptor recognition and signaling. Biochemistry 2005; 44:10947-53; PMID:16101278; http://dx.doi.org/10.1021/bi050789w
- Stein E, Huynh-Do U, Lane AA, Cerretti DP, Daniel TO. Nck recruitment to Eph receptor, EphB1/ELK, couples ligand activation to c-Jun kinase. J Biol Chem 1998; 273:1303-8; PMID:9430661; http://dx.doi.org/10.1074/jbc.273.3.1303
- Himanen JP, Nikolov DB. Eph signaling: a structural view. Trends Neurosci 2003; 26:46-51; PMID:12495863; http://dx.doi.org/10.1016/S0166-2236(02)00005-X
- Coulthard MG, Lickliter JD, Subanesan N, Chen K, Webb GC, Lowry AJ, Koblar S, Bottema CD, Boyd AW. Characterization of the Epha1 receptor tyrosine kinase: expression in epithelial tissues. Growth Fact 2001; 18:303-17; PMID:11519828; http://dx.doi.org/10.3109/08977190109029118
- Gale NW, Holland SJ, Valenzuela DM, Flenniken A, Pan L, Ryan TE, Henkemeyer M, Strebhardt K, Hirai H, Wilkinson DG, et al. Eph receptors and ligands comprise two major specificity subclasses and are reciprocally compartmentalized during embryogenesis. Neuron 1996; 17:9-19; PMID:8755474; http://dx.doi.org/10.1016/S0896-6273(00)80276-7
- Janis LS, Cassidy RM, Kromer LF. Ephrin-A binding and EphA receptor expression delineate the matrix compartment of the striatum. J Neurosci 1999; 19:4962-71; PMID:10366629
- Smith FM, Vearing C, Lackmann M, Treutlein H, Himanen J, Chen K, Saul A, Nikolov D, Boyd AW. Dissecting the EphA3/Ephrin-A5 interactions using a novel functional mutagenesis screen. J Biol Chem 2004; 279:9522-31; PMID:14660665; http://dx.doi.org/10.1074/jbc.M309326200
- Irie N, Takada Y, Watanabe Y, Matsuzaki Y, Naruse C, Asano M, Iwakura Y, Suda T, Matsuo K. Bidirectional signaling through ephrinA2-EphA2 enhances osteoclastogenesis and suppresses osteoblastogenesis. J Biol Chem 2009; 284:14637-44; PMID:19299512; http://dx.doi.org/10.1074/jbc.M807598200
- Nakamoto M. Eph receptors and ephrins. Int J Biochem Cell Biol 2000; 32:7-12; PMID:10661890; http://dx.doi.org/10.1016/S1357-2725(99)00096-5
- Himanen JP, Goldgur Y, Miao H, Myshkin E, Guo H, Buck M, Nguyen M, Rajashankar KR, Wang B, Nikolov DB. Ligand recognition by A-class Eph receptors: crystal structures of the EphA2 ligand-binding domain and the EphA2/ephrin-A1 complex. EMBO Rep 2009; 10:722-8; PMID:19525919; http://dx.doi.org/10.1038/embor.2009.91
- Nieto MA, Gilardi-Hebenstreit P, Charnay P, Wilkinson DG. A receptor protein tyrosine kinase implicated in the segmental patterning of the hindbrain and mesoderm. Development 1992; 116:1137-50; PMID:1295734
- Becker N, Seitanidou T, Murphy P, Mattei MG, Topilko P, Nieto MA, Wilkinson DG, Charnay P, Gilardi-Hebenstreit P. Several receptor tyrosine kinase genes of the Eph family are segmentally expressed in the developing hindbrain. Mech Dev 1994; 47:3-17; PMID:7947319; http://dx.doi.org/10.1016/0925-4773(94)90091-4
- Bergemann AD, Cheng HJ, Brambilla R, Klein R, Flanagan JG. ELF-2, a new member of the Eph ligand family, is segmentally expressed in mouse embryos in the region of the hindbrain and newly forming somites. Mol Cell Biol 1995; 15:4921-9; PMID:7651410
- Helbling PM, Saulnier DM, Robinson V, Christiansen JH, Wilkinson DG, Brandli AW. Comparative analysis of embryonic gene expression defines potential interaction sites for Xenopus EphB4 receptors with ephrin-B ligands. Dev Dyn 1999; 216:361-73; PMID:10633856; http://dx.doi.org/10.1002/(SICI)1097-0177(199912)216:4/5%3c361::AID-DVDY5%3e3.0.CO;2-W
- Smith A, Robinson V, Patel K, Wilkinson DG. The EphA4 and EphB1 receptor tyrosine kinases and ephrin-B2 ligand regulate targeted migration of branchial neural crest cells. Curr Biol 1997; 7:561-70; PMID:9259557; http://dx.doi.org/10.1016/S0960-9822(06)00255-7
- Manzanares M, Trainor PA, Nonchev S, Ariza-McNaughton L, Brodie J, Gould A, Marshall H, Morrison A, Kwan CT, Sham MH, et al. The role of kreisler in segmentation during hindbrain development. Dev Biol 1999; 211:220-37; PMID:10395784; http://dx.doi.org/10.1006/dbio.1999.9318
- Helbling PM, Tran CT, Brandli AW. Requirement for EphA receptor signaling in the segregation of Xenopus third and fourth arch neural crest cells. Mech Dev 1998; 78:63-79; PMID:9858686; http://dx.doi.org/10.1016/S0925-4773(98)00148-8